Regenerative technology for future therapy of erectile dysfunction
Introduction
Although oral phosphodiesterase-5 (PDE5) inhibitors are generally an effective and well-tolerated therapy for erectile dysfunction (ED), such therapies are still far from curing ED and do not reverse the vasculopathic or neuropathic processes associated with ED. Moreover, certain populations with organic ED, such as that resulting from diabetes or radical prostatectomy (RP), respond poorly to PDE5 inhibitors. This reduced responsiveness to PDE5 inhibitors may be related to the severity of endothelial dysfunction or nerve damage. Because the effects of PDE5 inhibitors rely on endogenous nitric oxide (NO) formation, if the bioavailable NO is insufficient as the result of severe endothelial dysfunction or nerve damage, PDE5 inhibitors may fail to increase the level of cGMP above the necessary threshold. Furthermore, PDE5 inhibitors have limited spontaneity. In addition, the use of PDE5 inhibitors is absolutely contraindicated in men who take nitrate compounds because of the risk of extreme hypotension or even death (1). Therefore, new therapeutics need to be developed to solve these problems.
Regenerative medicine is the repair or replacement of damaged tissues and organs through a range of therapeutic strategies. Restoring NO bioavailability by rejuvenating or regenerating cavernous endothelial cells, cavernous nerve, or both, may serve as a promising therapeutic strategy for curing ED, especially in men with severe ED that is unresponsive to PDE5 inhibitors. This article addresses the current treatment targets in the preclinical stage, including pharmacotherapy, growth factor therapy, gene therapy, and cell or stem cell therapy to restore erectile function by regenerating corpus cavernosum tissue or cavernous nerve. We focus on the potential for the use of regenerative technology to induce therapeutic angiogenesis or neuroregeneration and to inhibit tissue fibrosis. We do not address tissue engineering in this section, because the technique may have a role in only a limited group of patients who have major loss of penile tissue from injury or malignancy. This work also addresses future perspectives in ED therapy from our point of view.
Current status of regenerative technology
Therapeutic angiogenesis
The penis is a richly vascularized organ, and ED is predominantly a vascular disease. Recently, a link between ED and cardiovascular disease was uncovered whereby both diseases were shown to share the same risk factors, including hypercholesterolemia, hypertension, diabetes mellitus, and smoking, with endothelial cell dysfunction being the common denominator between these two conditions. These findings suggest that ED is another manifestation of systemic vascular disorder. It has been shown that vascular risk factors in men and animal models cause impairments in endothelium-dependent smooth muscle relaxation, endothelial nitric oxide synthase (eNOS) enzyme activity, and penile angiogenesis as well as a decrease in expression of angiogenic factors, resulting in ED (2). Thus, a promising therapeutic strategy in patients with ED of vascular origin may be to augment endogenous NO bioactivity and to reestablish the structural and functional microvasculature. In this regard, neovascularization is an emerging therapy for the treatment of ED.
To date, many reports have been made of targeting cavernous endothelial regeneration by use of direct protein delivery, gene transfer, stem cells, or stem cells transduced with angiogenic factor (Figure 1). Vascular endothelial growth factor-A (VEGF-A) has been the most intensively studied in relationship to the penile vasculature and erectile function. Intracavernous delivery of the VEGF-A gene or protein has been shown to restore erectile function in animal models of vasculogenic ED (2). However, treatment with exogenous VEGF-A often results in leaky, inflamed, and disorganized blood vessels in experimental systems (3), which greatly compromises the therapeutic value of VEGF-A. In comparison, angiopoietin-1 (Ang1), the ligand of the Tie2 receptor tyrosine kinase, is an angiogenic growth factor that specifically functions to generate a nonleaky, stable, and functional vasculature. Transgenic overexpression or gene transfer of Ang1 not only enhances vessel formation, but also protects the adult vasculature against vascular leakage (4). Thus, Ang1 has potential therapeutic applications in angiogenesis and the prevention of vascular leakage. In addition, when administered with VEGF, Ang1 can counteract VEGF-induced side effects while having an additive effect on vessel formation (4).
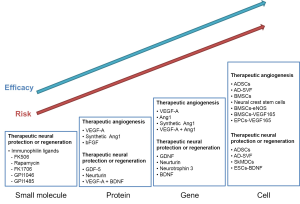
We recently reported in mouse models of type I and type II diabetic ED that two successive intracavernous injections of synthetic Ang1 protein, a soluble and potent Ang1 variant, significantly increased cavernous endothelial cell proliferation, eNOS phosphorylation, and cGMP expression and decreased the production of reactive oxygen species, such as superoxide anion and peroxynitrite (5,6). These changes restored erectile function up to 4 weeks in the diabetic mice. Local delivery of synthetic Ang1 protein also induced complete recovery of endothelial cell-cell junction proteins and decreased cavernous endothelial permeability in the diabetic mice (5). However, some debate remains over the efficacy of administration of proteins, which have a short half-life and thus may not result in a durable response. To test whether the angiogenic factor protein can induce cavernous endothelial cell proliferation, we assessed the number of endothelial cells staining positive for phosphohistone H3 (a nuclear protein indicative of cell proliferation). We noted significant increases in phosphohistone H3-positive endothelial cells 1, 3, and 6 h after injection of synthetic Ang1 protein (5). This finding suggests that the effects of synthetic Ang1 protein are established at an early time point after injection and provides a mechanism by which an angiogenic factor protein with a short half-life can induce durable effects. The long-lasting restoration of erectile function achieved after two successive intracavernous injections of synthetic Ang1 protein is intriguing: from a clinical standpoint, the use of a therapeutic protein to treat human ED could promote safer and more accurate therapeutic angiogenesis than that produced by gene therapy.
Since the late 1990s, many investigators have reported the results of gene therapy in ED with the use of a variety of therapeutic genes. We previously reported that combined adenovirus-mediated gene transfer of Ang1 and VEGF-A promoted angiogenesis cooperatively in a rat model of hypercholesterolemic ED and resulted in a complete recovery of erectile function, whereas intracavernous injection of either ad-Ang1 or ad-VEGF165 alone elicited partial improvement in erectile function (7). Despite encouraging results in preclinical studies, however, no therapeutic genes have so far been approved for the treatment of patients with ED. One possible reason for this lack of approval is problems with the vectors used for gene delivery. For most studies of gene therapy in ED, viral or naked plasmid vectors have been used. However, viral vectors have high immunogenicity and possible mutagenesis, thus limiting their application in humans. By contrast, naked plasmid DNA is safe in terms of cytotoxicity and immunogenicity, but its low transfection efficiency limits its use in a clinical setting.
The infidelity of current gene delivery methods, such as the lack of specificity, can lead to the transduction of a therapeutic gene to unwanted cells in the corpus cavernosum tissue. We believe that one solution to this problem in the case of vasculogenic ED may be the use of a hypoxia-specific gene expression system to facilitate gene delivery into ischemic corpus cavernosum tissue. For example, the expression system consisting of the RTP801 promoter and the erythropoietin (Epo) enhancer induces gene expression in a hypoxia-dependent manner (8). We recently observed that reporter gene expression was profoundly induced by Epo enhancer and RTP801 promoter in both the corpus cavernosum tissue of hypercholesterolemic mice in vivo and in mouse cavernous endothelial cells exposed to hypoxic conditions in vitro (9). In contrast, gene expression was not induced in normal corpus cavernosum tissue, which may thus reduce the uncontrolled expression of the exogenous gene in nonischemic areas. Interestingly, transgene expression was mainly distributed in the cavernous endothelial cells and not in the smooth muscle cells. Therefore, it seems particularly advantageous to apply the hypoxia-inducible gene expression system to genes targeting endothelial cell regeneration, such as VEGF-A and Ang1. The cell-specific expression of therapeutic genes will lead to more precise conclusions about the exact function of specific proteins.
Increasing attention has been given to stem cell therapy to enhance endothelial regeneration for the treatment of ED. Lue’s group from the University of California San Francisco found that cultured adipose tissue-derived stem cells increase cavernous endothelial cell content and restore erectile function in rats with ED from type II diabetes or hypercholesterolemia (10). Meanwhile, adipose tissue-derived stromal vascular fraction (SVF) has been introduced as an ideal source of multipotent stem cells that can be easily harvested in high quantities through minimally invasive procedures. Stem cells isolated from SVF can promote neovascularization in the ischemic condition in vivo by differentiating into vascular endothelial cells or by secreting angiogenic factors (11). We found that implanting SVF into the mouse ischemic hind limb induced the robust formation of a local neovascular network and salvaged the limb. Moreover, the co-implantation of SVF prevented fat absorption in a subcutaneous adipose tissue graft model (12). In the first application of SVF in the sexual medicine field, we observed in a mouse model of type I diabetes that a single intracavernous injection of freshly isolated SVF significantly increased cavernous endothelial cell proliferation, eNOS phosphorylation, and cGMP expression, which resulted in the recovery of erectile function in the diabetic mice (13). A significant increase in VEGF-A expression was noted in the cavernous tissue of diabetic mice after intracavernous injection of SVF. Moreover, SVF-induced cavernous angiogenesis and subsequent restoration of erectile function was abolished or reduced in the presence of VEGF-Trap, a soluble VEGF-A neutralizing antibody. Although some SVF integrated into the cavernous endothelium and underwent differentiation into endothelial cells, the frequency of this event was relatively low. These results suggest that the paracrine effect rather than the direct transformation of SVF into endothelial cells may play a preeminent role in the recovery of cavernous endothelial integrity and erectile function. The main advantages of using freshly isolated SVF are that this fraction can be easily harvested from ED patients in great quantities with simple and minimally invasive techniques and that the SVF can be transplanted to the patients without further in vitro selection or expansion of the cells. We believe that local SVF therapy will be a useful autologous treatment option for curing vascular disease-induced ED. The effect of SVF on diabetes-induced ED, however, was not complete. It remains to be elucidated whether modification of SVF therapy, such as preconditioning of SVF or combination with angiogenic factors, will result in better outcomes for ED patients with severe vascular diseases.
Therapeutic neuroregeneration
Similar to the premise of therapeutic angiogenesis, neuromodulatory strategies offer the potential to restore normal erectile function. Much attention has been given to strategies for restoring cavernous nerve in men undergoing RP for prostate cancer. Despite the introduction of nerve-sparing techniques and advances in operative techniques, ED resulting from cavernous nerve injury is one of the major complications following RP for prostate cancer. Even with bilateral nerve-sparing approaches, some degree of cavernous nerve injury or neurapraxia is unavoidable owing to the lack of knowledge regarding the actual running course of cavernous nerves, which supposedly run close to the prostate. Advances in the neurobiology field have led us to develop neuromodulatory strategies targeting cavernous nerve protection or regeneration. Several treatment modalities are currently under investigation in animal models, including immunophilin ligands, neurotrophins, growth factors, stem cells, and stem cells transduced with neurotrophic factor (Figure 1). Although neuromodulatory strategies have been shown to induce recovery of erectile function at the preclinical level (14), a recent clinical trial to evaluate the efficacy of GPI 1485, a nonimmunosuppressive analogue of FK506, on erectile function in men undergoing nerve-sparing RP did not report the recovery of erectile function (15). This finding suggests that treatment of neurapraxia or partial nerve injury may be not enough to restore erectile function because pathophysiologic changes have already begun in the erectile tissues, such as apoptosis of endothelial and smooth muscle cells and fibrosis in the corpus cavernosum tissue. Along with identification of the novel molecule that promotes neuroprotection or neuroregeneration, further studies are needed to test whether combinations of neuromodulatory strategies can achieve positive clinical outcomes.
Recently, Lue’s group evaluated the effect of autologous adipose-derived SVF on erectile function in a rat model of cavernous injury. They found that both immediate and delayed treatment with SVF resulted in a significant recovery of erectile function. The intracavernous injection of SVF significantly increased the expression of neuronal NOS and neurofilament in dorsal penile nerves compared with that in the vehicle-treated group. Furthermore, the smooth muscle-to-collagen ratio within the corpus cavernosum tissue was significantly improved in the SVF groups. Thus, autologous SVF treatment is expected to be clinically applicable to prevent or treat ED in association with RP (16).
Anti-fibrosis
Cavernous fibrosis characterized by the accumulation of extracellular matrix and a decrease in smooth muscle content is another pathologic consequence of ED resulting from vascular risk factors or from nerve injury. Transforming growth factor-β (TGF-β) has been suggested as the most relevant fibrogenic cytokine. We and other investigators have reported in animals and humans that cavernous nerve injury or vascular risk factors increase the expression of TGF-β1 and induce the phosphorylation of the Smad2/3 transcriptional factor (17,18), the crucial step in the initiation of TGF-β-mediated tissue fibrosis, in the corpus cavernosum tissue. Phosphorylation of Smad2/3 in turn promotes the deposition of extracellular matrix proteins and tissue fibrosis. Moreover, TGF-β and the Smad2/3 signal pathway are known to mediate smooth muscle apoptosis and to inhibit endothelial cell proliferation (17). We recently observed in mice that apoptosis in cavernous smooth muscle and endothelial cells and up-regulation of the TGF-β1-Smad2 pathway in the corpus cavernosum tissue begin as early as 3 days after cavernous nerve injury (17). This finding led us to investigate the effectiveness of an intervention that inhibits the TGF-β signal pathway in an animal model of cavernous nerve injury. Smad7 is an inhibitory Smad protein that blocks TGF-β signaling through a negative feedback loop. Smad7 protein binds the TGF-β type I receptor, which blocks the phosphorylation of receptor-associated Smads, including Smad2 and Smad3, and eventually inhibits downstream signaling of TGF-β. A recent study reported that Smad7 gene transfer attenuates renal fibrosis in diabetic rats, whereas targeted deletion of the Smad7 gene enhances renal fibrosis and inflammation (19). We observed that a single intracavernous injection of adenovirus encoding the Smad7 gene into cavernous nerve injury mice significantly restored erectile function by inducing cavernous endothelial cell proliferation and eNOS phosphorylation and by decreasing endothelial cell apoptosis and the production of extracellular matrix proteins (Ryu JK and Suh JK, unpublished data). These findings suggest that an anti-fibrotic strategy through inhibition of the TGF-β signaling pathway may represent a promising therapeutic strategy for RP-induced ED.
Although we can anticipate greater therapeutic efficacy and more durable responses with gene- or stem cell-based therapy than with pharmacotherapy or protein therapy, there are potential risks or drawbacks to gene- or stem cell-based therapies (Figure 1). These include the overexpression of the transduced gene in an undesired area, unintended differentiation of the injected cells, and lack of long-term safety data in humans. Optimization of the safety, specificity, and efficacy of therapeutic genes or stem cells, as well as determining the proper dosage and ideal delivery methods, is necessary to overcome the current restrictions in the clinical development of gene or stem cell therapy.
Future perspectives
Whereas numerous studies have so far reported promising results with the use of regenerative technologies in a variety of animal models, we are not yet at the point of beginning human trials. Because ED in humans is multifactorial in etiology and because no single factor is solely responsible for the pathogenesis of ED, it is necessary to determine whether the combination of angiogenesis, neuroprotection or neuroregeneration, and anti-fibrosis strategies can induce synergic effects on the recovery of erectile function (Figure 2). Furthermore, targeted therapy tailored to individual patients that is based on molecular pathophysiology may also accelerate the clinical application of regenerative technology in the field of ED (Figure 3).
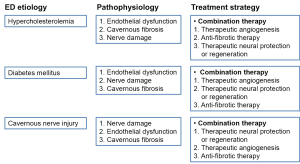
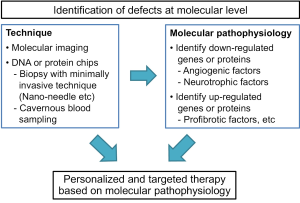
Combination therapy
It is obvious that all of the therapeutic strategies addressed so far can be applied not only individually but also in combination. Because blood vessel formation requires the involvement of a variety of growth factors or cytokines with cascades of intracellular signal transductions, such therapies may require the application of more than a single growth factor. This concept is supported by our previous study showing that combined application of the angiogenic factors Ang1 and VEGF-A synergistically induces the recovery of cavernous angiogenesis and erectile function in hypercholesterolemic rats compared with that in the groups treated with either therapy alone (7). Similarly, combination therapy with an angiogenic factor and a neurotrophic factor, VEGF-A and brain-derived neurotrophic factor (BDNF), was also shown to induce additive effects on erectile function in animal models of cavernous nerve injury and hypercholesterolemia (20,21).
Although the causes of ED, including hypercholesterolemia, diabetes, and cavernous nerve injury, are various, the pathological manifestations involved in the deterioration of erectile function are somewhat similar. Endothelial dysfunction, cavernous nerve damage, and cavernous fibrosis are the common pathologic consequences of ED. The prolonged absence of erection secondary to cavernous nerve neurapraxia is known to induce apoptosis of cavernous endothelial and smooth muscle cells and to result in cavernous fibrosis. If apoptosis and fibrosis in the corpus cavernosum tissue following RP could be prevented with angiogenic or anti-fibrotic factor therapy while the cavernous nerve regenerates, it is expected that irreversible or permanent damage to the erectile tissue could be avoided and the recovery of erectile function would be enhanced. In addition, vascular risk factors including diabetes and dyslipidemia, the major causal factors of cavernous endothelial dysfunction and fibrosis, may also induce neuropathic changes in the penis. This finding is supported by results from a previous study showing that adenovirus-mediated gene transfer of BDNF resulted in the recovery of erectile function by reducing neuronal damage in a rat model of hypercholesterolemia induced by a high-fat diet (21). In another study, herpes simplex virus vector encoding the neurotrophin-3 gene also increased nNOS expression in pelvic ganglion and restored erectile function in diabetic rats (22).
The results of these studies provide a new direction for the therapeutic strategy in ED. The combination of therapeutic angiogenesis, therapeutic neuroprotection or neuroregeneration, and anti-fibrosis strategies can be broadly useful or be specifically advantageous for patients with severe ED (Figure 2).
Targeted therapy based on molecular pathophysiology
We propose the concept that pathophysiologic consequences, especially at the molecular level, may differ among individual ED patients, even though the patients have similar risk factors for ED. In the near future, with advances in technology in the field of molecular imaging and gene or protein analysis techniques, we will be able to identify which angiogenic or neurotrophic factors are down-regulated and which profibrotic factors are up-regulated by use of a small amount of cavernous blood or cavernous tissue obtained by a minimally invasive technique or by use of noninvasive molecular imaging. According to these results, we can deliver personalized and targeted therapy to patients with ED (Figure 3).
With the success of combination strategies and targeted therapy tailored to individual patients, we believe that regenerative technology may become a realistic therapeutic tool in the field of ED.
Acknowledgements
Funding: This study was supported by a grant of the Korea Healthcare technology R&D Project, Ministry for Health, Welfare & Family Affairs, Republic of Korea (Jun-Kyu Suh, A110076).
We thank Jennifer Holmes for help in preparing the manuscript.
Footnote
Conflicts of Interest: The authors have no conflicts of interest to declare.
References
- Dorsey P, Keel C, Klavens M, et al. Phosphodiesterase type 5 (PDE5) inhibitors for the treatment of erectile dysfunction. Expert Opin Pharmacother 2010;11:1109-22. [PubMed]
- Lysiak JJ, Kavoussi PK, Ellati RT, et al. Angiogenesis therapy for the treatment of erectile dysfunction. J Sex Med 2010;7:2554-63. [PubMed]
- Lee RJ, Springer ML, Blanco-Bose WE, et al. VEGF gene delivery to myocardium: deleterious effects of unregulated expression. Circulation 2000;102:898-901. [PubMed]
- Augustin HG, Koh GY, Thurston G, et al. Control of vascular morphogenesis and homeostasis through the angiopoietin-Tie system. Nat Rev Mol Cell Biol 2009;10:165-77. [PubMed]
- Jin HR, Kim WJ, Song JS, et al. Intracavernous delivery of a designed angiopoietin-1 variant rescues erectile function by enhancing endothelial regeneration in the streptozotocin-induced diabetic mouse. Diabetes 2011;60:969-80. [PubMed]
- Jin HR, Kim WJ, Song JS, et al. Intracavernous delivery of synthetic angiopoietin-1 protein as a novel therapeutic strategy for erectile dysfunction in the type II diabetic db/db mouse. J Sex Med 2010;7:3635-46. [PubMed]
- Ryu JK, Cho CH, Shin HY, et al. Combined angiopoietin-1 and vascular endothelial growth factor gene transfer restores cavernous angiogenesis and erectile function in a rat model of hypercholesterolemia. Mol Ther 2006;13:705-15. [PubMed]
- Kim HA, Mahato RI, Lee M. Hypoxia-specific gene expression for ischemic disease gene therapy. Adv Drug Deliv Rev 2009;61:614-22. [PubMed]
- Lee M, Ryu JK, Piao S, et al. Efficient gene expression system using the RTP801 promoter in the corpus cavernosum of high-cholesterol diet-induced erectile dysfunction rats for gene therapy. J Sex Med 2008;5:1355-64. [PubMed]
- Lin CS, Xin ZC, Wang Z, et al. Stem cell therapy for erectile dysfunction: a critical review. Stem Cells Dev 2012;21:343-51. [PubMed]
- Gimble JM, Bunnell BA, Chiu ES, et al. Concise review: Adipose-derived stromal vascular fraction cells and stem cells: let’s not get lost in translation. Stem Cells 2011;29:749-54. [PubMed]
- Koh YJ, Koh BI, Kim H, et al. Stromal vascular fraction from adipose tissue forms profound vascular network through the dynamic reassembly of blood endothelial cells. Arterioscler Thromb Vasc Biol 2011;31:1141-50. [PubMed]
- Ryu JK, Tumurbaatar M, Jin HR, et al. Intracavernous delivery of freshly isolated adipose tissue-derived stromal vascular fraction rescues erectile function by enhancing endothelial regeneration in the streptozotocin-induced diabetic mouse. Eur Urol Suppl 2011;10:328-9.
- Bella AJ, Lin G, Cagiannos I, et al. Emerging neuromodulatory molecules for the treatment of neurogenic erectile dysfunction caused by cavernous nerve injury. Asian J Androl 2008;10:54-9. [PubMed]
- Burnett AL, McCullough AR, Smith JA Jr, et al. Neuromodulation to preserve erectile function after radical prostatectomy: results from the GPI 1485 neuroimmunophilin ligand clinical trial. J Urol 2007;177:383-4. (Abstract 1162).
- Qiu X, Fandel TM, Ferretti L, et al. Both Immediate and Delayed Intracavernous Injection of Autologous Adipose-derived Stromal Vascular Fraction Enhances Recovery of Erectile Function in a Rat Model of Cavernous Nerve Injury. Eur Urol 2012;62:720-7. [PubMed]
- Jin HR, Chung YG, Kim WJ, et al. A mouse model of cavernous nerve injury-induced erectile dysfunction: functional and morphological characterization of the corpus cavernosum. J Sex Med 2010;7:3351-64. [PubMed]
- Hu WL, Hu LQ, Li SW, et al. Expression of transforming growth factor-beta1 in penile tissue from rats with bilateral cavernosal nerve ablation. BJU Int 2004;94:424-8. [PubMed]
- Chen HY, Huang XR, Wang W, et al. The protective role of Smad7 in diabetic kidney disease: mechanism and therapeutic potential. Diabetes 2011;60:590-601. [PubMed]
- Chen KC, Minor TX, Rahman NU, et al. The additive erectile recovery effect of brain-derived neurotrophic factor combined with vascular endothelial growth factor in a rat model of neurogenic impotence. BJU Int 2005;95:1077-80. [PubMed]
- Gholami SS, Rogers R, Chang J, et al. The effect of vascular endothelial growth factor and adeno-associated virus mediated brain derived neurotrophic factor on neurogenic and vasculogenic erectile dysfunction induced by hyperlipidemia. J Urol 2003;169:1577-81. [PubMed]
- Bennett NE, Kim JH, Wolfe DP, et al. Improvement in erectile dysfunction after neurotrophic factor gene therapy in diabetic rats. J Urol 2005;173:1820-4. [PubMed]