Astragaloside IV protects against ischemia/reperfusion (I/R)-induced kidney injury based on the Keap1-Nrf2/ARE signaling pathway
Introduction
Acute kidney injury (AKI) commonly appears in clinical treatment, and has high morbidity and mortality (1). Studies have shown that severe AKI accounts for more than half of in-hospital deaths, and approximately 300,000 people die from AKI every year in the United States (2,3). The main feature of AKI is the rapid loss of renal excretion, which results in increased serum creatinine (CRE), urea, and decreased urine output, which is usually considered the diagnostic standard for AKI (4,5). Recently, epidemiological studies have shown that patients suffering from AKI are at high risk of developing chronic kidney disease (CKD) (2). CKD, which is characterized by chronic kidney structure and dysfunction, greatly increases the risk of several diseases, including cardiovascular disease, and thus has attracted public health attention worldwide (6).
Many studies have explored the possible role of Chinese medicine in the treatment of kidney disease and its complications (7). It has been reported that Bupleurum polysaccharides inhibit kidney inflammation and renal fibrosis (8), and alkaloids extracted from Corydalis yanhusuo to exert a protective effect in AKI by inhibiting apoptosis and inflammation (9). These studies suggest that traditional Chinese medicine could provide therapeutic drugs for the treatment of kidney disease. Astragaloside IV (AS-IV) purified from astragalus, which is a traditional Chinese medicine, has attracted wide interest in recent years, AS-IV has various pharmacological activities, including protective effects on cerebral injury and central nervous system (CNS), cardiovascular disease, respiratory system, kidney, endocrine system, organic immune system, liver and cancer due to its wide range of pharmacological actions,
Such as antioxidant, cardioprotective effects, anti-inflammatory, antiviral, anti-bacterial, anti-fibrosis, anti-diabetes and immunoregulation effects (10). Studies have shown that AS-IV attenuates the pathological process of DKA (diabetic ketoacidosis) mice by regulating the JNK/Nrf2 signaling pathway (11). Ding et al. reported that AS-IV alleviates rat pulpitis by exerting anti-inflammation and promoting differentiation effects (12). AS-IV has also been shown to have an anti-tumor effect in hepatocellular carcinoma (13) and to improve diabetic nephropathy (14).
Nrf2 plays a vital role in both the Keap1-Nrf2 signaling pathway and oxidative stress, which are closely related to kidney diseases (15,16). Recently, oxidative stress has been considered an important regulatory factor in AKI and CKD (17,18). The regulation of the Nrf2/HO-1 pathway has been shown to have a protective effect in an AKI rat model (19). Based on the above evidence, we sought to investigate whether AS-IV exerts any protective effects and explore its potential mechanism in an I/R-induced AKI rat model. We present the following article in accordance with the ARRIVE reporting checklist (available at https://tau.amegroups.com/article/view/10.21037/tau-22-505/rc).
Methods
Animal groups
A total of 36 male Sprague-Dawley rats (aged: 8 weeks; weighing: 200–220 g), purchased from Laboratory Animal Center of Southern Medical University, were randomly divided into the following 6 groups (n=6 per group): (I) the control group; (II) the sham operation group; (III) the kidney I/R injury group (the I/R group); (IV) the kidney I/R injury group treated with 2 mg/kg of AS-IV (the low-dose group); (V) the kidney I/R injury group treated with 5 mg/kg of AS-IV (the mid-dose group); and (VI) the kidney I/R injury group treated with 10 mg/kg of AS-IV (the high-dose group). All the animals were housed in a temperature (72±3 °F) and air- (50%±20% relative humidity) controlled room at an SPF animal facility, with a 12-h light/dark cycle, and were fed a standard diet and tap water. Animal experiments were performed under a project license (No. 2019001) granted by the ethics committee of Southern Medical University (People’s Hospital of Huadu District), in compliance with national guidelines for the care and use of animals. A protocol was prepared before the study without registration.
Establishment of I/R model in rats
AS-IV was intragastrically administered to the rats at doses of 10, 5, or 2 mg/kg 1 hour before the establishment of the I/R model, while equivalent amounts of physiological saline were administered to those in the I/R group. The I/R rat model was established as described in a previous study (20). In brief, the rats were anesthetized, and unilateral renal ischemia was then conducted by applying microvascular forceps to the left renal pedicle for 45 min, followed by right renal nephrectomy. The sham operation group received an abdominal incision but did not undergo unilateral renal ischemia treatment. The rats (n=3 each group) were then sacrificed to establish the AKI and CKD models 24 hours and 14 days after the establishment of the I/R model, respectively. Serum and kidney tissues were collected for subsequent analyses.
Renal function tests
To analyze renal function, the rats serum was isolated from the whole blood after the end of the experiment, serum CRE and urea were measured using enzyme-linked immunoassay kits (Jiancheng Biotech, Nanjing, China). The operation was performed according to the manufacturer’s instructions. The microplate reader (MB530; HEALES) was used to measure the A values of each group at the wavelengths of 546 nm (CRE), 640 nm (urea). The respective concentrations of CRE and urea were calculated by the kit instruction formula.
Oxidative stress analysis
The renal tissues of rats were isolated and then ground, centrifuged at 8,000 g 4 ℃ for 10 min, and the supernatant was obtained. Corresponding kits (Elabscience Biotechnology, Wuhan, China) were used to determine the production of malondialdehyde (MDA) and superoxide dismutase (SOD) in the kidney tissues. The operation was performed according to the manufacturer’s instructions. After 30 min of 37 ℃ water bath, the absorbance of each tube was measured at 450 and 560 nm by microplate reader (MB530; HEALES).
Histomorphological staining
The kidney tissue was obtained and fixed with 4% paraformaldehyde at room temperature for more than 24 h, and then embedded and sliced into thin slices with a slicer, slices were baked at 62 ℃ for 2–6 h. After dewaxing with xylene, the tissue was stained with hematoxylin-eosin (H&E) and periodic acid-Schiff (PAS). After drying, the slices were mounted and assessed under microscopic photographs (BA210T; MOTIC, Xiamen, China).
TUNEL assays
According to the reagent instructions (Beyotime Biotechnology, Shanghai, China), the tissue sections were incubated with proteinase K working solution, followed by the prepared terminal transferase dUTP nick end labeling (TUNEL) reaction mixture at 37 ℃ for 1 h in a dark box. The sections were then observed in a later analysis by microscopic examination.
IHC staining
The slides were treated with 3% hydrogen peroxide at room temperature for 10 min, blocked with 10% BSA for 40 min, and then incubated with anti-Nrf2 (PA5-27882) or anti-HO-1 (PA5-77833) antibodies (Thermo Fisher Scientific, Waltham, MA, USA) at 4 ℃ overnight. The slides were washed with PBS and incubated with horseradish peroxidase for 40 min, and then stained with diaminobenzidine for 10 min at room temperature. After dehydration, the slides were mounted and observed under a microscope.
QRT-PCR
The RNAi Plus kit (9108, Otsu, Takakawa, Shiga, Japan) was used to extract the total ribonucleic acid (RNA) from the kidney tissue, and the concentration of RNA was determined after purification. Complementary deoxyribonucleic acid (DNA) was obtained using the Takara RT-PCR kit (RR047A, Takara), and messenger RNA (mRNA) levels were quantified using the Takara SYBR q-PCR kit (RR820A, Takara). We performed 40 cycles of RT-PCR reactions using the ABI 7500 system (Thermo Fisher Scientific). GAPDH was used as the internal reference, and the expression of the mRNA levels was normalized to GAPDH. Primer sequences for RT-PCR (Table 1).
Table 1
ID | Primer sequences (5'-3') |
---|---|
Nrf-2 | Forward: 5'-ATTTGTAGATGACCATGAGTCGC-3' |
Reverse: 5'-CTGCCAAACTTGCTCCATGT-3' | |
HO-1 | Forward: 5'-ACGCATATACCCGCTACCTG-3' |
Reverse: 5'-TCTCCAGAGTGTTCATGCGA-3' | |
Bcl-2 | Forward: 5'-GCCTTCTTTGAGTTCGGTGG-3' |
Reverse: 5'-CTCAGTCATCCACAGAGCGA-3' | |
Bax | Forward: 5'-CGAATTGGCGATGAACTGGA-3' |
Reverse: 5'-CCAGTTGAAGTTGCCGTCTG-3' | |
GAPDH | Forward: 5'-CCTCGTCTCATAGACAAGATGGT-3' |
Reverse: 5'-GGGTAGAGTCATACTGGAACATG-3' |
RT-PCR, reverse transcription-polymerase chain reaction.
Western blot
The kidney tissue was cut into small fragments with scissors, and RIPA lysis (P0013, Beyotime Biotechnology) was used to lyse the tissue on ice, after which the tissue was ground with a grinder to make it fully lysed. The supernatant was obtained after high-speed refrigeration centrifugation, and the protein concentration was determined by a BCA kit (CW0130S, CWBIO, Beijing, China). Electrophoresis was carried out through 8–15% SDS-PAGE, and the sample was transferred to PVDF membranes, then blocked at room temperature for 1 h, and subsequently incubated with the primary antibody at 4 ℃ in incubators overnight. The membranes were washed with PBS and then incubated in the secondary antibody at room temperature for 1 h. The membranes were examined using a chemiluminescence system (Beyotime Biotechnology). The following primary antibodies were used: Nrf2 (PA5-27882), HO-1 (PA5-77833), Bcl-2 (PA5-27094), Bax (MA5-14003), and GAPDH (MA5-15738-D680, Thermo Fisher Scientific).
Statistical analysis
The statistical analysis was performed using GraphPad Prism 7. The data are presented as the mean ± standard deviation (SD). Statistical comparisons were carried out by a 1-way analysis of variance, followed by a Tukey’s test. A P value <0.05 was considered statistically significant.
Results
AS-IV protected renal function and alleviated the renal tubular pathological damage caused by I/R
We first assessed renal function and the tubular pathological changes in the I/R-induced AKI rat model. The structure of AS-IV is shown in Figure 1A. As Figure 1B,1C show, the serum CRE and urea levels in the I/R group were markedly elevated, indicating the successful establishment of the I/R model. AS-IV significantly decreased serum CRE and urea levels in a dose-dependent manner. Consistent with the above results, the H&E and PAS staining showed seriously damaged renal tubules tissues, disordered tubular epithelial cells, and increased inflammatory cell infiltration in the I/R group, while AS-IV inhibited these changes to some extent (see Figure 1D,1E). Our results showed the protective effect on the I/R rat model.
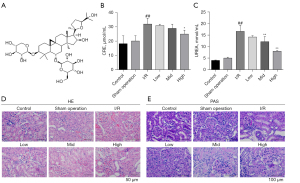
AS-IV treatment relieved I/R-induced apoptosis and regulated the Nrf2 /HO-1 pathway in rat kidney tissues
Cell viability is closely related to tissue function. To explore whether AS-IV exerted a protective effect on cell viability in the I/R-induced AKI rat model, we determined cell apoptosis using TUNEL assays. The results showed that renal cell apoptosis was significantly more alleviated in the AS-IV treatment groups, especially the high-dose group, than the I/R group (see Figure 2A). Next, we sought to examine whether AS-IV exerted a protective effect by regulating the Nrf2/HO-1 signaling pathway. The immunohistochemical (IHC) assay results showed that the expression of Nrf2 and HO-1 in the I/R group was decreased, but later increased after AS-IV treatment (see Figure 2B,2C). The qRT-PCR results further confirmed that the expression of the anti-apoptosis factor (Bcl-2) was downregulated and the expression of the pro-apoptosis factor (Bax) was upregulated in the I/R group, while AS-IV treatment suppressed these trends (see Figure 2D,2E), and the AS-IV treatment reversed the decreased expression of Nrf2 and HO-1 caused by I/R (see Figure 2F,2G). These results demonstrated that the AS-IV treatment decreased I/R-induced cell apoptosis in the kidneys. Consistent with these results, the western blot results also showed that the AS-IV treatment reversed the decreased expression of Nrf2 and HO-1 caused by I/R (see Figure 2H-2J), the anti-apoptosis factor (Bcl-2) was downregulated and the expression of the pro-apoptosis factor (Bax) was upregulated in the I/R group (Figure 2K,2L). Our data indicated that AS-IV relieved cell apoptosis, and this effect was partially related to the regulation of the Nrf2/HO-1 pathway in the I/R rat model.
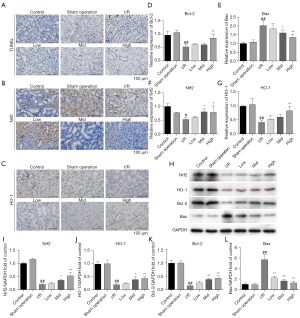
AS-IV treatment alleviated lipid peroxidation in I/R-induced rat kidney damage
Lipid peroxidation alters the structural integrity of the cell membrane and causes an oxidative stress response, which in turn leads to cell death. We also investigated whether AS-IV regulated the lipid peroxidation response in AKI injury. We found that the increased MDA level and the decreased SOD activity in the I/R group were reversed after AS-IV treatment. Thus, AS-IV treatment had a protective effect to suffer from oxygen free radicals damage and alleviated I/R-induced kidney lipid peroxidation (see Figure 3A,3B).
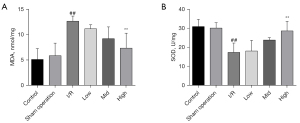
AS-IV treatment protected renal function and protected against pathological damage in the I/R-induced CDK model
We sought to explore whether AS-IV continued to protect the kidneys if the establishment of the I/R model lasted for a longer time. To our surprise, even 14 days after the establishment of the I/R model, AS-IV exerted protective effects on kidney function and structure. Consistent with previous results, we found that the serum CRE and urea levels were elevated in the I/R group and that these levels decreased after AS-IV treatment (see Figure 4A,4B). The H&E and PAS staining showed damaged renal tubules tissues with accumulated inflammatory cell infiltration and disordered epithelial cells in the I/R group, while the AS-IV treatment alleviated the pathological damage (see Figure 4C,4D). These results indicated that AS-IV treatment provided protection against the I/R-induced CKD.
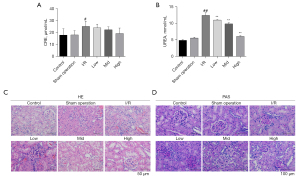
I/R-induced CKD model
Next, we examined the renal cell apoptosis and the expression of the Nrf2/HO-1 pathway in the CKD model. Consistent with the results for the AKI model, renal cell apoptosis was also more alleviated in the AS-IV treatment group than the I/R group (see Figure 5A). The IHC results showed that the expression of Nrf2 and HO-1 in the I/R group were dramatically reduced, but increased after AS-IV treatment (see Figure 5B,5C). Additionally, the qRT-PCR results showed that the expression of the downregulated anti-apoptosis factor (Bcl-2) and the upregulated pro-apoptosis factor (Bax) in the I/R group were inhibited by the AS-IV treatment (see Figure 5D,5E), and the AS-IV treatment reversed the decreased expression of Nrf2 and HO-1 caused by I/R (see Figure 5F,5G), which indicates that AS-IV protects against I/R-induced apoptosis in CKD. Further, the expression of the Nrf2/HO-1 pathway in the CKD model was similar to that in the AKI model. The western blot results further confirmed the same results as those found for the IHC and q-PCR assays (see Figure 5H-5L). Our data indicated that AS-IV has a protective effect against apoptosis and oxidative stress in CKI.
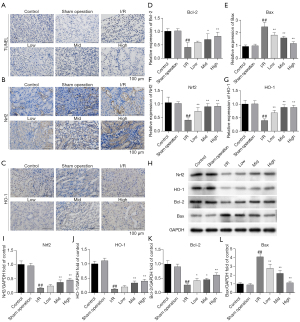
AS-IV attenuated renal lipid peroxidation in the I/R-induced CKD model
Similarly, we examined the effect of AS-IV on lipid peroxidation in the CKD model. We found that 14 days after the establishment of I/R model, the rats in the I/R group had an elevated level of MDA and decreased SOD activity; however, AS-IV treatment improved these trends (see Figure 6A,6B), just as it did in the AKI model. Our data showed that AS-IV alleviated renal lipid peroxidation in the CKD model.
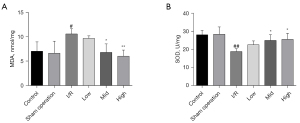
Discussion
Studies have shown that AS-IV leads to improvements in a variety of kidney diseases. Ju et al. reported that AS-IV protected rats from diabetic nephropathy by regulating the apoptosis of the endoplasmic reticulum pathway (21). AS-IV has also been shown to alleviate cisplatin-caused kidney damage by regulating the autophagy pathway (22). Further, AS-IV relieves oxidative stress, which in turn decreases the tubulointerstitial damage induced by indoxyl sulfate (23). However, it was unclear whether AS-IV would have a protective effect on AKI and CKI.
According to a previous study, left renal ischemia for 45 minutes combined with right renal nephrectomy effectively establishes an I/R rat model (20). Our results showed that AS-IV exerted a protective effect against AKI and CKI partially by regulating the antiapoptotic and antioxidative stress pathway.
Apoptosis is part of programmed cell death, which aims to maintain a cell microenvironmental balance (24-26). Several studies have shown that apoptosis is involved in the pathogenesis of renal diseases (27). Apoptosis has two pathways (i.e., exogenous and endogenous) (28), and the endogenous pathway can be further divided into mitochondrial pathways and endoplasmic reticulum pathways (29-31). In our study, as the key factor in mitochondrial apoptosis, the decreased of Bcl-2 and the upregulation of Bax in the I/R-induced AKI and CKD models might indicate the occurrence of mitochondrial apoptosis. It may be that AS-IV suppresses cell apoptosis in kidney injury by regulating mitochondrial apoptosis.
Previous studies have shown that oxidative stress is related to the pathogenesis of AKI. Notably, Xia et al. reported that honokiol plays a protective role in AKI through the regulation of peroxidative stress (32). Progressive CKD and its complications are closely associated with increased levels of oxidative stress (33). It is well known that decreased SOD and increased MDA are considered the biomarkers of oxidative stress. In our study, AS-IV increased SOD activity and decreased MDA content in the AKI and CKD models, which suggests that it has antioxidant effects in I/R-induced renal injury.
The activation of Nrf2 plays a vital role in the antioxidant system (34). Dai et al. found Scu led to up-regulation of HO-1 through activating the Nrf2 signalling pathway, protecting the kidneys from ischemia/reperfusion (I/R)-induced oxidative damage (35). Zhang et al. Studies demonstrated that UA can alleviate oxidative stress and promote autophagy by activating the p62-Keap1-Nrf2 signaling pathway, which could protect the kidneys from ischemia reperfusion injury (36). In the Nrf2/ARE/HO-1 signaling pathway, Nrf2 regulates the expression of HO-1 by binding to ARE (37). SAL effectively alleviated renal function and ameliorated epithelial tubular injury, oxidative stress, and inflammatory response via anti-apoptotic effects through activation of the Keap1-Nrf2-ARE signaling pathway in renal tubular cells (38). In our study, various experimental methods resulted in decreased expression levels of Nrf2 and HO-1 in the I/R-induced AKI and CKD models. It may be that I/R in the kidney decreases the expression of Nrf2 and its downstream factor HO-1, which in turn weakens their interactions and leads to antioxidant stress dysfunction. Interestingly, treatment with AS-IV was found to upregulate the expression of Nrf2 and HO-1, which suggests that AS-IV might partially exert its protective effects by regulating the Nrf2/HO-1 pathway. However, more research on this issue needs to be conducted in the future. Zhang et al. found that Irisin treatment alleviated renal injury, downregulated inflammatory response, improved mitochondrial function, and reduced ER stress and oxidative stress after renal I/R, which were associated with upregulation of GPX4 (39). Zheng et al. Studies demonstrated that Porous Se@SiO2 nanospheres exhibited a protective effect in I/R-induced AKI by resisting oxidative stress and inflammation (20). Up to now, there are many literatures about Astragaloside IV, and Astragaloside IV is widely used in clinic with less side effect, and it has extensive anti-inflammatory and enhancing immune function, so its clinical application value is high.
In summary, we found that AS-IV protected kidney function in I/R-induced AKI and CKD rat models, and it might partially achieve this through the antiapoptotic and antioxidative response. Our findings indicate that AS-IV is a promising drug for treating kidney injury.
Acknowledgments
Funding: This work was supported by the Guangzhou Medical Key Discipline Construction Project (No. 2021-2023), the Guangzhou Health Science and Technology Project (No. 20202A010022), the Guangzhou Huadu District Science and Technology Project (No. 20-HDWS-024), the Binzhou Medical University “Clinical + X” Science and Technology Innovation Projects (No. BYLCX24), the Binzhou Medical University Xu Rongxiang Foundation (No. BY2020XRX05), the Health Science and Technology Project of Guangzhou (No. 20211A011113), and the Guangzhou Science and Technology project (Nos. 202102080616 and 202201011649).
Footnote
Reporting Checklist: The authors have completed the ARRIVE reporting checklist. Available at https://tau.amegroups.com/article/view/10.21037/tau-22-505/rc
Data Sharing Statement: Available at https://tau.amegroups.com/article/view/10.21037/tau-22-505/dss
Conflicts of Interest: All authors have completed the ICMJE uniform disclosure form (available at https://tau.amegroups.com/article/view/10.21037/tau-22-505/coif). The authors have no conflicts of interest to declare.
Ethical Statement: The authors are accountable for all aspects of the work, including ensuring that any questions related to the accuracy or integrity of any part of the work have been appropriately investigated and resolved. Animal experiments were performed under a project license (No. 2019001) granted by the ethics committee of Southern Medical University (People’s Hospital of Huadu District), in compliance with national guidelines for the care and use of animals.
Open Access Statement: This is an Open Access article distributed in accordance with the Creative Commons Attribution-NonCommercial-NoDerivs 4.0 International License (CC BY-NC-ND 4.0), which permits the non-commercial replication and distribution of the article with the strict proviso that no changes or edits are made and the original work is properly cited (including links to both the formal publication through the relevant DOI and the license). See: https://creativecommons.org/licenses/by-nc-nd/4.0/.
References
- Huang J, Li J, Lyu Y, et al. Molecular optical imaging probes for early diagnosis of drug-induced acute kidney injury. Nat Mater 2019;18:1133-43. [Crossref] [PubMed]
- Jagarlamudi N, Wong F. Acute kidney injury: prediction, prognostication and optimisation for liver transplant. Hepatol Int 2020;14:167-79. [Crossref] [PubMed]
- Ogbadu J, Singh G, Aggarwal D. Factors affecting the transition of acute kidney injury to chronic kidney disease: Potential mechanisms and future perspectives. Eur J Pharmacol 2019;865:172711. [Crossref] [PubMed]
- Menez S, Parikh CR. Assessing the health of the nephron in acute kidney injury: biomarkers of kidney function and injury. Curr Opin Nephrol Hypertens 2019;28:560-6. [Crossref] [PubMed]
- Wang S, Yang J, Chen X, et al. The risk factors of acute kidney injury caused by hemophagocytic lymphohistiocytosis. Nephrology Dialysis Transplantation 2021; [Crossref]
- Kieneker LM, Hartmann O, Bergmann A, et al. Proenkephalin and risk of developing chronic kidney disease: the Prevention of Renal and Vascular End-stage Disease study. Biomarkers 2018;23:474-82. [Crossref] [PubMed]
- Li HD, Meng XM, Huang C, et al. Application of Herbal Traditional Chinese Medicine in the Treatment of Acute Kidney Injury. Front Pharmacol 2019;10:376. [Crossref] [PubMed]
- Liu ZZ, Weng HB, Zhang LJ, et al. Bupleurum polysaccharides ameliorated renal injury in diabetic mice associated with suppression of HMGB1-TLR4 signaling. Chin J Nat Med 2019;17:641-9. [Crossref] [PubMed]
- Zhang B, Zeng M, Li M, et al. Protopine Protects Mice against LPS-Induced Acute Kidney Injury by Inhibiting Apoptosis and Inflammation via the TLR4 Signaling Pathway. Molecules 2019;25:15. [Crossref] [PubMed]
- Zhang J, Wu C, Gao L, et al. Astragaloside IV derived from Astragalus membranaceus: A research review on the pharmacological effects. Adv Pharmacol. 2020;87:89-112. [Crossref] [PubMed]
- Deng LL. Astragaloside IV as Potential Antioxidant Against Diabetic Ketoacidosis in Juvenile Mice Through Activating JNK/Nrf2 Signaling Pathway. Arch Med Res 2020;51:654-63. [Crossref] [PubMed]
- Ding Q, Gao J, Zheng J, et al. Astragaloside IV attenuates inflammatory injury and promotes odontoblastic differentiation in lipopolysaccharide-stimulated MDPC-23 cells and rat pulpitis. J Oral Pathol Med 2019;48:951-8. [Crossref] [PubMed]
- Li Y, Ye Y, Chen H. Astragaloside IV inhibits cell migration and viability of hepatocellular carcinoma cells via suppressing long noncoding RNA ATB. Biomed Pharmacother 2018;99:134-41. [Crossref] [PubMed]
- Zhou XT, Zou JJ, Ao C, et al. Renal protective effects of astragaloside IV, in diabetes mellitus kidney damage animal models: A systematic review, meta-analysis. Pharmacol Res 2020;160:105192. [Crossref] [PubMed]
- Nezu M, Suzuki N, Yamamoto M. Targeting the KEAP1-NRF2 System to Prevent Kidney Disease Progression. Am J Nephrol 2017;45:473-83. [Crossref] [PubMed]
- Ogura Y, Kitada M, Xu J, et al. CD38 inhibition by apigenin ameliorates mitochondrial oxidative stress through restoration of the intracellular NAD+/NADH ratio and Sirt3 activity in renal tubular cells in diabetic rats. Aging (Albany NY) 2020;12:11325-36. [Crossref] [PubMed]
- Lv W, Booz GW, Fan F, et al. Oxidative Stress and Renal Fibrosis: Recent Insights for the Development of Novel Therapeutic Strategies. Front Physiol 2018;9:105. [Crossref] [PubMed]
- Pang Y, Zhang PC, Lu RR, et al. Andrade-Oliveira Salvianolic Acid B Modulates Caspase-1-Mediated Pyroptosis in Renal Ischemia-Reperfusion Injury via Nrf2 Pathway. Front Pharmacol 2020;11:541426. [Crossref] [PubMed]
- Aladaileh SH, Hussein OE, Abukhalil MH, et al. Formononetin Upregulates Nrf2/HO-1 Signaling and Prevents Oxidative Stress, Inflammation, and Kidney Injury in Methotrexate-Induced Rats. Antioxidants (Basel) 2019;8:430. [Crossref] [PubMed]
- Zheng Z, Deng G, Qi C, et al. Porous Se@SiO2 nanospheres attenuate ischemia/reperfusion (I/R)-induced acute kidney injury (AKI) and inflammation by antioxidative stress. Int J Nanomedicine 2018;14:215-29. [Crossref] [PubMed]
- Ju Y, Su Y, Chen Q, et al. Protective effects of Astragaloside IV on endoplasmic reticulum stress-induced renal tubular epithelial cells apoptosis in type 2 diabetic nephropathy rats. Biomed Pharmacother 2019;109:84-92. [Crossref] [PubMed]
- Qu X, Gao H, Tao L, et al. Astragaloside IV protects against cisplatin-induced liver and kidney injury via autophagy-mediated inhibition of NLRP3 in rats. J Toxicol Sci 2019;44:167-75. [Crossref] [PubMed]
- Ji C, Luo Y, Zou C, et al. Effect of astragaloside IV on indoxyl sulfate-induced kidney injury in mice via attenuation of oxidative stress. BMC Pharmacol Toxicol 2018;19:53. [Crossref] [PubMed]
- Wu J, Ye J, Kong W, et al. Programmed cell death pathways in hearing loss: A review of apoptosis, autophagy and programmed necrosis. Cell Prolif 2020;53:e12915. [Crossref] [PubMed]
- Nagata S. Apoptosis and Clearance of Apoptotic Cells. Annu Rev Immunol 2018;36:489-517. [Crossref] [PubMed]
- Song B, Zhou T, Liu J, et al. Involvement of Programmed Cell Death in Neurotoxicity of Metallic Nanoparticles: Recent Advances and Future Perspectives. Nanoscale Res Lett 2016;11:484. [Crossref] [PubMed]
- Wen S, Wang ZH, Zhang CX, et al. Caspase-3 Promotes Diabetic Kidney Disease Through Gasdermin E-Mediated Progression to Secondary Necrosis During Apoptosis. Diabetes Metab Syndr Obes 2020;13:313-23. [Crossref] [PubMed]
- Cho HD, Lee JH, Moon KD, et al. Auriculasin-induced ROS causes prostate cancer cell death via induction of apoptosis. Food Chem Toxicol 2018;111:660-9. [Crossref] [PubMed]
- Song J, Zhang Q, Wang S, et al. Cleavage of caspase-12 at Asp94, mediated by endoplasmic reticulum stress (ERS), contributes to stretch-induced apoptosis of myoblasts. J Cell Physiol 2018;233:9473-87. [Crossref] [PubMed]
- Kim K, Kenigsberg S, Jurisicova A, et al. The Role of Mitochondria in Oocyte and Early Embryo Health. OBM Genetics 2019;3:70. [Crossref]
- Qian RH, Zhu XH, Hao JM, et al. Essential oil from Chenopodium ambrosioides L. induces mitochondrial-mediated pathway and endoplasmic reticulum stress-related apoptosis in human liver cancer SMMC-7721 cells. Tropical Journal of Pharmaceutical Research 2020;19:837-43. [Crossref]
- Xia S, Lin H, Liu H, et al. Honokiol Attenuates Sepsis-Associated Acute Kidney Injury via the Inhibition of Oxidative Stress and Inflammation. Inflammation 2019;42:826-34. [Crossref] [PubMed]
- Daenen K, Andries A, Mekahli D, et al. Oxidative stress in chronic kidney disease. Pediatr Nephrol 2019;34:975-91. [Crossref] [PubMed]
- ALHaithloul HAS. Olea europaea leaf extract up-regulates Nrf2/ARE/HO-1 signaling and attenuates cyclophosphamide-induced oxidative stress, inflammation and apoptosis in rat kidney. Biomed Pharmacother 2019;111:676-85. [Crossref] [PubMed]
- Dai J, Li C, Zhao L, et al. Scutellarin protects the kidney from ischemia/reperfusion injury by targeting Nrf2. Nephrology (Carlton) 2022;27:690-700. [Crossref] [PubMed]
- Zhang Y, Liu M, Zhang Y, et al. Urolithin A alleviates acute kidney injury induced by renal ischemia reperfusion through the p62-Keap1-Nrf2 signaling pathway. Phytother Res 2022;36:984-95. [Crossref] [PubMed]
- Liu X, Zhu Q, Zhang M, et al. Isoliquiritigenin Ameliorates Acute Pancreatitis in Mice via Inhibition of Oxidative Stress and Modulation of the Nrf2/HO-1 Pathway. Oxid Med Cell Longev 2018;2018:7161592. [Crossref] [PubMed]
- Sun D, Cui S, Ma H, et al. Salvianolate ameliorates renal tubular injury through the Keap1/Nrf2/ARE pathway in mouse kidney ischemia-reperfusion injury. J Ethnopharmacol. 2022;293:115331. [Crossref] [PubMed]
- Zhang J, Bi J, Ren Y, et al. Involvement of GPX4 in irisin's protection against ischemia reperfusion-induced acute kidney injury. J Cell Physiol 2021;236:931-45. [Crossref] [PubMed]
(English Language Editor: L. Huleatt)