Glycated serum albumin decreases connexin 43 phosphorylation in the corpus cavernosum
Introduction
Erectile dysfunction (ED) is the persistent inability to attain or maintain an erection satisfactory for sexual performance (1,2). ED most often affects men older than 40 years, and the prevalence increases with age. ED is reported in 2% to 12% of men aged 40 to 49 years and 50% to 100% of men older than 70 years (1). The causes of ED include vascular, neurogenic, endocrinologic, and psychogenic disorders, congenital and acquired anatomic anomalies, surgical complications, trauma, respiratory, liver, and renal diseases, medications, and recreational substances. ED shares some risk factors (modifiable and non-modifiable) with cardiovascular disease (1,2). The potentially modifiable risk factors include smoking, lack of exercise, diabetes, hypertension, and obesity (2). Those risk factors are associated with endothelial injury and dysfunction, which are involved in ED (1,2).
Nitric oxide (NO) plays a central role in vascular function and penile erection (3) and is produced by the endothelial NO synthase (eNOS). The phosphorylation of eNOS is one of the hallmarks of endothelial cell (EC) bioactivity, and restoration of the serine-threonine kinase (Akt)-eNOS signaling reduces diabetic cardiomyopathy and myocardial dysfunction (4). Connexin 43 (Cx43) is widely found in ECs and smooth muscle cells (SMCs). Cx43 is a receptor essential for maintaining endothelial continuity and integrity (5,6). Various kinases regulate the phosphorylation of Cx43. For instance, PKA phosphorylates the S368 site of Cx43 (7), while the S262 and S279/282 sites are phosphorylated by PKC (8) and MAPK (9), respectively. Cx43 phosphorylation can alter the conductivity and permeability of vascular endothelial gap junction (GJ) channels (5,10,11). The phosphorylation of eNOS and Cx43 is regulated by the phosphatidylinositol 3-kinase (PI3K)-Akt signaling pathway (12).
Glycated serum albumin (GSA) is an early glycosylation product that mainly reflects the blood glucose control level over the past 2–3 weeks and is considered a complementary marker for evaluating glycemic control in type 1 and 2 diabetes (13-16). It is also involved in the damages associated with diabetes mellitus, such as retinopathy, nephropathy, neuropathy, and coronary artery disease (14,17,18). GSA has been associated with diabetic nephropathy, microangiopathy (19), and diabetic retinopathy (16). In addition, GSA correlates with the generally recognized plasma markers of endothelial or vascular dysfunction (20,21). Microvascular damage due to elevated GSA can occur in the early stages of abnormal glucose metabolism (22), arteriosclerosis (23), and neurodegenerative diseases (24). In a previous study by our group, glycosylated serum protein (GSP) was positively correlated with the severity of ED in patients without diabetes, and the patients with high levels of GSP had a lower endothelial function (25). Hence, elevated GSA levels and the associated damage to the corpus cavernosum might be limited to not only diabetic patients but also non-diabetic patients and even individuals with normal glucose levels.
However, whether GSA can cause early endothelium damage to the cardiovascular system is still unproven. In addition, whether GSA injures the vascular endothelium by downregulating the phosphorylation of eNOS and Cx43 through the PI3K/Akt pathway (Figure 1) is unclear. Therefore, this study aimed to examine the role of GSA in early damage to the corpus cavernosum and identify the pathways involved in GSA-induced endothelium damage. We present the following article in accordance with the ARRIVE reporting checklist (available at https://tau.amegroups.com/article/view/10.21037/tau-22-317/rc).
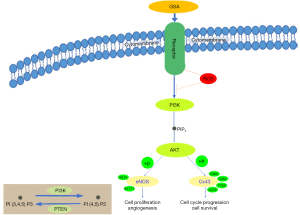
Methods
Experimental animals
Male Sprague-Dawley (SD) rats were acquired from the animal center of Shanghai Jiao Tong University School of Medicine. This study was approved by the Institutional Animal Care and Use Committee of Shanghai Jiao Tong University of Medicine (No. HKDL[2016]149). All procedures were conducted in accordance with the institutional guidelines for animal care and use. A protocol was prepared with registration before the start of the study. All efforts were made to minimize the suffering and the number of animals used. The rats were housed under a 12-h light/dark cycle with free access to food and water for 10 days prior to the experiments. A total of nine male 8-week-old SD rats weighing 250–300 g were divided into two groups: the control (n=3) and GSA (n=6) groups. The rats in the control and GSA groups were injected daily with saline vehicle or GSA 200 µg/kg through the tail vein for 4 weeks respectively (26). After treatment, the rats were anesthetized using an intraperitoneal injection of phenobarbital sodium (40 mg/kg), and the tissue specimens were taken.
Cell culture
Human umbilical vein ECs (HUVECs) were isolated from human umbilical cords, which were purchased from American Type Culture Collection (ATCC, Manassas, VA, USA). The HUVECs were cultured in Dulbecco’s Modified Eagle Medium containing 10% fetal bovine serum (GIBCO, Invitrogen Inc., Carlsbad, CA, USA), supplemented with penicillin 100 U/mL and streptomycin 100 mg/mL. The cells were incubated at 37 ℃ in a humidified atmosphere with 5% CO2. The HUVECs were treated with GSA at different concentrations and durations. In the concentration groups, the cells were treated for 12 h with GSA at 0, 0.5, 1, or 2 µg/mL. In the duration groups, the cells were treated with 1 µg/mL GSA for 0, 6, 12, or 24 h (27,28).
Immunohistochemistry
The corpus cavernosum, heart, and brain stem tissues were harvested, fixed in 4% paraformaldehyde solution, and paraffin-embedded for histological studies. Sections were cut at 5 µm for analysis. For immunohistochemistry, the sections were detected with primary antibodies against p-Cx43 (S262) (1:100; sc-17219-R; Santa Cruz Biotechnology, Santa Cruz, CA, USA), p-eNOS (1:100; sc-376542; Santa Cruz Biotechnology), p-PI3K (1:100; #17366; Cell Signaling Technology, Inc., Danvers, MA, USA), and p-Akt (1:100; #4691; Cell Signaling Technology, Inc.) overnight at 4 ℃. After incubation with the appropriate secondary antibodies, the sections were developed with diaminobenzidine and counterstained with hematoxylin. Quantitative image analysis was done at ×40 magnification. Histological images were captured using a Zeiss Axio Imager 2 microscope (Zeiss, Thornwood, NY, USA).
Western blot
Tissues or cultured cells were lysed using the radioimmunoprecipitation assay (RIPA) lysis buffer (Beyotime, Nantong, China). The bicinchoninic acid assay (Thermo Fisher Scientific, Inc., Waltham, MA, USA) was used to determine the protein concentrations. Total protein extract (20 µg) was separated by 10% sodium dodecyl sulfate-polyacrylamide gel electrophoresis and transferred to polyvinylidene difluoride membranes (Millipore, Bedford, MA, USA). The protein binding sites on the membranes were blocked with 5% bovine serum albumin (BSA) (Biosharp, Hefei, China). The membranes were gently agitated and incubated with antibodies against Cx43 (1:1,000; ab11370; Abcam, Cambridge, UK), p-Cx43 (1:1,000; ab30559; Abcam), eNOS (1:1,000; #32027; Cell Signaling Technology, Inc.), p-eNOS (1:1,000; sc-376542; Cell Signaling Technology, Inc.), Akt (1:1,000; #4691; Cell Signaling Technology, Inc.), p-Akt (1:1,000; #4060; Cell Signaling Technology, Inc.), PI3K (1:1,000; #4249; Cell Signaling Technology, Inc.), p-PI3K (1:1,000; #17366; Cell Signaling Technology, Inc.), p-Cx43 (S368) (1:1,000; ab194928; Abcam), p-Cx43 (S262) (1:1,000; sc-17219-R; Santa Cruz Biotechnology), p-Cx43 (Y265) (1:1,000; ab193373; Abcam), p-Cx43 (S255) (1:1,000; sc-12899; Santa Cruz Biotechnology), and p-Cx43 (S279/282) (1:1,000; sc-13621; Santa Cruz Biotechnology) at 4 ℃ overnight. After incubation with the appropriate secondary antibodies, the sections were developed with diaminobenzidine. The results were quantified by densitometry and normalized to glycolytic enzyme glyceraldehyde 3-phosphate dehydrogenase (GAPDH) expression. Immunoreactive bands were quantitatively analyzed with Image J software version 1.4.3.67 (National Institutes of Health, Bethesda, MD, USA).
Cx43 overexpression
The pCDNA3-Cx43 plasmid was generated by cloning the coding sequence of the constitutively active Cx43 into the pCDNA3 expression vector according to the manufacturer’s protocol (Invitrogen Inc.). For Cx43 overexpression, the HUVECs were transfected using Lipofectamine 2000 (Invitrogen Inc.), according to the manufacturer’s instructions.
Statistical analysis
All variables were presented as means ± standard deviation. The differences among groups were analyzed with Student’s t-test and Mann-Whitney U-test or Kruskal-Wallis test (nonparametric data). P value <0.05 was considered statistically significant. All the statistics were conducted using Statistical Package for Social Sciences (SPSS) (version 22.0 for Windows).
Results
Expression of phosphorylated PI3K/Akt, Cx43, and eNOS in the penis, heart, and brain stem of control and GSA rats
The expression levels of p-Cx43, p-eNOS, p-PI3K, and p-Akt were measured in the penis, heart, and brain of the rats after treatment with GSA to determine the impact of GSA on those proteins involved in endothelial function. In the penis (Figure 2) and heart (Figure S1), the expression levels of p-Cx43, p-eNOS, p-PI3K, and p-Akt were significantly lower in GSA-treated rats than in control rats. In the brain (Figure S2), the expression levels of p-Cx43 and p-Akt were lower in the GSA group than in the vehicle group, but the expression of p-eNOS and p-PI3K showed no significant differences between the two groups. Of note, the expression of p-Cx43 was lower in the three organs of the GSA rats than in controls. Compared with the heart and brain, penile tissue showed a more significant decrease in the phosphorylation expression of EC function proteins after GSA treatment (Figure 1 and Figures S1,S2).
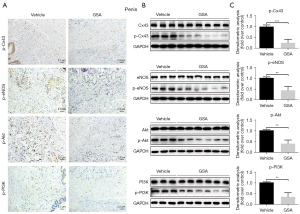
Decreased phosphorylation of PI3K/Akt, Cx43, and eNOS in HUVECs treated with GSA
HUVECs were treated with GSA at different concentrations and for different durations. The expression levels of p-Cx43, p-eNOS, p-PI3K, and p-Akt (all proteins involved in endothelial function) were examined. p-Cx43, p-eNOS, p-PI3K, and p-Akt were decreased in HUVECs exposed to GSA in dose- and time-dependent manners (Figure 3). Higher doses and longer durations inhibited the expression of all four proteins. The most significant impact was observed with 1 µg/mL of GSA for 12 h.
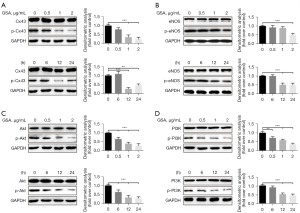
Phosphorylation sites of Cx43 in HUVECs treated with GSA
The phosphorylation of Cx43 at different sites is regulated by various kinases (7-9). Since the function of Cx43 depends on the phosphorylation position, overexpression experiments of Cx43 were performed to investigate which phosphorylation sites were regulated by GSA. The results showed that the phosphorylation of the S368, S262, Y265, S255, and S279/282 sites was downregulated by GSA. Among them, the suppressive effects of GSA were more important on the S368 phosphorylation site than on other sites (Figure 4).
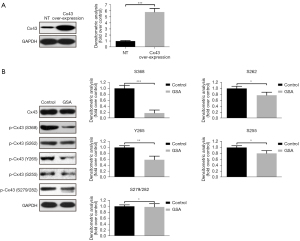
Discussion
GSA is an early glycosylation product that participates in diabetic vascular complications (13,14,16,19). This study examined the role of GSA in early damage to the corpus cavernosum and the involved mechanisms. The results showed that GSA decreased the expression of phosphorylated Cx43 in the corpus cavernosum of rats. This effect might be related to the decreased phosphorylation of p-eNOS, p-PI3K, and p-Akt, as well as by the downregulation of the phosphorylation at the Cx43 S368 site in the corpus cavernosum. Similar results were also observed in the heart and brain. Taken together, endothelial dysfunction could play a role in ED since the risk factors for ED are the same as for cardiovascular diseases.
GSA can induce vascular damage in patients with diabetes (17,18) and in individuals with pre-diabetes and normal glucose levels (22). GSA-induced damage is involved in arteriosclerosis (23), neurodegenerative diseases (24), ED (25), and retinopathy (29). In the present study, GSA induced damage to the penis, heart, and brain, as supported by previous studies (17,18,25). Moreover, the phosphorylated levels of endothelial function markers in the penis (especially Cx43 and eNOS) were more decreased than in the brain, which might suggest that the microvascular system of the penis is more susceptible to being damaged by GSA than the brain.
The role of GSA in endothelial damage still needs to be clarified. Some in vivo studies demonstrated that the endothelium damage induced by high concentrations of GSA was related to the excessive production of reactive oxygen species (ROS) mediated by the upregulation of the NADPH oxidase (Nox), particularly the main subunit of Nox4 (28), and by NF-κB activation and eNOS uncoupling (20). In vitro, GSA can cause retinal pigment epithelium (RPE) function breakdown, which is mediated by specific receptor for advanced glycation end-products (RAGE) and vascular endothelial growth factor receptor 2 (VEGFR2) signaling (30). Amore et al. (31) also found that GSA at concentrations similar to those in diabetic patients induces the NO-dependent apoptosis in ECs. The present study showed that the GSA-induced endothelial dysfunction might be due to a downregulation of eNOS and Cx43 phosphorylation. These findings might support the clarification of the mechanisms of vascular EC injury induced by GSA.
Cx43 has been identified to be important for cardiac and brain ischemia/reperfusion injuries and is affected by age and sex as well as by several pathophysiological alterations such as hypertension, hypertrophy, diabetes, hypercholesterolemia, and ischemia (32). This in vivo study also showed that Cx43 is the most significant injury marker for vascular damage induced by GSA in the penis. Cx43 can be modified by nitros(yl)ation and phosphorylation/de-phosphorylation, modulating the channel activity of the GJ (5). Cx43 is a phosphoprotein with clear evidence for phosphorylation at more than 12 serine (S) and tyrosine (Y) sites in the C-terminal tails (CT) region of the protein via at least six kinases (33,34). The present study demonstrated that GSA downregulated the phosphorylation at the S368, S262, Y265, S255, and S279/282 sites at various degrees. In particular, S368 was the most remarkable phosphorylation site in the suppressive effects of GSA. Cx43 phosphorylation plays a key role in the modulation of the response to injury in the endothelium, and these findings might translate into therapeutic applications in the future.
In conclusion, the early glycosylation product GSA can injure the vascular endothelium in the penis by decreasing the phosphorylated levels of Cx43. The S368 and Y265 of Cx43 were the main suppressive phosphorylation sites in GSA-damaged ECs. Those results might suggest the importance of Cx43 as a therapeutic target in cardiovascular and corpus cavernosum protection.
Acknowledgments
Funding: This work was supported by grants from the National Natural Science Foundation of China (Nos. 81771577, 81401196).
Footnote
Reporting Checklist: The authors have completed the ARRIVE reporting checklist. Available at https://tau.amegroups.com/article/view/10.21037/tau-22-317/rc
Data Sharing Statement: Available at https://tau.amegroups.com/article/view/10.21037/tau-22-317/dss
Conflicts of Interest: All authors have completed the ICMJE uniform disclosure form (available at https://tau.amegroups.com/article/view/10.21037/tau-22-317/coif). The authors have no conflicts of interest to declare.
Ethical Statement: The authors are accountable for all aspects of the work in ensuring that questions related to the accuracy or integrity of any part of the work are appropriately investigated and resolved. This study was approved by the Institutional Animal Care and Use Committee of Shanghai Jiao Tong University of Medicine (No. HKDL[2016]149). All procedures were conducted in accordance with the institutional guidelines for animal care and use. A protocol was prepared with registration before the start of the study.
Open Access Statement: This is an Open Access article distributed in accordance with the Creative Commons Attribution-NonCommercial-NoDerivs 4.0 International License (CC BY-NC-ND 4.0), which permits the non-commercial replication and distribution of the article with the strict proviso that no changes or edits are made and the original work is properly cited (including links to both the formal publication through the relevant DOI and the license). See: https://creativecommons.org/licenses/by-nc-nd/4.0/.
References
- Shamloul R, Ghanem H. Erectile dysfunction. Lancet 2013;381:153-65. [Crossref] [PubMed]
- Salonia A, Bettocchi C, Boeri L, et al. European Association of Urology Guidelines on Sexual and Reproductive Health-2021 Update: Male Sexual Dysfunction. Eur Urol 2021;80:333-57. [Crossref] [PubMed]
- Terentes-Printzios D, Ioakeimidis N, Rokkas K, et al. Interactions between erectile dysfunction, cardiovascular disease and cardiovascular drugs. Nat Rev Cardiol 2022;19:59-74. [Crossref] [PubMed]
- Lei S, Su W, Xia ZY, et al. Hyperglycemia-Induced Oxidative Stress Abrogates Remifentanil Preconditioning-Mediated Cardioprotection in Diabetic Rats by Impairing Caveolin-3-Modulated PI3K/Akt and JAK2/STAT3 Signaling. Oxid Med Cell Longev 2019;2019:9836302. [Crossref] [PubMed]
- Solan JL, Lampe PD. Connexin43 phosphorylation: structural changes and biological effects. Biochem J 2009;419:261-72. [Crossref] [PubMed]
- Song YN, Zhang H, Zhao JY, et al. Connexin 43, a new therapeutic target for cardiovascular diseases. Pharmazie 2009;64:291-5. [PubMed]
- Totland MZ, Rasmussen NL, Knudsen LM, et al. Regulation of gap junction intercellular communication by connexin ubiquitination: physiological and pathophysiological implications. Cell Mol Life Sci 2020;77:573-91. [Crossref] [PubMed]
- Doble BW, Dang X, Ping P, et al. Phosphorylation of serine 262 in the gap junction protein connexin-43 regulates DNA synthesis in cell-cell contact forming cardiomyocytes. J Cell Sci 2004;117:507-14. [Crossref] [PubMed]
- Johnstone SR, Ross J, Rizzo MJ, et al. Oxidized phospholipid species promote in vivo differential cx43 phosphorylation and vascular smooth muscle cell proliferation. Am J Pathol 2009;175:916-24. [Crossref] [PubMed]
- Laird DW. The gap junction proteome and its relationship to disease. Trends Cell Biol 2010;20:92-101. [Crossref] [PubMed]
- Straub AC, Johnstone SR, Heberlein KR, et al. Site-specific connexin phosphorylation is associated with reduced heterocellular communication between smooth muscle and endothelium. J Vasc Res 2010;47:277-86. [Crossref] [PubMed]
- Graupera M, Potente M. Regulation of angiogenesis by PI3K signaling networks. Exp Cell Res 2013;319:1348-55. [Crossref] [PubMed]
- Yoshiuchi K, Matsuhisa M, Katakami N, et al. Glycated albumin is a better indicator for glucose excursion than glycated hemoglobin in type 1 and type 2 diabetes. Endocr J 2008;55:503-7. [Crossref] [PubMed]
- Rondeau P, Bourdon E. The glycation of albumin: structural and functional impacts. Biochimie 2011;93:645-58. [Crossref] [PubMed]
- Chen S, Cohen MP, Ziyadeh FN. Amadori-glycated albumin in diabetic nephropathy: pathophysiologic connections. Kidney Int Suppl 2000;77:S40-4. [Crossref] [PubMed]
- Hasan NA. Effects of trace elements on albumin and lipoprotein glycation in diabetic retinopathy. Saudi Med J 2009;30:1263-71. [PubMed]
- Neelofar K, Ahmad J. An overview of in vitro and in vivo glycation of albumin: a potential disease marker in diabetes mellitus. Glycoconj J 2017;34:575-84. [Crossref] [PubMed]
- Raghav A, Ahmad J. Glycated serum albumin: a potential disease marker and an intermediate index of diabetes control. Diabetes Metab Syndr 2014;8:245-51. [Crossref] [PubMed]
- Raghav A, Ahmad J. Glycated albumin in chronic kidney disease: Pathophysiologic connections. Diabetes Metab Syndr 2018;12:463-8. [Crossref] [PubMed]
- Rodiño-Janeiro BK, Paradela-Dobarro B, Raposeiras-Roubín S, et al. Glycated human serum albumin induces NF-κB activation and endothelial nitric oxide synthase uncoupling in human umbilical vein endothelial cells. J Diabetes Complications 2015;29:984-92. [Crossref] [PubMed]
- Gan T, Liao B, Xu G. The clinical usefulness of glycated albumin in patients with diabetes and chronic kidney disease: Progress and challenges. J Diabetes Complications 2018;32:876-84. [Crossref] [PubMed]
- Anguizola J, Matsuda R, Barnaby OS, et al. Review: Glycation of human serum albumin. Clin Chim Acta 2013;425:64-76. [Crossref] [PubMed]
- Selvin E, Rawlings AM, Grams M, et al. Fructosamine and glycated albumin for risk stratification and prediction of incident diabetes and microvascular complications: a prospective cohort analysis of the Atherosclerosis Risk in Communities (ARIC) study. Lancet Diabetes Endocrinol 2014;2:279-88. [Crossref] [PubMed]
- Mukai N, Ohara T, Hata J, et al. Alternative Measures of Hyperglycemia and Risk of Alzheimer's Disease in the Community: The Hisayama Study. J Clin Endocrinol Metab 2017;102:3002-10. [Crossref] [PubMed]
- Huang Y, Sun X, Liu G, et al. Glycosylated serum protein may improve our ability to predict endothelial and erectile dysfunction in nonorganic patients. J Sex Med 2011;8:840-50. [Crossref] [PubMed]
- Neuman RG, Hud E, Cohen MP. Glycated albumin: a marker of glycaemic status in rats with experimental diabetes. Lab Anim 1994;28:63-9. [Crossref] [PubMed]
- Nirala BK, Perumal V, Gohil NK. Glycated serum albumin stimulates expression of endothelial cell specific molecule-1 in human umbilical vein endothelial cells: Implication in diabetes mediated endothelial dysfunction. Diab Vasc Dis Res 2015;12:290-7. [Crossref] [PubMed]
- Rodiño-Janeiro BK, González-Peteiro M, Ucieda-Somoza R, et al. Glycated albumin, a precursor of advanced glycation end-products, up-regulates NADPH oxidase and enhances oxidative stress in human endothelial cells: molecular correlate of diabetic vasculopathy. Diabetes Metab Res Rev 2010;26:550-8. [Crossref] [PubMed]
- Schalkwijk CG, Miyata T. Early- and advanced non-enzymatic glycation in diabetic vascular complications: the search for therapeutics. Amino Acids 2012;42:1193-204. [Crossref] [PubMed]
- Dahrouj M, Desjardins DM, Liu Y, et al. Receptor mediated disruption of retinal pigment epithelium function in acute glycated-albumin exposure. Exp Eye Res 2015;137:50-6. [Crossref] [PubMed]
- Amore A, Cirina P, Conti G, et al. Amadori-configurated albumin induces nitric oxide-dependent apoptosis of endothelial cells: a possible mechanism of diabetic vasculopathy. Nephrol Dial Transplant 2004;19:53-60. [Crossref] [PubMed]
- Schulz R, Görge PM, Görbe A, et al. Connexin 43 is an emerging therapeutic target in ischemia/reperfusion injury, cardioprotection and neuroprotection. Pharmacol Ther 2015;153:90-106. [Crossref] [PubMed]
- Hood AR, Ai X, Pogwizd SM. Regulation of cardiac gap junctions by protein phosphatases. J Mol Cell Cardiol 2017;107:52-7. [Crossref] [PubMed]
- Solan JL, Lampe PD. Specific Cx43 phosphorylation events regulate gap junction turnover in vivo. FEBS Lett 2014;588:1423-9. [Crossref] [PubMed]