Lycopene alleviates lipopolysaccharide-induced testicular injury in rats by activating the PPAR signaling pathway to integrate lipid metabolism and the inflammatory response
Highlight box
Key findings
• This study has obvious advantages as part of a series of studies. Comprehensive laboratory variable detection verified the protective effect of lycopene (LYC) on testicular injury and provided a novel treatment strategy for the management of inflammatory infertility.
What is known and what is new?
• Lycopene is a strong antioxidant with various biological functions.
• The precise role of LYC in reversing the dysregulation of lipid metabolism caused by testicular inflammation remains unclear. Based on the lipidome and transcriptome data, we suggested that LYC controls the expression of crucial genes in signaling pathways linked to lipid metabolism. This regulatory function combats cytokine overproduction and lipid peroxidation caused by testicular inflammation.
What is the implication, and what should change now?
• This theory that LYC regulates testicular lipid stability provides new insights into its anti-inflammatory and anti-oxidative properties and its therapeutic potential. Further validating study is still needed.
Introduction
Nearly half of all infertile couples worldwide experience male infertility (1). Male fertility is declining due to a variety of circumstances, with testicular dysfunction caused by inflammation and infection being the most common cause (2). In addition, testicular and systemic infections and inflammation, such as sepsis and COVID-19, can impair the testicular function and reduce fertility (3,4).
Lipopolysaccharide (LPS) is the main pathogenic component of gram-negative bacteria, and has been shown to cause systemic and testicular inflammation. The efficacy of spermatogenesis and the preservation of an adult male’s functional reproductive system depends on proper testicular lipid metabolism, which controls testosterone synthesis. The fluidity of the sperm membrane, sperm motility, and sperm-ovum fusion are all directly impacted by the lipid components of the sperm membrane. LPS disrupts the lipid metabolism in the testes, causing a rise in saturated fatty acids, such as palmitic acid (PA) (5). PA works synergistically with LPS to promote the development of pro-inflammatory responses. Furthermore, PA is been shown to facilitate the dimerization and translocation of the Toll-like receptor 4/2 (TLR4/2) into lipid rafts in the plasma membrane, and further recruit and activate downstream signaling molecules there (6). LPS-induced inflammation causes disturbances in fatty acid metabolism that can cause mitochondrial dysfunction and lead to the production of excess reactive oxygen species (ROS) (7). ROS in turn plays a specific role in the induction of inflammation as a secondary messenger, possibly activating nuclear factor kappa B (NF-κB) and escalating the inflammatory response (8). Moreover, ROS-induced lipid peroxidation results in loss of sperm membrane integrity, increased permeability, and DNA structural damage (9).
The peroxisome proliferator-activated receptors (PPARs) are ligand-activated transcription factors belonging to the nuclear receptor family. PPARs not only bind to DNA and regulate gene expression, but also act as intracellular receptors to bind active lipid molecules. The role of PPARs in inflammation is particularly relevant to disorders of lipid metabolism, such as metabolic syndrome and atherosclerosis, which have potential inflammatory factors in the pathogenesis (10). Furthermore, the mechanisms of PPARs in inflammation are diverse. PPAR can regulate key genes, and these genes have inflammation regulatory effects. For example, PPAR-α upregulates IκB expression, there by blocking nuclear translocation and activation of the pro-inflammatory transcription factor NF-κB. Alternatively, PPARs also regulate inflammatory processes by altering lipid metabolism, such as the lipid regulatory mechanism in human dendritic cells (11).
Antibiotic therapy alone cannot prevent infection nor inflammation-related testicular dysfunction. The spermatogenic function is frequently lost or impaired, although the endocrine function can still exist when infection or damage is alleviated. Lycopene (LYC, C40H56) is a natural carotenoid with 11 conjugated double bonds. LYC, which is mainly found in red fruits and vegetables, cannot be synthesized by the human body and needs to be ingested from the daily diet (12). LYC is a powerful antioxidant with various biological functions, such as anti-inflammatory, anti-cancer, lowering blood lipids and protecting the liver. It has been demonstrated to reduce oxidative stress and inflammation caused by LPS in many organs, including the liver, kidney, and lungs (13-15), as well as having the potential to treat cancer (16,17). In addition, research has demonstrated that LYC supplementation can improve sperm parameters and indicators of oxidative stress in oligospermia infertile males (18), suggesting a potential benefit of LYC for fertility preservation. The molecular mechanisms underlying the protective effects of LYC are complex. LYC promotes the activation of Nrf2/HO-1 pathway and further inhibits NLRP3 inflammasome by enhancing autophagy in Kupffer cells, thereby alleviating hepatic ischemia-reperfusion injury (19). Moreover, recent studies have revealed that LYC controls lipid homeostasis, preventing lipid peroxidation and lowering LPS in the circulation to treat inflammatory diseases (20,21). LYC was found to attenuate LPS-induced epididymis injury in our previous study. And its regulatory mechanism is related to the changes of plasma lipidome (22). The present study evaluated the protective effects of LYC against impaired testicular function caused by LPS-induced systemic inflammation. Furthermore, the testicular transcriptome and lipidomics studies revealed the possible mechanisms of LYC, offering potential therapeutic targets for treating inflammatory testicular injury. We present the following article in accordance with the ARRIVE reporting checklist (available at https://tau.amegroups.com/article/view/10.21037/tau-22-864/rc).
Methods
Animals and experimental design
A total of 40 Sprague-Dawley (SD) male rats (6–8 weeks old) weight 260±20 g were obtained from Jinling Hospital and housed under a conventional 12-hour light/dark cycle, at a constant temperature of 24±2 ℃, and relative humidity of 60%±5%. All animals were given standard feeding conditions and free access to water. All animal experiments were approved by the Experimental Animal Ethics Committee of Jinling Hospital (No. 2022JLHSXJDWLS-0021) and in compliance with National Institutes of Health protocol to laboratory guidelines for the care and use of animals.
After adaptive feeding for 14 days, the rats were randomly divided into the following 4 groups (n=10 in each group) using an Excel spreadsheet [2010]: control, oil, LPS, and LYC. Rats in the control group received no treatment. On day 0, LPS (0.5 mg/mL at 1 mL/100 g body weight) was intraperitoneally injected into the LYC and LPS groups. On day 1, rats in the oil group were administered 1 mL/100 g olive oil intragastrically. The LYC group was administered 0.5 mg/mL LYC (dissolved in olive oil) intragastrically at 1 mL/100 g body weight (23). Multiple strategies were applied in this procedure, including daily gavage at a fixed time and the researcher operating simultaneously to minimize potential confounders. On day 28, there were 31 rats remaining (10 rats in the control group, 10 in the oil group, 5 in the LPS group, and 6 in the LYC group), with the other rats excluded due to midway death. Rats were anesthetized by intraperitoneal injection of chloral hydrate and the testes were harvested immediately. To maximize the objectivity of the presented study, a protocol was prepared before the study. And this protocol was uploaded to the Open Science Frame work (https://osf.io/89cd7/).
Reagents
LPS was purchased from Sigma-Aldrich, Co. (USA). LYC was obtained from Nanjing Yuanjian Biotechnology Co., Ltd. (Nanjing, China). Interleukin-1 alpha (IL-1α, Cat ELK1148), interleukin-1 beta (IL-1β, Cat ELK1272), tumor necrosis factor-alpha (TNF-α, Cat ELK1396), monocyte chemotactic pretein1 (MCP-1, Cat ELK5504), and interleukin 6 (IL-6, Cat ELK1158) kits were purchased from ELK (Wuhan) Biotechnology Co., Ltd. (Wuhan, China). Catalase (CAT, Cat A007-1), glutathione peroxidase (GSH-PX, Cat A005-1), superoxide dismutase (SOD, Cat A001-1), total antioxidant capacity (TAOC, Cat A015-1), and malondialdehyde (MDA, Cat A003-1) kits were purchased from Nanjing Jiancheng Bioengineering Institute (Nanjing, China).
Enzyme-linked immunosorbent assay (ELISA)
The levels of cytokine IL-1α, IL-1β, TNF-α, IL-6, and MCP-1 (24) in the testes were determined using ELISA kits according to the instructions of the manufacturer.
Assay for redox status in testes
The redox status variables in the testes were measured according to the kit instructions. GSH-PX and TAOC were measured using colorimetry (25,26). CAT, SOD, and MDA were measured by spectrophotometry (24).
Histological evaluation
The testes were separated, transected, and one of them was left for 24 hours fixed in a centrifuge tube containing 4% paraformaldehyde. Different absolute ethanol concentrations (80%, 90%, 95%, and 100%) were used to perform gradient dehydration. The wax blocks were divided into sections with a thickness of 4–7 µm following treatment with the transparency and embedding procedures. The sections were deparaffinized and hydrated before being submerged in a hematoxylin staining solution for 8 minutes and then rinsed with water to remove the staining solution. The slices were separated in 1% hydrochloric acid ethanol for 5 seconds, re-dipped in ammonia water, and then washed with running water. The slices were then macerated for 3 minutes in an eosin staining solution. Following dehydration and sealing, the slices were examined, and image data was gathered using an inverted microscope with 100 and 200 views.
Lipidomics and data processing
The testes were retrieved from the −80 ℃ freezer and warmed gradually. A sample of the testes (50 mg) was added to 1.5 mL of a chloroform/methanol (2/1, v/v) solution, vortexed for one minute, and ground for 90 seconds at 60 Hz. The samples were centrifuged at room temperature for 10 minutes at 3,000 rpm. The organic phase was transferred to a different tube and mixed with 400 mL of isopropanol/propanol (1/1, v/v) and 5 mL of internal standard 0.14 mg/mL LPC (12:0). The samples were centrifuged at 4 ℃ at 12,000 rpm for 10 minutes. The supernatant was then transferred into the sample bottle. Mass spectrometric detection was performed using Orbitrap mass spectrometer (Q Exactive Orbitrap, Thermo Fisher Scientific, USA) (27). The chromatographic column (ACQUITY UPLC BEH C18 column, 2.1×100 mm, 1.7 µm) was used for chromatographic separation, which was carried out at 40 ℃ with a flow rate of 0.3 mL/min. A solution in acetonitrile/water (6:4, v/v, 10mM ammonium formate) made up the mobile phase A, and mobile phase B was composed of isopropyl alcohol/acetonitrile (9:1, v/v, 10 mM ammonium formate). The injection volumes were 1 µL (positive ion mode) and 3 µL (negative ion mode), respectively, and the temperature was 4 ℃. The gradient elution process was then performed. Quality control samples were always inserted during the detection process to assess the system’s stability throughout the entire experiment. They are created by mixing equal amounts of the samples to be tested.
The original file obtained by mass spectrometry was imported into Lipid Search (Thermo) software for image processing and database search to obtain qualitative and quantitative results on lipids. Multivariate analysis was then performed on the lipids. The differences in lipids between several groups were assessed using principal component analysis (PCA) and orthogonal projections to latent structure-discriminant analysis (OPLS-DA). The Student’s t-test P value of less than 0.05 and the Variable Importance in the Projection (VIP) of the first principal component of the OPLS-DA model being more than 1 were the criteria for identifying differential lipids. Further metabolic pathway analysis (enrichment analysis and topological analysis) showed the crucial pathways with the highest connection with significantly different lipids based on the Kyoko Encyclopedia of Genes and Genomes (KEGG) pathway analysis database (http://www.kegg.jp/kegg/pathway.html).
RNA sequencing and bioinformatics analysis
Total RNA was extracted using the extraction kit (magnetic bead method) (Cat T02-096) in accordance with the manufacturer’s instructions. The extracted total RNA was subjected to quality control by Agilent 2100 Bioanalyzer (Agilent Technologies, Santa Clara, CA, USA). RNA Clean XP Kit (Cat A63987, Beckman Coulter, Inc. Kraemer Boulevard Brea, CA, USA) and RNase-free DNase Set (Cat 79254, QIAGEN, GmBH, Germany) were used to purify the total RNA. Total RNA was re-checked by NanoDrop ND-2000 spectrophotometer/Qubit2.0 and Agilent 2100 Bioanalyzer (Agilent Technologies). After qualification, the purified total RNA was subjected to the steps of mRNA isolation, fragmentation, first strand cDNA synthesis, second strand cDNA synthesis, end repair, 3' end A addition, linker ligation, enrichment and so on according to the experimental operation instructions to complete the construction of sequencing sample library. Qubit® 2.0 Fluorometer was used to detect the concentration of the constructed library, and Agilent4200 was used to detect the size of the library to complete the quality inspection. Illumina NovaSeq6000 sequencer was used in PE150 mode. The sequencing quantity of all samples met the contract requirements, and the sequencing results met the contract standards, which could be used for data analysis.
Seqtk was used to filter the raw reads obtained by sequencing, and the spliced mapping algorithm of Hisat2 (version 2.0.4) was used to perform genome mapping of the preprocessed reads. To make the gene expression levels comparable, we first used Stringtie (version: 1.3.0) to count the number of fragments per gene after the Hisat2 alignment and then normalized them by the TMM (trimmed mean of M values) method. Finally, the FPKM (Fragments Per Kilobase of exon model per Million mapped reads) value of each gene was calculated. EdgeR software was used to analyze the differentially expressed genes between samples, and the P value was obtained and corrected for multiple hypothesis testing. The threshold of the P value was determined by controlling the False Discovery Rate, and the q-value was obtained after the P value correction. Meanwhile, Fold-change (FC) was calculated based on the FPKM value. Differential genes were identified using q-value ≤0.05 and FC ≥2.
Statistical analysis
All data were analyzed using statistical software SPSS 26.0. Comparisons were made using analysis of variance (ANOVA). A P value <0.05 was considered statistically significant.
Results
LYC alleviates LPS-induced excess cytokines and oxidative stress in testes
LPS significantly increased the levels of pro-inflammatory cytokines IL-1α, IL-6, and TNF-α (all P<0.001) compared with the control rats. The levels of IL1β (P<0.05) and MCP-1 (P<0.01) were also significantly increased (Figure 1). The excessive production of these cytokines indicated that LPS induced a strong inflammatory response in the testes. However, the activity of antioxidant enzymes GSH-PX, SOD, and CAT (all P<0.001) decreased significantly in the LPS group. The level of the lipid peroxide product MDA (P<0.001) increased significantly, and the TAOC level (P<0.01) decreased, indicating that the testes was subjected to oxidative stress and the antioxidant defense ability decreased (Figure 2).
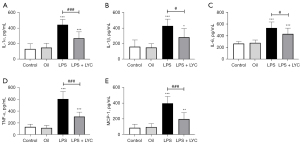
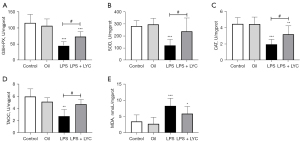
Compared with the LPS group, oral LYC significantly reduced IL-1α, TNF-α, and MCP-1 levels (all P<0.001). The levels of IL-1β (P<0.05) and IL-6 (P<0.05) were also significantly reduced (Figure 1). GSH-PX, SOD, CAT activity and TAOC levels (all P<0.05) were significantly elevated in the LYC group. However, there is no statistical difference in the MDA (P>0.05) levels between the two groups (Figure 2). These results suggested that LYC reduced testicular inflammation and oxidative stress induced by LPS and exerted a strong protective effect.
LYC improved the LPS-induced histological damage in the testes
Normal testis tissue was observed at 100 magnification in the control groups (Figure 3A,3B). In the LPS group, the interstitial space was broadening and obvious edema could be observed when compared with the control group (Figure 3C). The interstitial oedema was lessened after oral LYC treatment (Figure 3D). The details in normal seminiferous tubules can be observed at 200 magnification (Figure 3E,3F). In the LPS group, the seminiferous tubule epithelium was damaged or thinned. The spermatogenic cells were arranged disorderly, and the number was reduced (Figure 3G). In the LYC group, the seminiferous tubule epithelium was restored, and the spermatogenic lineage in the lumen was restored to its original order (Figure 3H).
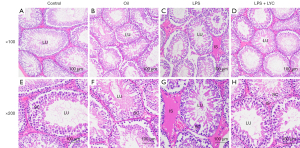
Lipidomic analysis of LYC-treated rats with LPS-induced testicular injury
A total of 1377 lipids were identified, including phosphatidylcholine (PC, 154), phosphatidylethanolamine (PE, 196), lysophosphatidylcholine (LPC, 73), phosphatidylglycerol (PG, 54), phosphatidylinositol (PI, 23), phosphatidylserine (PS, 34), ceramides (Cer, 38), sphingosine (SPH, 35), triglyceride (TG, 175), diglyceride (DG, 90) and others. These mainly fall under the categories of phospholipids, sphingolipids, neutral lipids, and other lipids. Multivariate analysis was then conducted to screen for lipids with significance. The PCA (Figure 4A,4B) and OPLS-DA (Figure 4C,4D) revealed a trend toward a substantial separation between the LYC and LPS groups, demonstrating that LYC therapy had a considerable impact on the lipid metabolism of rat testes. The permutation test (Figure 4E,4F) was used to validate the models. In the positive ion mode, the R2 and Q2 intercept values were 0.92 and 0.53, respectively, after 200 permutations. The low values of the Q2 intercept indicated the robustness of the models, and thus showed a low risk of over fitting and reliability. VIP >1 and P<0.05 were used as screening criteria, and 258 lipids were identified from the 1,377 lipids to be significantly changed.
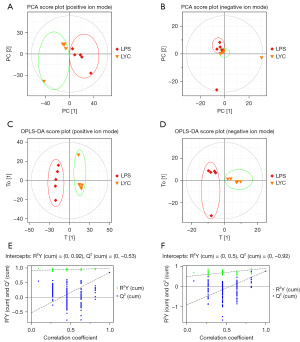
Bubble plots were drawn of the significantly different lipids (Figure 5A) to visualize individual lipids modulated by LYC intervention. These findings suggested that LYC significantly altered the lipid level and composition of the rat testes. The significant enrichment of several major metabolic pathways, such as glycerophospholipid metabolism, was revealed based on the KEGG database (Figure 5B). Glycerophospholipid metabolism is a primary cell membrane component that regulates terminal fluidity, kinetics, and homeostasis. Different lipids were dramatically altered by LYC, with PCs and PEs increasing while LPCs, PGs, and DGs decreased (Figure 5C). These pathways relating to fatty acid elongation, and fatty acid degradation and metabolism were extensively altered in LYC treatment of LPS-induced testicular injury (Figure 5C).
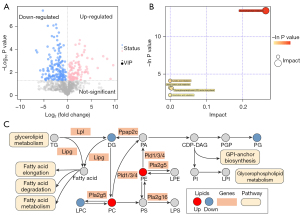
LYC changed gene expression and signaling pathways related lipid and fatty acid metabolism in the testes
Following LYC therapy, we identified 1,116 genes with differential expression, of which 1,043 were upregulated and 73 were downregulated (Figure 6A). The KEGG pathway enrichment analysis of differentially expressed genes revealed that LYC impacted several lipid metabolism-related pathways. The PPAR signaling pathway, the JAK-STAT signaling pathway, fatty acid metabolism, fatty acid breakdown, and fatty acid chain elongation were included (Figure 6B). RNA sequencing analysis found that the PPAR signaling pathways related genes, FABP3, LPL, Acsbg1, Hmgcs2, CD36, OLR1, CPT1A, PLTP, CYP27A1, and RXRα, had increased expression (Figure 6C).
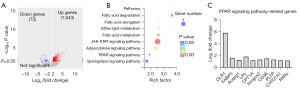
Upregulated RXRα gene expression in the PPAR pathway activates the PPAR/RXR dimer, stimulating downstream genes, such as FABP3, LPL, Acsbg1, CD36, and OLR1, which control fatty acid metabolism. CPT1A activation controls fatty acid oxidation. PLTP is activated to control lipid transport, and CYP27A1 expression was increased to control the metabolism of cholesterol (Figure 7). LYC intervention may increase the expression of genes essential for the PPAR signaling pathway. Additionally, by promoting the expression of PPAR signaling pathway downstream genes, the metabolic pathways for fatty acid metabolism, fatty acid degradation, and fatty acid elongation are controlled, partially explaining the changes in lipid composition and content (Figure 8).
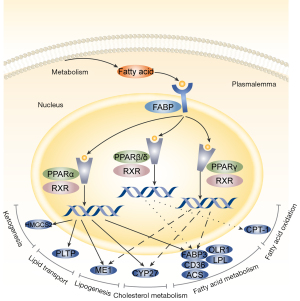
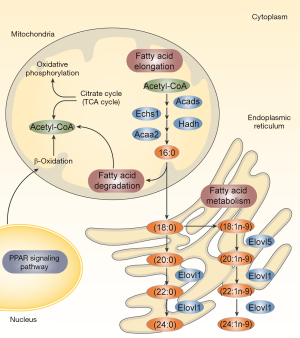
Discussion
This study conducted transcriptomes, lipidomics, and related investigations to identify the LYC-induced protective effects and underlying mechanisms against LPS-induced testicular injury. In the testes, LPS dramatically elevated the levels of cytokines IL-1α, IL-1β, TNF-α, MCP-1, and IL-6, suggesting that it causes intensive inflammation. Meanwhile, the high levels of MDA indicated lipid peroxidation injury, and the low activities of antioxidant enzymes, including GSH-PX, SOD, and CAT, showed that the antioxidant defenses decreased. However, LPS-induced testicular damage was considerably reversed by oral LYC therapy. Studies on the transcriptome and lipidome were conducted to confirm the aforementioned protective benefits. According to research, LYC therapy improves lipid homeostasis in LPS-induced testicular damage by changing the composition and quantity of lipids and lipid metabolism pathways. For example, increased PC content may reduce the overproduction of pro-inflammatory cytokines that LPS causes in the testes, in line with previous observations. PC may considerably lower the levels of TNF-α and IL-6 and decrease neutrophil infiltration (28), indicating that PC exerts anti-inflammatory activity in LPS-induced testicular immunological attack, which is suggestive of the ability of PC to reduce inflammation. In addition, it has been demonstrated that PC possesses antioxidant activity, and exogenous PC supplementation can raise antioxidant levels (29), which may help to protect sperm motility. The content of PC in seminal plasma is positively correlated with GPX and SOD activity, and highly active sperm antioxidant enzymes can delay lipid peroxidation and loss of motility (30). LPC, a lipid peroxidation product of PC, was significantly reduced after LYC treatment in this study, and it was negatively correlated with the activities of antioxidant enzymes in seminal plasma (21). Therefore, LYC can play an anti-inflammatory and anti-oxidative role in improving testicular injury by regulating testicular lipids. Transcriptome investigations were conducted to examine the underlying molecular mechanisms of LYC in LPS-induced testicular damage. The PPAR pathway was significantly impacted, as revealed by the KEGG enrichment study, along with several signaling pathways relevant to lipid and fatty acid metabolism.
Nuclear receptors, also referred to as PPAR, are lipid-activated transcription factors that control the transcriptional expression of target genes. They have emerged as important regulators of lipid metabolism and inflammation (31). There are three members of the PPAR family in the testes: PPARα, PPARβ/δ, and PPARγ. In somatic and germ cells, all PPARs are expressed (32). PPARα and PPARγ interact with the signaling pathway of the G protein-coupled estrogen receptor to regulate steroidogenesis by controlling lipid homeostasis (33). PPARs regulate fatty acid oxidation in Sertoli cells to support germ cell development and meet the energy requirements (34). Suppressing the expression of genes involved in inflammation is another essential role of PPARs. In numerous model systems, PPARs operate as signal-specific repressors of the transcription factors NF-κB, nuclear factor of activated T cells (NFAT), activator protein 1 (AP1), and signal transducer and activator of transcription (STATs) (35). PPAR transcriptional activity interferes with estrogen and androgen receptor expression and impairs testis receptor production and ROS metabolism LPS-induced inflammation (36). The PPAR nuclear receptor family has been shown to have a substantial association with lipid metabolism and inflammation (37).
This study demonstrated that LYC stimulated the PPAR signaling pathway in rats with LPS-induced testicular damage, activating the retinoic acid receptor rather than the PPAR receptor family members directly. Nuclear receptors govern transcription, and retinoic acid receptors are vital signaling nodes in this process. With PPAR nuclear receptors, they readily form dimers and are crucial in the development of inflammation and metabolic problems (38). This study revealed an increase in RXRα gene expression. RXRα is strongly expressed in macrophages in both rats and humans. The RXRα dimer regulates the gene transcription of macrophages, which controls inflammation, immunological responses, the clearance of apoptotic cells, and white blood cell movement. It may be a barrier to disorders associated with macrophage activity and a possible therapeutic target (39). Moreover, RXR/PPAR dimers are crucial for preserving cellular lipid homeostasis, controlling lipid uptake and storage in lipid droplets, β-oxidation, and cholesterol export. Since LYC treatment exhibits anticancer qualities linked to RXR activation (40) and excellent therapeutic promise in male-specific prostate cancer (41), it is predicted that the modulatory effects of LYC is produced through the RXR-mediated signaling pathway. However, no connection between LYC metabolism and the retinoic acid receptor activation pathway has been identified thus far (42). This study demonstrated that LYC activation of the RXR-mediated pathway reduced LPS-induced testicular damage.
The PPAR signaling pathway controls lipid transport, fatty acid transport, oxidation, degradation, and glycerophosphate metabolism by activating downstream genes. It partially explained the variations in the quantity and make-up of various lipid types in this study. In addition, we concentrated on several crucial downstream genes as prospective therapeutic targets. In the male reproductive system, fatty acid binding protein 3 (FABP3) is primarily found in the testicular Leydig cells, where it grows eight times from the first spermatogenic peak to adulthood (43). FABP3 is controlled by RXR/PPAR dimerization and predominantly influences fatty acid transport, specifically with a higher affinity for arachidonic acid than other fatty acids like PA (43,44). Arachidonic acid and its byproducts are crucial for controlling inflammation. In the current investigation, arachidonic acid metabolism was also shown to be significantly regulated in the testes homogenate lipidome by LYC treatment. Alternately, arachidonic acid is specifically involved in the movement of cholesterol mitochondria between the outer and inner membranes in Leydig cells, which is the first step in steroidogenesis. As a result, it has been hypothesized that FABP3 may contribute to testosterone synthesis by moving arachidonic acid (33). In response to LYC intervention, FABP3 expression was upregulated, indicating that it has a dual function in controlling inflammation and sperm in LPS-induced testicular damage. We also noticed that carnitine palmitoyltransferase 1A (CPT1A), a crucial enzyme involved in fatty acid oxidation, was upregulated. According to the findings, RXR/PPAR inhibits the ability of PA to cause lipid buildup via encouraging CPT1A-mediated fatty acid oxidation (34). This study has obvious advantages as part of a series of studies. Comprehensive laboratory variable detection verified the protective effect of LYC on testicular injury and provided a new treatment strategy for the basic treatment of inflammatory infertility. Meanwhile, this theory that LYC regulates testicular lipid stability provides new insights into its anti-inflammatory and anti-oxidative stress effects and may represent potential therapeutic targets. Nevertheless, there are still shortcomings in this study. The key signaling pathways and genes screened in this study should be verified in future experiments.
Conclusions
LYC enhanced RXR/PPAR expression activity, activated the PPAR signaling pathway, and integrated testicular lipid metabolism and inflammatory responses, which may partially explain the therapeutic mechanism of LYC in reducing cytokines and improving oxidative stress, thereby protecting male fertility. LYC may be an alternative supplement for treating male infertility associated with inflammation of the reproductive system. This study provides novel insights and potential therapeutic targets for LYC therapy in male infertility. Additionally, this study concentrated on the downstream PPAR signaling pathway genes, such for FABP3 and CPT1A, which may form the basis for further research.
Acknowledgments
Funding: This work was supported by the National Natural Science Foundation of China (No. 81771646), the Jiangsu Province Social and Development Project (No. BE2017724), and the Special Project of Family Planning in Military (No. 17JS013).
Footnote
Reporting Checklist: The authors have completed the ARRIVE reporting checklist. Available at https://tau.amegroups.com/article/view/10.21037/tau-22-864/rc
Data Sharing Statement: Available at https://tau.amegroups.com/article/view/10.21037/tau-22-864/dss
Peer Review File: Available at https://tau.amegroups.com/article/view/10.21037/tau-22-864/prf
Conflicts of Interest: All authors have completed the ICMJE uniform disclosure form (available at https://tau.amegroups.com/article/view/10.21037/tau-22-864/coif). The authors have no conflicts of interest to declare.
Ethical Statement: The authors are accountable for all aspects of the work in ensuring that questions related to the accuracy or integrity of any part of the work are appropriately investigated and resolved. Animal experiments were performed under a project license (No. 2022JLHSXJDWLS-0021) granted by the Experimental Animal Ethics Committee of Jinling Hospital, in compliance with National Institutes of Health protocol to laboratory guidelines for the care and use of animals.
Open Access Statement: This is an Open Access article distributed in accordance with the Creative Commons Attribution-NonCommercial-NoDerivs 4.0 International License (CC BY-NC-ND 4.0), which permits the non-commercial replication and distribution of the article with the strict proviso that no changes or edits are made and the original work is properly cited (including links to both the formal publication through the relevant DOI and the license). See: https://creativecommons.org/licenses/by-nc-nd/4.0/.
References
- Choy JT, Eisenberg ML. Male infertility as a window to health. Fertil Steril 2018;110:810-4. [Crossref] [PubMed]
- Sharma A, Minhas S, Dhillo WS, et al. Male infertility due to testicular disorders. J Clin Endocrinol Metab 2021;106:e442-59. [Crossref] [PubMed]
- Chen L, Wu X, Xu Y, et al. Modelling the dynamics of Trypanosoma rangeli and triatomine bug with logistic growth of vector and systemic transmission. Math Biosci Eng 2022;19:8452-78. [Crossref] [PubMed]
- Wang F, Liu W, Jiang Q, et al. Lipopolysaccharide-induced testicular dysfunction and epididymitis in mice: a critical role of tumor necrosis factor alpha. Biol Reprod 2019;100:849-61. [Crossref] [PubMed]
- Liu T, Tang X, Cui Y, et al. Fibroblast Growth Factor 19 Improves LPS-Induced Lipid Disorder and Organ Injury by Regulating Metabolomic Characteristics in Mice. Oxid Med Cell Longev 2022;2022:9673512. [Crossref] [PubMed]
- Hwang DH, Kim JA, Lee JY. Mechanisms for the activation of Toll-like receptor 2/4 by saturated fatty acids and inhibition by docosahexaenoic acid. Eur J Pharmacol 2016;785:24-35. [Crossref] [PubMed]
- Schönfeld P, Wojtczak L. Fatty acids as modulators of the cellular production of reactive oxygen species. Free Radic Biol Med 2008;45:231-41. [Crossref] [PubMed]
- Korbecki J, Bajdak-Rusinek K. The effect of palmitic acid on inflammatory response in macrophages: an overview of molecular mechanisms. Inflamm Res 2019;68:915-32. [Crossref] [PubMed]
- Hamilton LE, Oko R, Miranda-Vizuete A, et al. Sperm Redox System Equilibrium: Implications for Fertilization and Male Fertility. Adv Exp Med Biol 2022;1358:345-67. [Crossref] [PubMed]
- Varga T, Czimmerer Z, Nagy L. PPARs are a unique set of fatty acid regulated transcription factors controlling both lipid metabolism and inflammation. Biochim Biophys Acta 2011;1812:1007-22. [Crossref] [PubMed]
- Wahli W, Michalik L. PPARs at the crossroads of lipid signaling and inflammation. Trends Endocrinol Metab 2012;23:351-63. [Crossref] [PubMed]
- Imran M, Ghorat F, Ul-Haq I, et al. Lycopene as a Natural Antioxidant Used to Prevent Human Health Disorders. Antioxidants (Basel) 2020;9:706. [Crossref] [PubMed]
- Dong J, Li W, Cheng LM, et al. Lycopene attenuates LPS-induced liver injury by inactivation of NF-κB/COX-2 signaling. Int J Clin Exp Pathol 2019;12:817-25. [PubMed]
- Salari S, Ghorbanpour A, Marefati N, et al. Therapeutic effect of lycopene in lipopolysaccharide nephrotoxicity through alleviation of mitochondrial dysfunction, inflammation, and oxidative stress. Mol Biol Rep 2022;49:8429-38. [Crossref] [PubMed]
- Li WW, Wang TY, Cao B, et al. Synergistic protection of matrine and lycopene against lipopolysaccharide-induced acute lung injury in mice. Mol Med Rep 2019;20:455-62. [Crossref] [PubMed]
- Beynon RA, Richmond RC, Santos Ferreira DL, et al. Investigating the effects of lycopene and green tea on the metabolome of men at risk of prostate cancer: The ProDiet randomised controlled trial. Int J Cancer 2019;144:1918-28. [Crossref] [PubMed]
- Peng C, Zeleznik OA, Shutta KH, et al. A Metabolomics Analysis of Circulating Carotenoids and Breast Cancer Risk. Cancer Epidemiol Biomarkers Prev 2022;31:85-96. [Crossref] [PubMed]
- Nouri M, Amani R, Nasr-Esfahani M, et al. The effects of lycopene supplement on the spermatogram and seminal oxidative stress in infertile men: A randomized, double-blind, placebo-controlled clinical trial. Phytother Res 2019;33:3203-11. [Crossref] [PubMed]
- Xue R, Qiu J, Wei S, et al. Lycopene alleviates hepatic ischemia reperfusion injury via the Nrf2/HO-1 pathway mediated NLRP3 inflammasome inhibition in Kupffer cells. Ann Transl Med 2021;9:631. [Crossref] [PubMed]
- Kawata A, Murakami Y, Suzuki S, et al. Anti-inflammatory Activity of β-Carotene, Lycopene and Tri-n-butylborane, a Scavenger of Reactive Oxygen Species. In Vivo 2018;32:255-64. [PubMed]
- Wang J, Wang Z, Li B, et al. Lycopene attenuates western-diet-induced cognitive deficits via improving glycolipid metabolism dysfunction and inflammatory responses in gut-liver-brain axis. Int J Obes (Lond) 2019;43:1735-46. [Crossref] [PubMed]
- Li Y, Zhu J, Zhao X, et al. Oral Lycopene Administration Attenuates Inflammation and Oxidative Stress by Regulating Plasma Lipids in Rats with Lipopolysaccharide-Induced Epididymitis. J Inflamm Res 2022;15:6517-31. [Crossref] [PubMed]
- Gupta P, Bansal MP, Koul A. Lycopene modulates initiation of N-nitrosodiethylamine induced hepatocarcinogenesis: studies on chromosomal abnormalities, membrane fluidity and antioxidant defense system. Chem Biol Interact 2013;206:364-74. [Crossref] [PubMed]
- Chyra-Jach D, Kaletka Z, Dobrakowski M, et al. The Associations between Infertility and Antioxidants, Proinflammatory Cytokines, and Chemokines. Oxid Med Cell Longev 2018;2018:8354747. [Crossref] [PubMed]
- Long SH, Yu ZQ, Shuai L, et al. The hypoglycemic effect of the kelp on diabetes mellitus model induced by alloxan in rats. Int J Mol Sci 2012;13:3354-65. [Crossref] [PubMed]
- Mahfouz R, Sharma R, Sharma D, et al. Diagnostic value of the total antioxidant capacity (TAC) in human seminal plasma. Fertil Steril 2009;91:805-11. [Crossref] [PubMed]
- Wang J, Christison TT, Misuno K, et al. Metabolomic profiling of anionic metabolites in head and neck cancer cells by capillary ion chromatography with Orbitrap mass spectrometry. Anal Chem 2014;86:5116-24. [Crossref] [PubMed]
- Jung YY, Nam Y, Park YS, et al. Protective effect of phosphatidylcholine on lipopolysaccharide-induced acute inflammation in multiple organ injury. Korean J Physiol Pharmacol 2013;17:209-16. [Crossref] [PubMed]
- Khafaga AF. Exogenous phosphatidylcholine supplementation retrieve aluminum-induced toxicity in male albino rats. Environ Sci Pollut Res Int 2017;24:15589-98. [Crossref] [PubMed]
- Tavilani H, Goodarzi MT, Doosti M, et al. Relationship between seminal antioxidant enzymes and the phospholipid and fatty acid composition of spermatozoa. Reprod Biomed Online 2008;16:649-56. [Crossref] [PubMed]
- Bensinger SJ, Tontonoz P. Integration of metabolism and inflammation by lipid-activated nuclear receptors. Nature 2008;454:470-7. [Crossref] [PubMed]
- Froment P, Gizard F, Defever D, et al. Peroxisome proliferator-activated receptors in reproductive tissues: from gametogenesis to parturition. J Endocrinol 2006;189:199-209. [Crossref] [PubMed]
- Gorowska-Wojtowicz E, Dutka P, Kudrycka M, et al. Regulation of steroidogenic function of mouse Leydig cells: G-coupled membrane estrogen receptor and peroxisome proliferator-activated receptor partnership. J Physiol Pharmacol 2018; [Crossref] [PubMed]
- Monrose M, Thirouard L, Garcia M, et al. New perspectives on PPAR, VDR and FXRα as new actors in testicular pathophysiology. Mol Aspects Med 2021;78:100886. [Crossref] [PubMed]
- Glass CK, Ogawa S. Combinatorial roles of nuclear receptors in inflammation and immunity. Nat Rev Immunol 2006;6:44-55. [Crossref] [PubMed]
- Wang G, Cheng S, Zhang S, et al. LPS impairs steroidogenesis and ROS metabolism and induces PPAR transcriptional activity to disturb estrogen/androgen receptor expression in testicular cells. Mol Biol Rep 2020;47:1045-56. [Crossref] [PubMed]
- Chinetti G, Fruchart JC, Staels B. Peroxisome proliferator-activated receptors (PPARs): nuclear receptors at the crossroads between lipid metabolism and inflammation. Inflamm Res 2000;49:497-505. [Crossref] [PubMed]
- Sharma S, Shen T, Chitranshi N, et al. Retinoid X Receptor: Cellular and Biochemical Roles of Nuclear Receptor with a Focus on Neuropathological Involvement. Mol Neurobiol 2022;59:2027-50. [Crossref] [PubMed]
- Röszer T. Transcriptional control of apoptotic cell clearance by macrophage nuclear receptors. Apoptosis 2017;22:284-94. [Crossref] [PubMed]
- Aydemir G, Kasiri Y, Birta E, et al. Lycopene-derived bioactive retinoic acid receptors/retinoid-X receptors-activating metabolites may be relevant for lycopene's anti-cancer potential. Mol Nutr Food Res 2013;57:739-47. [Crossref] [PubMed]
- Marzocco S, Singla RK, Capasso A. Multifaceted Effects of Lycopene: A Boulevard to the Multitarget-Based Treatment for Cancer. Molecules 2021;26:5333. [Crossref] [PubMed]
- Caris-Veyrat C, Garcia AL, Reynaud E, et al. A Review About Lycopene-Induced Nuclear Hormone Receptor Signalling in Inflammation and Lipid Metabolism via still Unknown Endogenous Apo-10´-Lycopenoids. Int J Vitam Nutr Res 2016;86:62-70. [Crossref] [PubMed]
- Oresti GM, García-López J, Aveldaño MI, et al. Cell-type-specific regulation of genes involved in testicular lipid metabolism: fatty acid-binding proteins, diacylglycerol acyltransferases, and perilipin 2. Reproduction 2013;146:471-80. [Crossref] [PubMed]
- Murphy EJ, Owada Y, Kitanaka N, et al. Brain arachidonic acid incorporation is decreased in heart fatty acid binding protein gene-ablated mice. Biochemistry 2005;44:6350-60. [Crossref] [PubMed]
(English Language Editor: J. Teoh)