A clinical study of using ROC to compare the efficiency of ASL and BOLD in diagnosis of renal allograft function
Highlight box
Key findings
• The non-invasive assessment of arterial spin labeling (ASL) for clinical kidney transplant function is a more promising imaging technique than blood oxygen level-dependent (BOLD).
What is known and what is new?
• ASL–magnetic resonance imaging (MRI) is a functional MRI technique that can obtain perfusion information without injecting a contrast agent, which is unsuitable for post-transplant patients due to the effect of contrast agents on renal function in some cases.
• The microscopic oxygen metabolism and blood flow changes in the transplanted kidney can be observed during the follow-up period. The correlations can be used to explain the postoperative pathophysiological changes in the transplanted kidney.
What is the implication, and what should change now?
• Renal blood flow (RBF) values alone exhibit similar diagnostic performance to combined RBF and effective transverse relaxation rate values. Compared to BOLD, ASL is a more promising imaging technique for the non-invasive detection of renal allograft function.
Introduction
Following the first successful kidney transplantation in China in the 1960s, kidney transplantation has become a well-established method for the end-stage treatment of patients with chronic renal failure and uremia (1). Recently, the development of immunology, the use of immunosuppressants, and the improvement of surgical protocols have significantly improved the survival and quality of life of kidney transplant patients (2). Kidney transplantation in China has now entered a new phase, and under the current laws and regulations in China, donor organ donation after the death of a citizen and living organ donation by relatives have become the main sources of kidney transplantation organs.
The current clinical follow-up methods include serum creatinine (SCr) measurement, imaging tests, and transplant biopsy. Blood oxygen level-dependent (BOLD) magnetic resonance imaging (MRI) was first applied to the study of transplanted kidneys in 2005 and has undergone several changes in technical parameters and measurement indexes since this time. The imaging method is based on changes in the distribution of blood oxygen levels within the tissue, and it is the only method in current clinical studies that can assess the distribution of blood oxygen levels in living transplanted kidneys under non-invasive conditions, thus reflecting their internal oxygenation status (3,4). The hemoglobin in normal blood is divided into deoxyhemoglobin and oxygenated hemoglobin, which have opposite magnetic properties, the former being paramagnetic and the latter being inverse magnetic, and this property is used to cause a magnetic field gradient change in the water molecules around and inside the blood vessel. The signal generated by the magnetic resonance gradient echo sequence is detected by a single exponential curve-fitting equation for T2* at different TE (Echo time) times. The effective transverse relaxation rate (R2*) value indirectly reflects the microscopic changes of oxygen distribution in the transplanted kidney parenchyma, and the R2* value is proportional to the deoxygenated hemoglobin content.
In the early application of renal transplantation, the BOLD technique has been shown to have good application value in comparing different types of post-transplantation patients, and the R2* values of patients in the acute rejection (AR) group have been shown to be significantly lower than those of normal post-transplantation recipients (5,6). In the later study (7), scholars proposed corresponding differential diagnostic thresholds by calculation of the R2* value. In recent years, the BOLD technique has been used to understand postoperative changes in blood oxygen levels in donor-preserved kidneys and recipient kidneys after transplantation, and its feasibility in follow-up applications and its value in understanding the distribution and trends of blood oxygen after renal transplantation have been confirmed (8).
Arterial spin labeling (ASL) MRI is a functional MRI technique that can obtain perfusion information without injecting a contrast agent, which is unsuitable for post-transplant patients due to the effect of contrast agents on renal function in some cases; thus, ASL is uniquely suited to post-transplant applications. In post-transplantation applications, ASL has the unique advantage of being able to obtain the corresponding perfusion information by pre-labeling the water molecules in the arterial blood, acquiring both labeled and unlabeled images, and then calculating the signal changes based on the 2 sets of images. In previous studies, ASL has shown good potential and value in multiple applications in the kidney (9), including in its initial application to post-transplant perfusion analyses (10). One study compared different functional transplanted kidneys and found that the perfusion levels were significantly lower in insufficient kidneys (10). Further, several studies (11,12) have confirmed ASL reproducibility in post-transplant perfusion. Several studies (11,12) have confirmed its reproducibility and used quantitative analyses to establish differential diagnostic thresholds. Thus, ASL has been shown to provide a strong basis for the analysis of transplanted kidney perfusion in a non-invasive manner without the introduction of contrast agents. In previous studies (13,14), the ASL technique was used to longitudinally observe the pre- and post-transplant renal perfusion levels preoperatively (kidney transplant donors), 3 months, 1 year, and 2 years postoperatively (kidney transplant recipients). This study showed that the ASL technique can be used effectively in long-term post-transplantation follow-ups and to detect changes in perfusion in the transplanted kidney without invasion or contrast introduction.
The BOLD and ASL techniques can also provide information on morphology, blood oxygen levels, perfusion, and other aspects of the graft, and thus can be used in the early, non-invasive, and non-contrast detection of graft perfusion. The BOLD and ASL technologies provide richer and more sensitive information that can be used to detect changes in transplanted kidney function without the introduction of contrast agents. In addition, they can be used to observe the microscopic oxygen metabolism and blood flow changes in transplanted kidneys during the follow-up period. The correlations can be used to explain the postoperative pathophysiological changes in transplanted kidneys. We present the following article in accordance with the STARD reporting checklist (available at https://tau.amegroups.com/article/view/10.21037/tau-23-136/rc).
Methods
Participants
The study was conducted in accordance with the Declaration of Helsinki (as revised in 2013). The study was approved by ethics board of Jinling Hospital (No. 2019NZKYZY-009-01). All of the written informed consent was obtained from the participants or legal guardians in the study. From September 2019 to September 2021, the data of 169 serial kidney transplant recipients (with an indication for renal biopsy or an active request for renal biopsy) were collected from Jinling Hospital. All the subjects underwent ASL, BOLD-MRI, and a laboratory examination within 1 week before renal biopsy. After all the examinations, the subjects were transferred to the Nephrotic Center for Renal Transplantation Biopsy.
To be eligible for inclusion in this study, patients had to meet the following inclusion criteria: (I) be a renal transplant recipient with indications for renal biopsy; and (II) be able to cooperate and complete functional MRI. Patients were excluded from the study if they met any of the following exclusion criteria: (I) refused to undergo the relevant examination; (II) had a transplantation duration (renal transplantation completed to functional MRI examination) ≤1 month (patients with hyperacute rejection and accelerated rejection were excluded); (III) had obvious stenosis of the transplanted renal artery [stenosis ≥50% as revealed by non-contrast enhanced magnetic resonance angiography (MRA)]; (IV) had claustrophobia or other MRI contraindications; and/or (V) had poor quality MRI images.
Ultimately, 135 patients (103 males; average age: 38.59±11.03 years; range: 12–71 years) were included in the present study. Their SCr levels were obtained, and their estimated glomerular filtration rate (eGFR) (15) values were calculated on the day of the MRI. According to the eGFR values, patients were divided into the following 2 groups: (I) the normal renal allograft function group (eGFR ≥60 mL/min/1.73 m2; n=42; 34 males and 8 females; aged: 35.69±10.06 years, age range: 12–56 years); and (II) the renal allograft injury group (eGFR <60 mL/min/1.73 m2; n=93; 69 males and 24 females; age: 39.89±11.26 years; age range: 18–71 years). The threshold of 60 mL/min/1.73 m2 was selected because chronic kidney disease (CKD) patients who require kidney transplantation are identified by GFR values below this threshold.
MRI protocol and image analysis
The MRI instrument used was the 3.0T MRI system (Discovery MR750; GE Healthcare, Milwaukee, WI, USA). The patients were asked to fast at least 6 hours before MRI testing. Two abdominal radiologists (PJ and LS) with >10 years of experience in MRI analysis interpreted the ASL and BOLD images. The radiologists were blinded to the patient data.
ASL perfusion images were obtained using a 3-dimensional pseudo-continuous ASL (pCASL) sequence with spiral fast spin echo (FSE) readout. Each spiral arm included 512 sampling points in k-space and a total of 8 interleaves (arms) were acquired separately. Selective inversion and saturation pulses were applied to a slab containing the imaged region and ending at the labeling plane, with a labeling duration of 1.5 s (inversion time) and a post-labeling delay (PLD) of 1.5 s (16). The acquisition parameters of the sequence were as follows: repetition time (TR), 4,629 ms; echo time (TE), 10.5 ms; flip angle, 111 degrees, bandwidth, 62.5 kHz; field of view (FOV), 34×34 cm2; spiral acquisition matrix, 512×8 arms; reconstruction matrix, 128×180; slice thickness/gap, 4/1 mm; number of excitations (NEX), 3; labeling duration, 1,500 ms; and post labeling delay, 1,500 ms. A separate proton density-weighted reference scan was obtained for perfusion quantification with acquisition parameters identical to pCASL but without labeling or background suppression pulses. The patients were instructed to breathe smoothly and shallowly during the scans, then retrospectively adjust for motion correction. The ASL tag and non-tag images were then transferred to the Advantage 4.6 Workstation (GE Healthcare). ASL perfusion maps calculated from the tag and control non-tag pairs were averaged for each subject to form an individual whole kidney perfusion-weighted map. Quantitative renal blood flow maps were calculated based on a two-compartment ASL model, using the vendor-provided toolbox. The labeling efficiency was 85%, the blood-tissue partition coefficient was 0.9 mL/g, and the blood longitudinal relaxation time was 1,650 ms (16). On the proton density maps, 6–10 equal area circular regions of interest (ROIs) were manually placed in the cortex of the upper, middle, and lower poles of the renal allograft, and the mean RBF values was recorded. Regions with apparent susceptibility artifacts were avoided.
BOLD imaging was acquired using a 2-dimesnioanl multi-echo gradient recalled echo (GRE) sequence in the coronal plane during 11-second breath holds at the end-expiration using the following parameters: TR, 200 ms; TE, 2.1–32.8 ms; flip angle, 60 degrees; bandwidth, 62.5 kHz; FOV, 36×36 cm2; matrix, 128×128; slice thickness/gap, 6/1 mm; and NEX, 1. The R2* maps were generated using FuncTool software implemented in Advantage Workstation version 4.6 (GE Healthcare) by mono-exponential fitting. For each participant, 6–10 equal area circular regions of interest (ROIs) were manually placed in the medulla of the upper, middle, and lower poles of the renal allograft, and the mean R2* values was recorded. Regions with apparent susceptibility artifacts were avoided.
Statistical analysis
The data were analyzed by SPSS 25.0 (IBM Corp, Armonk, NY, USA). The metering data are presented as the mean ± standard deviation. The differences in the count data between the 2 groups were compared using the Chi-square test, and differences in age, SCr, eGFR, postoperative time, RBF, and R2* values between the 2 groups were compared using the Student’s t-test. Pearson’s method was used to analyze the relationship between RBF, R2* values, and eGFR. A receiver operating characteristic (ROC) curve the youden index analysis were performed to assess the diagnostic efficiency of RBF, R2* values, and their combination in distinguishing allografts with injury from those with normal function. A P value <0.05 was considered statistically significant.
Results
Clinical characteristics
As Table 1 and Figure 1 show, patients in the renal allograft injury group were older than those in the normally functioning allograft group, and those in the renal allograft injury group had a longer postoperative time, higher SCr levels, and lower eGFR levels. The mean RBF value was significantly lower in the renal allograft injury group than the normal group (104.33±54.76 mL/100 g/min versus 191.84±63.96 mL/100 g/min, respectively; P<0.0001), while the mean medullary R2* value was significantly higher in the renal allograft injury group than the normal group (27.91±3.35 versus 25.22±2.94 1/sec, respectively; P<0.0001.
Table 1
Characteristics | Normal renal allograft group (n=42) | Renal allograft injury group (n=93) | Total (n=135) | Statistic | P value |
---|---|---|---|---|---|
Gender, male | 34 (80.95) | 69 (74.19) | 103 (76.30) | 0.73 | 0.3926 |
Age (years) | 35.69±10.06 | 39.89±11.26 | 38.59±11.03 | –2.07 | 0.0401 |
Scr (μmol/L) | 98.15±22.66 | 252.62±139.81 | 204.56±136.86 | –10.36 | <0.0001 |
ASL (mL/100 g/min) | 191.84±63.96 | 104.33±54.76 | 131.55±70.45 | 8.15 | <0.0001 |
BOLD (sec-1) | 25.22±2.94 | 27.91±3.35 | 27.08±3.45 | –4.48 | <0.0001 |
eGFR (mL·min-1/1.73 m2) | 83.77±19.89 | 32.11±14.93 | 48.18±29.16 | 15.03 | <0.0001 |
Postoperative time (months) | 17.74±24.52 | 49.28±59.06 | 39.47±52.85 | –3.33 | 0.0011 |
Data are presented as the mean ± standard deviation or n (%). Postoperative time indicates time after kidney transplantation. BOLD value indicates medullary R2*. BOLD, blood oxygen level-dependent; ASL, arterial spin labeling; MRI, magnetic resonance imaging; Scr, serum creatinine; eGFR, estimated glomerular filtration rate.
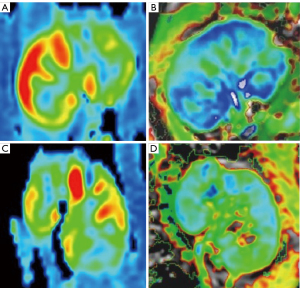
Relationship between ASL, BOLD and eGFR
As Figure 2 and Table 2 show, ASL was positively correlated to eGFR (r=0.7297, P<0.0001), while the R2* values were negatively correlated to eGFR (r=–0.4420, P<0.0001). Additionally, RBF was negatively correlated to the R2* values (r=–0.5392, P<0.0001).
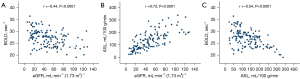
Table 2
Correlation | Correlation coefficient | P value |
---|---|---|
ASL and eGFR | 0.7297 | <0.0001 |
BOLD and eGFR | –0.4420 | <0.0001 |
ASL and BOLD | –0.5392 | <0.0001 |
ASL, arterial spin labeling; BOLD, blood oxygen level-dependent; eGFR, estimated glomerular filtration rate.
Diagnostic value of ASL and BOLD-MRI
As Table 3 and Figure 3 show, the AUC in discriminating the normal group and the injury group was higher for the RBF value (AUC =0.86) than the R2* value (AUC =0.72). The AUC value for the RBF and R2* combined diagnosis was higher than that for the RBF diagnosis alone (AUC =0.86, P=0.95). The BOLD parameters did not improve the diagnostic performance of renal injury function compared to ASL alone. However, the diagnostic performance of BOLD was enhanced when combined with ASL (AUC =0.86, P<0.01). As Table 4 show, the diagnostic accuracy of ASL was 80.00%, better than 71.85% of BOLD; the sensitivity and specificity of ASL in diagnosing renal allograft dysfunction were 79.57% and 80.95%, superior to 77.42% and 59.52% of BOLD.
Table 3
Diagnostic performance | AUC | 95% CI | χ2 | P value |
---|---|---|---|---|
ASL | 0.8632 | 0.8026, 0.9238 | 0.0035 | 0.9526 |
BOLD | 0.7163 | 0.6247, 0.8080 | 12.3814 | 0.0004 |
ASL + BOLD | 0.8635 | 0.8029, 0.9241 | Reference | Reference |
The χ2 statistic and P value were recalculated using the combination of ASL and BOLD as reference groups. ASL, arterial spin labeling; BOLD, blood oxygen level-dependent; AUC, area under the curve.
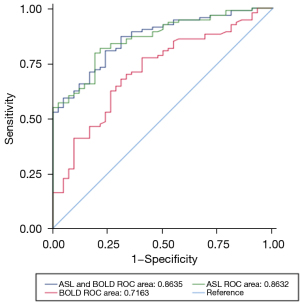
Table 4
Diagnostic performance | Statistical analysis (%) | ||||
---|---|---|---|---|---|
Sens. | Spec. | NPV | PPV | Acc. | |
ASL | 79.57 | 80.95 | 64.15 | 90.24 | 80.00 |
BOLD | 77.42 | 59.52 | 54.35 | 80.90 | 71.85 |
ASL, arterial spin labeling; BOLD, blood oxygen level-dependent; Sens., sensitivity; Spec., specificity; NPV, negative predictive value; PPV, positive predictive value; Acc., accuracy.
Discussion
The renal parenchyma consists of 2 parts; that is, the renal cortex and the renal medulla, of which the renal cortex is superficial and rich in blood flow distribution, and the renal medulla is located in the deeper layer, with significantly lower blood flow distribution than the renal cortex. The renal unit forms the basic unit of renal structure and function and participates in the completion of urinary functions in the human body together with the collecting ducts. The kidney is the most important organ in the urinary system for the excretion of metabolic products, glomerular filtration, tubular and collecting duct reabsorption, and maintenance of osmotic pressure levels. These physiological metabolic processes need oxygen consumption, and the renal medulla consumes the most oxygen. Research has shown that 20–25% of the total cardiac output blood passes through the kidneys, and the renal cortex accounts for >90% of the renal blood flow and the renal medulla for less; thus, the renal cortex is a high blood flow and high-oxygen content zone, while the renal medulla does the opposite (17). Early changes in oxygen content and perfused blood flow in renal tissues provide a theoretical basis for the application of functional MRI in the kidney.
Renal transplantation is the best treatment for patients with end-stage renal disease, and kidney transplantation from any source can benefit patients (18). Renal transplantation contributes to significant improvements in renal transplant recipients survival, which seems to be related to a decrease in acute rejection and the development of more effective immunosuppressive regimens and adequate care. However, with the loss of transplanted kidney function and the death of the transplanted kidney, only about 50% of the deceased donors and 70% of the transplanted kidneys of living donors are still functioning 10 years after transplantation (19).
Given the substantial morbidity associated with graft failure, the technology and timing for the detection of renal injury are key priorities for research on renal transplantation. SCR levels are widely used clinically to assess renal function, but their lack of specificity and sensitivity are unavoidable disadvantages. Severe kidney injury and loss of function may occur before significant changes in creatinine are detected in native kidney disease patients (20). Thus, when functional changes are detected, biopsies show more obvious and serious changes, often with limited reversibility and a lower chance of diminished kidney damage.
In response to hypoxia, a higher R2* value is correlated with a higher tissue content of deoxyhemoglobin with reduced tissue oxygenation and vice versa. When using BOLD imaging to assess kidney function, the following factors should be considered: (I) the renal medulla frequently shows a larger R2* value than the cortex, and the left kidney has a higher R2* value than the right kidney; (II) several transplantation-related factors should be examined, including hematocrit, circumstances that may alter the oxygen/hemoglobin dissociation curve, vascular volume fraction, and salt and water balance; and (III) it is essential to account for the use of diuretics, prostaglandin inhibitors, and angiotensin-converting enzyme inhibitors/angiotensin receptor blockers when measuring renal oxygenation.
In this study, a significant difference was observed between the normal renal allograft group and the renal allograft injury group in the medullary R2* value (25.22±2.94 versus 27.91±3.35 1/s, respectively; P<0.05), which suggests patients with injured renal function have increased medullary deoxyhemoglobin levels. Research has (21) reported higher R2* values in patients with acute rejection than in those without rejection. A previous study (22) showed that acute tubular necrosis (ATN) and AR patients had lower R2* values than functionally normal allograft patients. Another study (23) reported that the medullary R2* values were lower in transplanted kidneys than native kidneys, which may be due to increased blood flow due to allograft debulking.
ASL-MRI is a non-invasive method used to quantify renal blood flow without contrast and has high reproducibility for renal perfusion analysis. Factors that affect ASL include age, water content, intravenous injection, handgrip exercise results, and hip flexion (24). Consistent with the results of previous studies (10-12), we found a significant difference between the RBF values of the functionally normal allograft patients (191.84±63.96 mL/100 g/min) and the injured allograft patients (104.33±54.76 mL/100 g/min, P<0.05). This difference may indicate impaired cortical kidney perfusion, stemming from damaged capillaries and decreased renal filtration in damaged kidney allografts. A previous study (25) showed that cortical perfusion was lower in renal allograft recipients with low eGFR, while 2-4 weeks after transplantation.
Our ROC analysis showed that the R2* values could distinguish between injured allografts and normally functioning allografts (AUC =0.72, 95% CI: 0.62–0.81). A previous study (26) showed that R2* values in the medulla (AUC =0.98, P<0.01) can diagnose acute rejection, which is in line with our findings. Another study (27) reported that a cortical R2* value of 11.7 may predict early impaired graft function (defined as eGFR <40 mL/min/1.73 m2; AUC =0.71, P=0.02), but that R2* was not a significant predictor of renal function in the long-term follow-up period.
RBF could also distinguish between injured and normally functioning allografts (AUC =0.86, 95% CI: 0.80–0.92). A previous study (28) found that perfusion measured by ASL was predictive of disease if the eGFR of the transplanted kidney was >30 mL/min/1.73 m2 at 12 months (AUC =0.84). Studies (29,30) have found that the AUC for predicting renal allograft dysfunction (defined as eGFR <45 mL/min/1.73 m2) with ASL was 0.76 (95% CI: 0.65–0.88). In addition, the combination of ASL with reduced FOV in-vitro incoherent motion diffusion-weighted imaging did not significantly improve the performance of identifying renal dysfunction compared to the administration of ASL (P>0.05) (31,32).
Several studies (19,33) have investigated the function of renal allografts using ASL and other functional MRI techniques and have identified renal blood flow and regional renal perfusion as major determinants of oxygen delivery and thus oxygenation of renal tissues. BOLD imaging can detect hypoxic states but may be limited in distinguishing between increased oxygen consumption and decreased blood supply. A strong negative correlation between R2* and RBF was observed in the present study, which may explain the small diagnostic value added by the combination of R2* and RBF compared to RBF values alone. Previous studies (34,35) have evaluated renal function using ASL and BOLD, and reported an 8.3% reduction in the medullary R2* values of transplant patients during a 2-year follow-up period.
This study had a number of limitations. First, as a single-center study, the sample was small. Second, we did not collect kidney biopsy data. Third, this was a cross-sectional study, and other factors may affect graft function. Thus, a well-designed prospective study needs to be conducted to examine the diagnostic value of BOLD and ASL-MRI for renal allografts. Fourth, the method of averaging the 3 ROIs may have led to measurement error.
Conclusions
The findings of this study suggest a significant difference between the RBF and R2* values in patients with normal renal allografts and patients with injured renal allografts. Both RBF and R2* values can predict renal allograft function injury. RBF values alone exhibit similar diagnostic performance to combined RBF and R2* values. Compared to BOLD, ASL is a more promising imaging technique for the non-invasive detection of renal allograft function.
Acknowledgments
Funding: None.
Footnote
Reporting Checklist: The authors have completed the STARD reporting checklist. Available at https://tau.amegroups.com/article/view/10.21037/tau-23-136/rc
Data Sharing Statement: Available at https://tau.amegroups.com/article/view/10.21037/tau-23-136/dss
Peer Review File: Available at https://tau.amegroups.com/article/view/10.21037/tau-23-136/prf
Conflicts of Interest: All authors have completed the ICMJE uniform disclosure form (available at https://tau.amegroups.com/article/view/10.21037/tau-23-136/coif). The authors have no conflicts of interest to declare.
Ethical Statement: The authors are accountable for all aspects of the work in ensuring that questions related to the accuracy or integrity of any part of the work are appropriately investigated and resolved. The study was conducted in accordance with the Declaration of Helsinki (as revised in 2013). The study was approved by ethics board of Jinling Hospital (No. 2019NZKYZY-009-01). All of the written informed consent was obtained from the participants or legal guardians in the study.
Open Access Statement: This is an Open Access article distributed in accordance with the Creative Commons Attribution-NonCommercial-NoDerivs 4.0 International License (CC BY-NC-ND 4.0), which permits the non-commercial replication and distribution of the article with the strict proviso that no changes or edits are made and the original work is properly cited (including links to both the formal publication through the relevant DOI and the license). See: https://creativecommons.org/licenses/by-nc-nd/4.0/.
References
- Jagdale A, Cooper DK, Iwase H, et al. Chronic Dialysis in Patients with End-stage Renal Disease: Relevance to Kidney Xenotransplantation. Xenotransplantation 2019;26:e12471. [Crossref] [PubMed]
- Cantow K, Evans RG, Grosenick D, et al. Quantitative Assessment of Renal Perfusion and Oxygenation by Invasive Probes: Basic Concepts. Methods Mol Biol 2021;2216:89-107. [Crossref] [PubMed]
- Ku MC, Fernández-Seara MA, Kober F, et al. Noninvasive Renal Perfusion Measurement Using Arterial Spin Labeling (ASL) MRI: Basic Concept. Methods Mol Biol 2021;2216:229-39. [Crossref] [PubMed]
- Cai YZ, Li ZC, Zuo PL, et al. Diagnostic value of renal perfusion in patients with chronic kidney disease using 3D arterial spin labeling. J Magn Reson Imaging 2017;46:589-94. [Crossref] [PubMed]
- Ren T, Wen CL, Chen LH, et al. Evaluation of renal allografts function early after transplantation using intravoxel incoherent motion and arterial spin labeling MRI. Magn Reson Imaging 2016;34:908-14. [Crossref] [PubMed]
- Zhang JL, Lee VS. Renal perfusion imaging by MRI. J Magn Reson Imaging 2020;52:369-79. [Crossref] [PubMed]
- Yu YM, Ni QQ, Wang ZJ, et al. Multiparametric Functional Magnetic Resonance Imaging for Evaluating Renal Allograft Injury. Korean J Radiol 2019;20:894-908. [Crossref] [PubMed]
- Buchanan CE, Mahmoud H, Cox EF, et al. Quantitative assessment of renal structural and functional changes in chronic kidney disease using multi-parametric magnetic resonance imaging. Nephrol Dial Transplant 2020;35:955-64. [Crossref] [PubMed]
- Radovic T, Jankovic MM, Stevic R, et al. Detection of impaired renal allograft function in paediatric and young adult patients using arterial spin labelling MRI (ASL-MRI). Sci Rep 2022;12:828. [Crossref] [PubMed]
- OPTN/SRTR 2018 Annual Data Report: Introduction. Am J Transplant 2020;20:11-9. [Crossref] [PubMed]
- Langewisch E, Mannon RB. Chronic Allograft Injury. Clin J Am Soc Nephrol 2021;16:1723-9. [Crossref] [PubMed]
- Jiang K, Ferguson CM, Lerman LO. Noninvasive assessment of renal fibrosis by magnetic resonance imaging and ultrasound techniques. Transl Res 2019;209:105-20. [Crossref] [PubMed]
- Hall ME, Jordan JH, Juncos LA, et al. BOLD magnetic resonance imaging in nephrology. Int J Nephrol Renovasc Dis 2018;11:103-12. [Crossref] [PubMed]
- Shimizu K, Kosaka N, Fujiwara Y, et al. Arterial Transit Time-corrected Renal Blood Flow Measurement with Pulsed Continuous Arterial Spin Labeling MR Imaging. Magn Reson Med Sci 2017;16:38-44. [Crossref] [PubMed]
- Fiseha T, Mengesha T, Girma R, et al. Estimation of renal function in adult outpatients with normal serum creatinine. BMC Res Notes 2019;12:462. [Crossref] [PubMed]
- Nery F, Buchanan CE, Harteveld AA, et al. Consensus-based technical recommendations for clinical translation of renal ASL MRI. MAGMA 2020;33:141-61. [PubMed]
- Chowdhury AH, Cox EF, Francis ST, et al. A randomized, controlled, double-blind crossover study on the effects of 1-L infusions of 6% hydroxyethyl starch suspended in 0.9% saline (voluven) and a balanced solution (Plasma Volume Redibag) on blood volume, renal blood flow velocity, and renal cortical tissue perfusion in healthy volunteers. Ann Surg 2014;259:881-7. [Crossref] [PubMed]
- Haddock BT, Francis ST, Larsson HBW, et al. Assessment of Perfusion and Oxygenation of the Human Renal Cortex and Medulla by Quantitative MRI during Handgrip Exercise. J Am Soc Nephrol 2018;29:2510-7. [Crossref] [PubMed]
- Mani LY, Seif M, Nikles F, et al. Hip Position Acutely Affects Oxygenation and Perfusion of Kidney Grafts as Measured by Functional Magnetic Resonance Imaging Methods-The Bent Knee Study. Front Med (Lausanne) 2021;8:697055. [Crossref] [PubMed]
- Shehata M, Shalaby A, Switala AE, et al. A multimodal computer-aided diagnostic system for precise identification of renal allograft rejection: Preliminary results. Med Phys 2020;47:2427-40. [Crossref] [PubMed]
- Hanssen O, Erpicum P, Lovinfosse P, et al. Non-invasive approaches in the diagnosis of acute rejection in kidney transplant recipients. Clinical Kidney Journal 2017;10:97-105. [PubMed]
- Lal H, Mohamed E, Soni N, et al. Role of Blood Oxygen Level-dependent MRI in Differentiation of Acute Renal Allograft Dysfunction. Indian J Nephrol 2018;28:441-7. [Crossref] [PubMed]
- Pruijm M, Aslam I, Milani B, et al. Magnetic Resonance Imaging to Diagnose and Predict the Outcome of Diabetic Kidney Disease-Where Do We Stand? Kidney Dial 2022;2:407-18. [Crossref]
- Heusch P, Wittsack HJ, Blondin D, et al. Functional evaluation of transplanted kidneys using arterial spin labeling MRI. J Magn Reson Imaging 2014;40:84-9. [Crossref] [PubMed]
- Smith TB, Nicholson ML, Hosgood SA. Advances in Hypothermic and Normothermic Perfusion in Kidney Transplantation. Transplantology 2021;2:460-77. [Crossref]
- Rossi C, Artunc F, Martirosian P, et al. Histogram analysis of renal arterial spin labeling perfusion data reveals differences between volunteers and patients with mild chronic kidney disease. Invest Radiol 2012;47:490-6. [Crossref] [PubMed]
- Xiao W, Xu J, Wang Q, et al. Functional evaluation of transplanted kidneys in normal function and acute rejection using BOLD MR imaging. Eur J Radiol 2012;81:838-45. [Crossref] [PubMed]
- Seif M, Eisenberger U, Binser T, et al. Renal Blood Oxygenation Level-dependent Imaging in Longitudinal Follow-up of Donated and Remaining Kidneys. Radiology 2016;279:795-804. [Crossref] [PubMed]
- Vermathen P, Binser T, Boesch C, et al. Three-year follow-up of human transplanted kidneys by diffusion-weighted MRI and blood oxygenation level-dependent imaging. J Magn Reson Imaging 2012;35:1133-8. [Crossref] [PubMed]
- Hueper K, Gueler F, Bräsen JH, et al. Functional MRI detects perfusion impairment in renal allografts with delayed graft function. Am J Physiol Renal Physiol 2015;308:F1444-51. [Crossref] [PubMed]
- Yu YM, Wang W, Wen J, et al. Detection of renal allograft fibrosis with MRI: arterial spin labeling outperforms reduced field-of-view IVIM. Eur Radiol 2021;31:6696-707. [Crossref] [PubMed]
- Wang W, Yu Y, Wen J, et al. Combination of Functional Magnetic Resonance Imaging and Histopathologic Analysis to Evaluate Interstitial Fibrosis in Kidney Allografts. Clin J Am Soc Nephrol 2019;14:1372-80. [Crossref] [PubMed]
- Sławińska A, Serafin Z, Zawada E, et al. Noninvasive evaluation of renal tissue oxygenation with blood oxygen level-dependent magnetic resonance imaging early after transplantation has a limited predictive value for the delayed graft function. Pol J Radiol 2018;83:e389-93. [Crossref] [PubMed]
- Niles DJ, Artz NS, Djamali A, et al. Longitudinal Assessment of Renal Perfusion and Oxygenation in Transplant Donor-Recipient Pairs Using Arterial Spin Labeling and Blood Oxygen Level-Dependent Magnetic Resonance Imaging. Invest Radiol 2016;51:113-20. [Crossref] [PubMed]
- Hesp AC, Schaub JA, Prasad PV, et al. The role of renal hypoxia in the pathogenesis of diabetic kidney disease: a promising target for newer renoprotective agents including SGLT2 inhibitors? Kidney Int 2020;98:579-89. [Crossref] [PubMed]
(English Language Editor: L. Huleatt)