Cellular regenerative therapy in stress urinary incontinence: new frontiers?—a narrative review
Introduction
The pathophysiology of female stress urinary incontinence (SUI) is multifactorial. It may involve loss of urethral sphincter muscle function, but often decreased support of the pelvic floor and vaginal connective tissue around the bladder neck and urethra can be important contributors (1). Drug therapy (including duloxetine; not approved in the US) has limited efficacy and frequent adverse effects (2). Current surgical treatment is efficacious, but is also limited by adverse events such as complications with implanted permanent synthetic meshes and postoperative voiding dysfunction. This and the unfavorable long-term recurrence rates have led to a quest for the development of new non-surgical approaches. Stem cell therapy (SCT) seems a promising strategy for restoring urethral sphincter function in patients with SUI (3-6), and in a recent systematic review of the clinical trials available on SCT for SUI in woman, Klapper-Goldstein et al. (5) concluded that this therapy is safe and effective and gives promise for the future. Mariotti et al. (6) performed a systematic review and meta-analysis of 12 clinical trials and found a 41% mean rate of continence recovery with the use of autologous muscle derived (MDSC) or adipose derived stem cells (ADSCs). However, cell therapy still has some challenges (7), and there seems to be room for improvements.
The ability of mesenchymal stem cells (MSCs) to self-renew, to form clonal cell populations and to differentiate into multiple cell lineages is well established. The cells have anti-fibrotic, angiogenic, and anti-apoptotic properties and these properties are particularly attractive for restoring function in damaged tissues as in SUI. It was originally believed that e.g., autologous MSCs, engrafted or differentiated into injured host tissue. However, it is now well established that MSCs secrete soluble factors through a paracrine mechanism, which is believed to provide the therapeutic regenerative effect (8,9). Among the secreted factors are e.g., cytokines, such as stromal derived growth factor 1 (SDF-1; CXCL12), which are believed to act as homing factors, attracting cells from the circulation and/or the bone marrow to the site of damage where they repair tissues and restore function (10).
Stem cells may also exert a significant therapeutic effect by secreting packages of bioactive factors—the “secretome”—producing their anti-fibrotic, angiogenic, and anti-apoptotic properties (8-13). The effects of Li-ESWT on stem cells in culture suggest that it may be used as a non-invasive method to stimulate cell growth and mobilization in vivo. Supporting this, recent clinical evidence (14) suggests that Li-ESWT could serve as a potentially novel treatment for SUI. Even if regenerative electrical stimulation (RES) has not been tested clinically for SUI treatment it may open new therapeutic opportunities.
Is the therapeutic use of cytokines, secretome/secretome components, Li-ESWT and RES new therapeutic frontiers for management of SUI? The aim of the present review is to give an update on recent information as an answer to this question. We present this article in accordance with the Narrative Review reporting checklist (https://tau.amegroups.com/article/view/10.21037/tau-22-682/rc).
Methods
Using PubMed, Google Scholar and Scopus, a comprehensive literature search was performed. In Table 1, the search strategy for articles included is given. Peer-reviewed original articles and reviews and randomized controlled clinical trials (when available), published between 2000 and 2023, were analyzed to identify articles of relevance. The keywords searched included: “female stress incontinence”, “homing factors”, “CXCL12”, “secretome”, “low energy shockwave therapy” and “regenerative electrical stimulation”. Publications that contained information that added to the existing body of literature within the field were included.
Table 1
Items | Specification |
---|---|
Date of search | Oct 16, 2022 |
Databases and other sources searched | PubMed, Google Scholar, Scopus |
Search terms | “female stress incontinence”, “homing factors”, “CXCL12”, “secretome”, “low energy shockwave therapy”, “regenerative electrical stimulation” |
Time frame | Between 2000 and 2023 |
Inclusion criteria | Inclusion criteria: (I) peer-reviewed original articles; (II) reviews covering the field |
Selection process | References were independently selected by the authors and included after consensus |
Discussion
Promise of “homing factors”
Karp and Leng Teo (15) defined MSC homing “as the arrest of MSCs within the vasculature of a tissue followed by transmigration across the endothelium”. A more recent definition was given by Liesveld et al. (16): “Stem cell homing refers to the ability of circulating stem cells or exogenously administered stem cells to locate and enter an environmental niche.” It has been shown that stem cells respond to gradients of chemo attractants. They migrate up the gradients and lodge within specific tissue areas. This migration is believed to be of importance in the recruitment of marrow mesenchymal and stromal stem cells to sites of injury and regeneration. This mechanism is less well understood but may be important when these cells are used for repair purposes (16,17).
As mentioned, adult transplanted MSCs may contribute to tissue repair by their paracrine function. This may well be their main mechanism of action as suggested by data from animal models using animal MSCs transplanted into the urethra. These studies showed that in vivo differentiation of the MSC into terminally differentiated muscle cells was less important than repair through secretion of trophic factors (9).
MSCs are guided by chemotactic signals such as PDGFa, insulin growth factor and CXCL12 (16). CXCL12 interacts with its receptors CXC chemokine receptor 4 (CXCR4) and atypical chemokine receptor 3 (ACKR3) to exert its physiological and pathophysiological functions (18).
There are studies suggesting that even in the absence of stem cells, the stem cell-derived secretory factors alone may be sufficient to enhance tissue repair after injury. Already in 2003 Askari et al. showed that CXCL12 was sufficient to induce therapeutic stem-cell homing to injured myocardium in rats. They suggested that therapeutic strategies focused on stem-cell mobilisation for regeneration (at least of myocardial tissue) must be initiated within days of injury (19). Williams et al. (20) showed that in non-human primates (NHPs) with chronic sphincter deficiency, local sphincter administration of CXCL12 without cell therapy, restored short-term urinary sphincter structure and function. The mechanism is still being explored but it is thought to involve an interaction between homing cytokines and their respective receptors on the cell surface of MSCs.
Studies have shown that effects of the sum of secretions from progenitor cells rather than their individual regenerative efficacy stimulate structural and functional regeneration of the urethra (21,22). Cells alone were shown unable to mobilize bone marrow cells and restore vascularization and innervation of the injured NHP sphincter, confirming their limited homing ability. In contrast CXCL12 was effective in restoring sphincter structure and function (20,23). However, there are reports that seem to contradict these results and question the beneficial effects of CXCL12 on urological tissues (24,25). Thus, Liu et al. (24) found in mice that the C-X-C motif chemokine receptor 4 aggravated renal fibrosis, and Macoska et al. (25) found that obesity-induced LUT fibrosis and voiding dysfunction in mice could be repressed by antagonizing the activity of the CXCR4 chemokine receptor. These studies focused on inhibition of the secretion of physiologic CXCL12. Ray et al. (26) showed that under physiologic conditions CXCL12 forms dimers. Use of low concentrations of monomeric CXCL12, which is the active form stimulating chemotaxis, was suggested by Veldkamp et al. (27,28). It cannot be excluded that use of high concentrations of CXCL12 may lead to pathology and malignancy and inhibition of chemotaxis. Both mammalian cells and bacteria secret monomeric CXCL12 and monomeric CXCL12 is commercially available in recombinant, purified form and currently used for treatment injections. Thus, the way of testing the molecule, the dose and its interaction with other pathways are critical factors for the translation to human use. This is illustrated by one of the few clinical trials where the effects of CXCL12 were assessed. Shishehbor et al. (29) investigated the actions of JVS-100, a non-viral DNA plasmid-based therapy that encodes CXCL12 (SDF-10) with an improved longevity. This compound was used as an adjunct to successful revascularization in a Phase 2B randomized, double-blind, placebo-controlled trial. It was found that JVS-100 could not impact wound healing at 3 months, which was the clinical endpoint parameter.
Despite the good underlying rationale and the positive findings in NHP with sphincter insufficiency, studies on the effects of monomeric CXCL12 in human stress incontinence caused by sphincter damage have, to the best of our knowledge, not yet been performed.
Other cytokines have been studied preclinically. It has been shown CCL7 (C-C motif ligand 7) can recruit MSCs to an injured area in the myocardium, where they engraft, produce growth factors, facilitate healing from injury, and improve functional recovery (30). In mice models of simulated birth trauma-induced urinary incontinence, Hijaz et al. (31) investigated the expression of CCL7. They found overexpression of urethral CCL7 after vaginal distension but not pudendal nerve transection, suggesting that nerve injury does not contribute to the CCL7 overexpression. In a model of simulated birth trauma-induced SUI in rats, Jiang et al. (32,33) combined treatment with CCR1-overexpressing MSCs and CCL7 and found that engraftment of MSCs could increase and promote functional recovery. They suggested that this could be a new therapeutic strategy for SUI.
Secretomes
As mentioned, MSCs home to sites of injury in response to cytokine gradients. A small percentage of the cells may engraft, differentiate, and integrate with host tissues. However, these mechanisms alone cannot explain their therapeutic benefit (9). There is evidence supporting the paracrine hypothesis of stem cell action, postulating that stem cells exert a significant therapeutic effect by secreting the “secretome”, which may exert significant therapeutic effects by their anti-fibrotic, angiogenic, and anti-apoptotic properties (8-10,34). A secretome is defined as all the proteins and secretory pathway constituents secreted by a cell into the extra-cellular space (Figure 1). These secreted factors include microvesicles, exosomes and apoptotic bodies and can be found in the medium where the stem cells are cultured, also called conditioned medium (CM). The exosomes have an endosome-derived membrane, whereas the microvesicles could directly bud from the plasma membrane, so they have a plasma-derived membrane. The apoptotic bodies are released during the later phase of apoptosis (34). Exosomes are characterized by a diameter of 40–150 nm and a density of 1.09–1.18 g/mL (8), whereas the microvesicles measure 100–500 nm and the apoptotic bodies 500–1,000 nm (34). The secretome of human MSCs can include growth factors, chemokines, cytokines, adhesion molecules, proteases, and receptors.
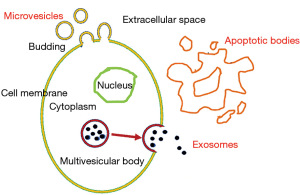
The molecules within the secretomes may enable more efficient remote communication and targeting than can be obtained with MSCs themselves. The mechanisms involved in e.g., immunomodulation, angiogenesis, inflammation, apoptosis, and tissue repair may be regulated by secretomes (8,9). Conditioned media or exosomes have a potential as a ready-to-go biologic product and may present considerable advantages over cells with respect to manufacturing, storage, handling and product shelf life (8). The use of secretomes, with or without stem cells, may thus represent a promising approach for the treatment of SUI.
Not only exosomes derived from MSCs may be useful. Wang et al. (11) evaluated the in vitro effects of extracellular vesicles secreted by rat ADSCs in a SUI model and the regulatory mechanism of delivered microRNA-93 (miR-93). ADSCs were isolated and cultured, and extracellular vesicles from the cells were extracted and identified. Their study indicated that miR-93 delivered by ADSCs-extracellular vesicles could regulate extracellular matrix remodeling of SUI fibroblasts and promote activation of SUI satellite cells through targeting F3. However, the authors used male Sprague Dawley animals and the SUI model was not described. Nevertheless, the results illustrate important effects of exosomes.
The effects of exosomes secreted by human urine-derived stem cells injected in and around the pubococcygeal muscle were tester in a rat SUI model produced by balloon dilatation of the vagina (12). They found that after the injection of exosomes there was a significant improvement of urodynamic parameters and a promotion of activation, proliferation and differentiation of muscle satellite cells.
The secretions of MSCs include brain-derived neurotrophic factor (BDNF) which can improve neuroregeneration. BDNF seems to be a key factor in the MSC secretome for the facilitation of functional recovery following a dual simulated childbirth injury (20,33,35). In female Sprague-Dawley rats, who had undergone bilateral pudendal nerve crush and vaginal distension (injury) or sham injury, Yuan et al. (36) showed that BDNF in concentrated conditioned media containing MSC secretome accelerated recovery. BDNF was considered an important contributor, but not the only factor responsible for the recovery of the nerve and muscle injury.
Low intensity shock wave therapy
The possibility of using non-invasive methods to stimulate stem cell growth and mobilization in vivo was suggested by recent demonstrations of the effects of low intensity shock wave therapy (Li-ESWT) on stem cells in culture (37-39). Such an approach avoids the requirement for stem cell acquisition, expansion, characterization, transplantation and immune responses of the recipient (40,41). The effects of Li-ESWT are multifactorial and thought to be mediated, at least partly, by upregulation of chemoattractant factors and recruitment/activation of stem/progenitor cells, but also to enhance expression of vascular endothelial growth factor, endothelial nitric oxide synthase, and proliferating cell nuclear antigen (24). Studies have shown promising results with Li-ESWT in the management of erectile dysfunction (42-44), where the potential effects on penile tissue have been suggested to include cell proliferation, enhanced cell survival, mitigation of fibrosis and inflammation, and recruitment/activation of endogenous stem cells. This may result in angiogenesis, improved wound healing, and regeneration of muscle and nerve tissue (24). Li-ESWT has been shown to stimulate regeneration of skeletal muscle tissue and accelerate tissue repair processes (41). However, another study showed that Li-ESWT had no incremental effect on PAX7 positive cells and proangiogenic genes in vivo (45).
In a single-arm, open-label, multicentre study, Long et al. (46) treated 50 female SUI patients with Li-ESWT. They found that 8 weeks of Li-ESWT attenuated SUI symptoms on physical activity, reduced bladder leaks, overactive bladder (OAB) symptoms and significantly improved quality of life.
In a multicenter, single-blind, randomized-controlled trial on sixty female SUI patients, Lin et al. (14) evaluated the effects of Li-ESWT. Primary endpoints were the changes in urine leakage as measured by a pad test and validated standardized questionnaires. The secondary endpoint was the changes in a 3-day urinary diary. Four weeks of Li-ESWT significantly decreased urine leakage based on pad test and validated standardized questionnaire scores, as compared to the sham group. Eight weeks of Li-ESWT significantly reduced urine leakage, increased urine volume and attenuated urgency symptoms. At week eight validated standardized questionnaire scores were significantly improved compared to the baseline. The authors suggested that Li-ESWT could serve as a potentially novel and non-invasive treatment for SUI.
RES
In experimental models of SUI (bilateral pudendal nerve crush and vaginal distension) nerve injury is an important component. This may be the case also in a clinical situation. The evidence for the efficacy of electrical stimulation (ES), daily exercise and their combination in promoting nerve regeneration after peripheral nerve injuries in both animal models and in human patients was reviewed by Gordon et al. (47). They concluded that both ES and exercise are promising experimental treatments for peripheral nerve injury that seem to be ready to be translated to clinical use.
Balog et al. (48) suggested in a recent review that ES could be a new therapeutic paradigm for SUI treatment. Several preclinical studies have supported this suggestion. In a study on female rats with simulated childbirth injury using a dual-injury model involving both vaginal distension and bilateral pelvic neve crush, Jiang et al. (49) showed that acute electrical stimulation of the pudendal nerve proximal to the crush site upregulated BDNF and bII-tubulin in Onuf’s nucleus, which they speculated could be a potential preventive option for SUI after childbirth injury. Jiang et al. (50) using the same model found that repeat pudendal nerve stimulation promoted neuromuscular continence, mechanism recovery, again possibly via a BDNF upregulation in the pudendal motoneurons in this model of SUI.
These studies suggested that electrical stimulation of the pudendal nerve may improve recovery after childbirth and ameliorate symptoms of SUI by promoting neuroregeneration after injury. To the best of our knowledge no such RES studies on SUI patients have been performed.
Summary and conclusions
Treatment of SUI with stem cells has been shown to be safe and effective in many patients with SUI (3,5,6). However, since their beneficial effects are mediated by secretion of factors rather than the cells themselves there is a good rationale for further exploring the therapeutic effects of both homing factors such as CXCL12 and the secretome and/or its components. A large number of human clinical studies are already available on the use of secretome products from MSCs on various conditions (51,52). However, controlled clinical trials on patients with SUI, have to the best of our knowledge, not yet been performed.
Li-ESWT may be a promising alternative and there is good clinical evidence for efficacy. This approach has similar advantages to using cell products like CXCL12 and bypasses the need for expensive and time-consuming cell therapy. RES may be a future alternative for some SUI patients, but so far clinical proof-of-concept studies are lacking.
Acknowledgments
Funding: None.
Footnote
Provenance and Peer Review: This article was commissioned by the Guest Editor (Eric Chung) for the series “50 Years Anniversary of the Modern Artificial Urinary Sphincter” published in Translational Andrology and Urology. The article has undergone external peer review.
Reporting Checklist: The authors have completed the Narrative Review reporting checklist. Available at https://tau.amegroups.com/article/view/10.21037/tau-22-682/rc
Peer Review File: Available at https://tau.amegroups.com/article/view/10.21037/tau-22-682/prf
Conflicts of Interest: Both authors have completed the ICMJE uniform disclosure form (available at https://tau.amegroups.com/article/view/10.21037/tau-22-682/coif). The series “50 Years Anniversary of the Modern Artificial Urinary Sphincter” was commissioned by the editorial office without any funding or sponsorship. Both authors have applied for a patent that covers CXCL12 for sphincter dysfunction (urinary, anal). K.W. got RO1 from NIDDK. The authors have no other conflicts of interest to declare.
Ethical Statement: The authors are accountable for all aspects of the work in ensuring that questions related to the accuracy or integrity of any part of the work are appropriately investigated and resolved.
Open Access Statement: This is an Open Access article distributed in accordance with the Creative Commons Attribution-NonCommercial-NoDerivs 4.0 International License (CC BY-NC-ND 4.0), which permits the non-commercial replication and distribution of the article with the strict proviso that no changes or edits are made and the original work is properly cited (including links to both the formal publication through the relevant DOI and the license). See: https://creativecommons.org/licenses/by-nc-nd/4.0/.
References
- Falah-Hassani K, Reeves J, Shiri R, et al. The pathophysiology of stress urinary incontinence: a systematic review and meta-analysis. Int Urogynecol J 2021;32:501-52. [Crossref] [PubMed]
- Michel MC, Cardozo L, Chermansky CJ, et al. Current and Emerging Pharmacological Targets and Treatments of Urinary Incontinence and Related Disorders. Pharmacol Rev 2023;75:554-74. [Crossref] [PubMed]
- Aragón IM, Imbroda BH, Lara MF. Cell therapy clinical trials for stress urinary incontinence: current status and perspectives. Int J Med Sci 2018;15:195-204. [Crossref] [PubMed]
- Barakat B, Franke K, Schakaki S, et al. Stem cell applications in regenerative medicine for stress urinary incontinence: A review of effectiveness based on clinical trials. Arab J Urol 2020;18:194-205. [Crossref] [PubMed]
- Klapper-Goldstein H, Tamam S, Sade S, et al. A systematic review of stem cell therapy treatment for women suffering from stress urinary incontinence. Int J Gynaecol Obstet 2022;157:19-30. [Crossref] [PubMed]
- Mariotti G, Salciccia S, Viscuso P, et al. Regenerative medicine-based treatment of stress urinary incontinence with mesenchymal stem cells: a systematic review and meta-analysis. Curr Stem Cell Res Ther 2023;18:429-37. [Crossref] [PubMed]
- Schmid FA, Williams JK, Kessler TM, et al. Treatment of Stress Urinary Incontinence with Muscle Stem Cells and Stem Cell Components: Chances, Challenges and Future Prospects. Int J Mol Sci 2021;22:3981. [Crossref] [PubMed]
- Vizoso FJ, Eiro N, Cid S, et al. Mesenchymal Stem Cell Secretome: Toward Cell-Free Therapeutic Strategies in Regenerative Medicine. Int J Mol Sci 2017;18:1852. [Crossref] [PubMed]
- Sun DZ, Abelson B, Babbar P, et al. Harnessing the mesenchymal stem cell secretome for regenerative urology. Nat Rev Urol 2019;16:363-75. [Crossref] [PubMed]
- Williams JK, Andersson KE. Regenerative pharmacology: recent developments and future perspectives. Regen Med 2016;11:859-70. [Crossref] [PubMed]
- Wang L, Wang Y, Xiang Y, et al. An In Vitro Study on Extracellular Vesicles From Adipose-Derived Mesenchymal Stem Cells in Protecting Stress Urinary Incontinence Through MicroRNA-93/F3 Axis. Front Endocrinol (Lausanne) 2021;12:693977. [Crossref] [PubMed]
- Wu R, Huang C, Wu Q, et al. Exosomes secreted by urine-derived stem cells improve stress urinary incontinence by promoting repair of pubococcygeus muscle injury in rats. Stem Cell Res Ther 2019;10:80. [Crossref] [PubMed]
- Rolland TJ, Peterson TE, Singh RD, et al. Author Correction: Exosome biopotentiated hydrogel restores damaged skeletal muscle in a porcine model of stress urinary incontinence. NPJ Regen Med 2022;7:65. [Crossref] [PubMed]
- Lin KL, Chueh KS, Lu JH, et al. Low Intensity Extracorporeal Shock Wave Therapy as a Novel Treatment for Stress Urinary Incontinence: A Randomized-Controlled Clinical Study. Medicina (Kaunas) 2021;57:947. [Crossref] [PubMed]
- Karp JM, Leng Teo GS. Mesenchymal stem cell homing: the devil is in the details. Cell Stem Cell 2009;4:206-16. [Crossref] [PubMed]
- Liesveld JL, Sharma N, Aljitawi OS. Stem cell homing: From physiology to therapeutics. Stem Cells 2020;38:1241-53. [Crossref] [PubMed]
- Ullah M, Liu DD, Thakor AS. Mesenchymal Stromal Cell Homing: Mechanisms and Strategies for Improvement. iScience 2019;15:421-38. [Crossref] [PubMed]
- Janssens R, Struyf S, Proost P. The unique structural and functional features of CXCL12. Cell Mol Immunol 2018;15:299-311. [Crossref] [PubMed]
- Askari AT, Unzek S, Popovic ZB, et al. Effect of stromal-cell-derived factor 1 on stem-cell homing and tissue regeneration in ischaemic cardiomyopathy. Lancet 2003;362:697-703. [Crossref] [PubMed]
- Williams JK, Dean A, Badra S, et al. Cell versus Chemokine Therapy in a Nonhuman Primate Model of Chronic Intrinsic Urinary Sphincter Deficiency. J Urol 2016;196:1809-15. [Crossref] [PubMed]
- Lavoie JR, Rosu-Myles M. Uncovering the secretes of mesenchymal stem cells. Biochimie 2013;95:2212-21. [Crossref] [PubMed]
- Deng K, Lin DL, Hanzlicek B, et al. Mesenchymal stem cells and their secretome partially restore nerve and urethral function in a dual muscle and nerve injury stress urinary incontinence model. Am J Physiol Renal Physiol 2015;308:F92-F100. [Crossref] [PubMed]
- Williams JK, Mariya S, Suparto I, et al. Cell Versus Chemokine Therapy Effects on Cell Mobilization to Chronically Dysfunctional Urinary Sphincters of Nonhuman Primates. Int Neurourol J 2018;22:260-7. [Crossref] [PubMed]
- Liu Y, Feng Q, Miao J, et al. C-X-C motif chemokine receptor 4 aggravates renal fibrosis through activating JAK/STAT/GSK3β/β-catenin pathway. J Cell Mol Med 2020;24:3837-55. [Crossref] [PubMed]
- Macoska JA, Wang Z, Virta J, et al. Inhibition of the CXCL12/CXCR4 axis prevents periurethral collagen accumulation and lower urinary tract dysfunction in vivo. Prostate 2019;79:757-67. [Crossref] [PubMed]
- Ray P, Lewin SA, Mihalko LA, et al. Secreted CXCL12 (SDF-1) forms dimers under physiological conditions. Biochem J 2012;442:433-42. [Crossref] [PubMed]
- Veldkamp CT, Seibert C, Peterson FC, et al. Structural basis of CXCR4 sulfotyrosine recognition by the chemokine SDF-1/CXCL12. Sci Signal 2008;1:ra4. [Crossref] [PubMed]
- Veldkamp CT, Ziarek JJ, Su J, et al. Monomeric structure of the cardioprotective chemokine SDF-1/CXCL12. Protein Sci 2009;18:1359-69. [Crossref] [PubMed]
- Shishehbor MH, Rundback J, Bunte M, et al. SDF-1 plasmid treatment for patients with peripheral artery disease (STOP-PAD): Randomized, double-blind, placebo-controlled clinical trial. Vasc Med 2019;24:200-7. [Crossref] [PubMed]
- Schenk S, Mal N, Finan A, et al. Monocyte chemotactic protein-3 is a myocardial mesenchymal stem cell homing factor. Stem Cells 2007;25:245-51. [Crossref] [PubMed]
- Hijaz AK, Grimberg KO, Tao M, et al. Stem cell homing factor, CCL7, expression in mouse models of stress urinary incontinence. Female Pelvic Med Reconstr Surg 2013;19:356-61. [Crossref] [PubMed]
- Jiang HH, Ji LX, Li HY, et al. Combined Treatment With CCR1-Overexpressing Mesenchymal Stem Cells and CCL7 Enhances Engraftment and Promotes the Recovery of Simulated Birth Injury-Induced Stress Urinary Incontinence in Rats. Front Surg 2020;7:40. [Crossref] [PubMed]
- Jiang M, Liu J, Liu W, et al. Bone marrow stem cells secretome accelerates simulated birth trauma-induced stress urinary incontinence recovery in rats. Aging (Albany NY) 2021;13:10517-34. [Crossref] [PubMed]
- Hashemi ZS, Ghavami M, Khalili S, et al. Chapter 5: The emerging role of exosome nanoparticles in regenerative medicine. In: Madhyastha H, Chauhan DN. editors. Nanopharmaceuticals in Regenerative Medicine. Boca Raton: CRC Press; 2022:67-93.
- Janssen K, Lin DL, Hanzlicek B, et al. Multiple doses of stem cells maintain urethral function in a model of neuromuscular injury resulting in stress urinary incontinence. Am J Physiol Renal Physiol 2019;317:F1047-57. [Crossref] [PubMed]
- Yuan X, Balog BM, Lin DL, et al. Brain-Derived Neurotrophic Factor Is an Important Therapeutic Factor in Mesenchymal Stem Cell Secretions for Treatment of Traumatic Peripheral Pelvic Injuries. Front Cell Neurosci 2022;16:866094. [Crossref] [PubMed]
- Zhao Y, Wang J, Wang M, et al. Activation of bone marrow-derived mesenchymal stromal cells-a new mechanism of defocused low-energy shock wave in regenerative medicine. Cytotherapy 2013;15:1449-57. [Crossref] [PubMed]
- Lin G, Reed-Maldonado AB, Wang B, et al. In Situ Activation of Penile Progenitor Cells With Low-Intensity Extracorporeal Shockwave Therapy. J Sex Med 2017;14:493-501. [Crossref] [PubMed]
- Chen PY, Cheng JH, Wu ZS, et al. New Frontiers of Extracorporeal Shock Wave Medicine in Urology from Bench to Clinical Studies. Biomedicines 2022;10:675. [Crossref] [PubMed]
- Ventura C. Tuning stem cell fate with physical energies. Cytotherapy 2013;15:1441-3. [Crossref] [PubMed]
- Zissler A, Steinbacher P, Zimmermann R, et al. Extracorporeal Shock Wave Therapy Accelerates Regeneration After Acute Skeletal Muscle Injury. Am J Sports Med 2017;45:676-84. [Crossref] [PubMed]
- Dong L, Chang D, Zhang X, et al. Effect of Low-Intensity Extracorporeal Shock Wave on the Treatment of Erectile Dysfunction: A Systematic Review and Meta-Analysis. Am J Mens Health 2019;13:1557988319846749. [Crossref] [PubMed]
- Raheem OA, Natale C, Dick B, et al. Novel treatments of erectile dysfunction: review of the current literature. Sex Med Rev 2021;9:123-32. [Crossref] [PubMed]
- Porst H. Review of the Current Status of Low Intensity Extracorporeal Shockwave Therapy (Li-ESWT) in Erectile Dysfunction (ED), Peyronie's Disease (PD), and Sexual Rehabilitation After Radical Prostatectomy With Special Focus on Technical Aspects of the Different Marketed ESWT Devices Including Personal Experiences in 350 Patients. Sex Med Rev 2021;9:93-122. [Crossref] [PubMed]
- Hansen LK, Schrøder HD, Lund L, et al. The effect of low intensity shockwave treatment (Li-SWT) on human myoblasts and mouse skeletal muscle. BMC Musculoskelet Disord 2017;18:557. [Crossref] [PubMed]
- Long CY, Lin KL, Lee YC, et al. Therapeutic effects of Low intensity extracorporeal low energy shock wave therapy (LiESWT) on stress urinary incontinence. Sci Rep 2020;10:5818. [Crossref] [PubMed]
- Gordon T, English AW. Strategies to promote peripheral nerve regeneration: electrical stimulation and/or exercise. Eur J Neurosci 2016;43:336-50. [Crossref] [PubMed]
- Balog BM, Deng K, Labhasetwar V, et al. Electrical stimulation for neuroregeneration in urology: a new therapeutic paradigm. Curr Opin Urol 2019;29:458-65. [Crossref] [PubMed]
- Jiang HH, Gill BC, Dissaranan C, et al. Effects of acute selective pudendal nerve electrical stimulation after simulated childbirth injury. Am J Physiol Renal Physiol 2013;304:F239-47. [Crossref] [PubMed]
- Jiang HH, Song QX, Gill BC, et al. Electrical stimulation of the pudendal nerve promotes neuroregeneration and functional recovery from stress urinary incontinence in a rat model. Am J Physiol Renal Physiol 2018;315:F1555-64. [Crossref] [PubMed]
- Mendt M, Rezvani K, Shpall E. Mesenchymal stem cell-derived exosomes for clinical use. Bone Marrow Transplant 2019;54:789-92. [Crossref] [PubMed]
- Lee BC, Kang I, Yu KR. Therapeutic Features and Updated Clinical Trials of Mesenchymal Stem Cell (MSC)-Derived Exosomes. J Clin Med 2021;10:711. [Crossref] [PubMed]