Microenergy shockwave therapies for female stress urinary incontinence
Introduction
Stress urinary incontinence (SUI) is the symptom of urinary leakage during moments of physical activity that increases abdominal pressure. SUI occurs when there are impairments in the structure and function of the urethral sphincter and pelvic floor muscles, resulting in leakage of urine during sneezing, coughing, or physical exertion. SUI is a major problem in urogynecology. The worldwide incidence of SUI was projected to affect 167 million people (a prevalence of 3.3%) by 2018 (1).
The growing awareness of complications related to mesh and urethral sling procedures has generated interest in the use of regenerative medicine techniques to restore the structure and function of the urethral sphincter and pelvic floor (2). Stem cell therapy and tissue engineering with stem cells currently dominate the research field for SUI and pelvic floor prolapse (POP) respectively (3-5). While there is increasing interest in using stem cell products and tissue-resident stem/progenitor cells to improve the regenerative process for SUI, stem cell therapy still requires significant investigation before it can be implemented clinically. Previous studies have shown promising results, and further research is needed to fully understand the potential benefits and risks of this approach (6).
To study the pathophysiology of SUI and potential therapeutic options, various animal models have been developed and tested in the past three decades. Animal models used include rodents, rabbits, dogs, rhesus monkeys, and transgenic obese rats. The methods of creating the models range from urethral dilation, vaginal distension, local electrocautery, pudendal nerve crush or transection to urethrolysis, urethral sphincterotomy and pubourethral ligament transection (7).
In 1998, Lin and associates reported a rat model of SUI by vaginal balloon distension (VBD) for 4 hours to mimic injury to the urethra and pelvic floor from prolonged 2nd stage labor during childbirth. This method has been modified and used by other researchers as a reversible, short-term model of stress urinary. Researchers also developed a highly reproducible, long-term, irreversible animal model of SUI with a combination of VBD and lysyl oxidase inhibition resulting in disorganized collagen, elastin, and muscle fibers.
Many researchers have conducted extensive studies to better understand the reversible and irreversible changes in the urethra and pelvic floor in these animal models. These examinations include the microarchitecture and molecular biological changes of the mucosa, submucosal vascular spaces, smooth muscle, striated muscles, collagen, and elastic fibers, as well as neural innervation and blood supply (8-12). They also identified the distribution of tissue-resident stem/progenitor cells after VBD injury and lysyl oxidase inhibition (8-12).
In 2010, researchers began to examine the feasibility of stem cell therapy in regenerating urethral tissue and improving sphincter function. After several years, they noticed several main shortcomings of adipose-derived stem cells (ADSCs) injection: the injected ADSCs did not differentiate and integrate into host tissue; they also moved to lungs, spleen, and bone marrow after a few days (13). The beneficial effects seem to derive from the paracrine effects of the injected ADSCs (14,15). To explore better alternatives, some researchers began to use low-intensity extracorporeal shock waves (Li-ESWT) to activate tissue-resident stem cells in the urethra and pelvic floor (Figure 1). The objective of this review is to summarize the potential mechanisms associated with microenergy shockwave therapies for SUI, including Li-ESWT and microenergy acoustic pulses (MAP).
Low intensity extracorporeal shockwave therapy and microenergy acoustic pulse therapy for stress urinary incontinence
In 2010, Vardi et al. (16) reported a pilot study of applying Li-ESWT to improve erectile function in men with vasculogenic erectile dysfunction. To obtain better tissue distribution of shock wave energy, a non-focused hydroelectric device was used to conduct early exploratory animal experiments. When the concept of extracorporeal resident stem cell activation was developed, the researchers decided to use a non-focused electromagnetic device that has more consistent waveforms and better energy distribution. The technology was named MAP to differentiate it from waveforms produced by other devices (17). The mechanobiological effects of MAP on the bladder, urethra, anal sphincter, and pelvic floor muscles in animal models were examined (18).
Pre-clinical studies of non-focused Li-ESWT in animal models of SUI
The effects of early Li-ESWT in vaginal balloon distension-induced rat model of SUI
In 2016, the study investigated the effects of Li-ESWT on SUI using the VBD rat model. The rats were grouped into three, including the normal control, VBD, and VBD with a non-focused electrohydraulic Li-ESWT, while the Li-ESWT was applied twice per week for 3 weeks immediately following the VBD. The results of leak-point pressure (LPP) showed that rats treated with Li-ESWT following VBD had significantly higher LPP compared to those receiving VBD only. Histological examinations demonstrated increased urethral sphincter regeneration and vascularity in the Li-ESWT group. Furthermore, Li-ESWT-treated rats showed a significant increase in EdU+ label-retaining cells (LRCs). This preclinical study indicates that Li-ESWT ameliorated SUI by enhancing angiogenesis, progenitor cell recruitment, and urethral sphincter regeneration (19).
The effects of Li-ESWT in an irreversible, long-term rat model of SUI
One of the major drawbacks of the VBD model is the reversible nature of SUI especially in younger rats that underwent VBD for less than 4 hours in duration. Extracellular fibers including elastic and collagen fibers are essential elements in the maintenance of sphincter structure and function. To produce a long-term model of SUI, a lysyl oxidase inhibitor, β-aminopropionitrile (BAPN) was added, after VBD to block restoration of collagen, elastin and muscles and thus makes the SUI irreversible (20).
The rats were also assigned into 3 groups: sham, VBD + BAPN, and VBD + BAPN + Li-ESWT group. Six weeks after VBD, Li-ESWT therapy was administered twice a week for two weeks. Following a one-week washout period in the treatment group, functional and histological studies were conducted on all 24 rats. The results of the functional analysis with LPP measurement showed that the VBD + BAPN + Li-ESWT group had significantly higher LPPs compared to the VBD + BAPN group. Notably, the VBD + BAPN + Li-ESWT group demonstrated an increase in urethral and vaginal smooth and striated muscle content, as well as an increase in the thickness of the vaginal wall. Additionally, the VBD + BAPN group showed an increase in tyrosine hydroxylase (TH)/neuronal nitric oxide (nNOS)-positive nerves in the smooth muscle layers of the urethra, while the VBD + BAPN + Li-ESWT group had fewer TH+ nerves and more nNOS+ nerves. Moreover, there was a significant increase in EdU-LRCs in the VBD + BAPN + Li-ESWT group as well, indicating that Li-ESWT could potentially activate local stem/progenitor cells in the urethral wall of long-term SUI animals. In summary, these results suggest that Li-ESWT treatment can increase smooth muscle content in the urethra and vagina, improve striated muscle content and neuromuscular junctions, restore the integrity of the urothelium, and increase the number of EdU-retaining progenitor cells in the urethral wall of long-term SUI animals (10).
The effects of MAP therapy for obesity-associated stress urinary incontinence
Starting in 2015, researchers began to use a non-focused electromagnetic non-focused device that has more consistent wave forms and better energy distribution. The therapeutic effect of this technology on SUI was verified in another animal model for SUI, the obesity-associated stress urinary incontinence (OA-SUI). The impact of MAP therapy on the structure and function of the urethra and pelvic floor in female ZUC-Leprfa 186 (ZL) and fatty ZUC-Leprfa 185 (ZF) have been investigated. A total of 48 rats were randomly divided into four groups: ZL, ZL+MAP, ZF, and ZF+MAP. The MAP treatment was applied twice a week for four weeks. The results showed that ZF rats had lower LPP compared to ZL rats and MAP treatment significantly improved the LPP in the ZF rats. Furthermore, impaired muscle contractile activity (MCA) was observed in the ZF rats in the organ bath study, which was significantly increased by MAP treatment. The thickness of the striated muscle layer and the number of neuromuscular junctions (NMJ) were also increased with MAP therapy. This preclinical study revealed that obesity impairs the function of both the urethral sphincter and the pelvic floor, leading to atrophy and distortion of the striated muscle fibers, which contributes to OA-SUI. MAP therapy improved urethral structure and sphincter function from activation of muscle satellite stem cells as well as inducing neural regeneration (17).
Clinical trials of focused electromagnetic Li-ESWT for SUI
In 2021, Lin et al. conducted a multicenter, single-blind, randomized controlled clinical trial after a smaller pilot study (ClinicalTrials.gov., NCT04059133) (21). The SUI patients were treated with focused Li-ESWT once a week for 4 weeks (W4) or 8 weeks (W8), or a sham device without energy output. The clinical efficacy of Li-ESWT was evaluated primarily by measuring the change in urine leakage using the pad weight test and validated standardized questionnaires, while the secondary endpoint was the changes in a 3-day urinary diary at baseline (W0), W4, and W8 of Li-ESWT, as well as 1-month (F1), 3-month (F3), and 6-month (F6) follow-up after Li-ESWT. The results indicated a significant reduction in urine leakage following 4 weeks of Li-ESWT compared to the sham group, as evidenced by the pad test and validated standardized questionnaire scores. Moreover, 8 weeks of Li-ESWT led to a significant decrease in urine leakage, an increase in urine volume, and a reduction in urgency symptoms. Furthermore, validated standardized questionnaire scores significantly improved at W8, F1, F3, and F6 compared to the baseline (W0) (21,22).
Molecular mechanisms of Li-ESWT and MAP therapy for SUI
The mechanisms of Li-ESWT and MAP therapy have been postulated to involve 4 stages of action: propagation, physic effect, mechanotransduction, and biological effects (Figure 2).
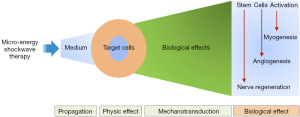
Activation of tissue-resident stem cells
Human bodies are made up of many different cells, including stem cells, progenitor cells and terminally differentiated cells. Self-renewing tissue-resident stem cells (TRSCs) and rapidly proliferating stem cell-generated progenitors are essential for maintaining tissue homeostasis by balancing proliferation and differentiation. The extent of cellular turnover required for homeostasis varies depending on the tissue type. For instance, in an adult human body, approximately 1011 cells die every day (23). While cellular replacement is constant in the blood, epidermis, and intestine, it is episodic in hair follicles and lactating mammary glands, and limited in the brain and muscle (24). However, the homeostatic requirements are also dependent on the context. Even quiescent stem cells, such as those in muscle, can be activated in response to tissue injury (25).
To explore whether quiescent muscle stem cells can be activated with mechanical stimuli without tissue injury, Kang and associates [2019] applied a previously determined safe dosage of MAP to animal models of obesity-associated SUI as described above (9,17). The female Zucker Lean and Zucker Fatty rats were randomly allocated into four groups: ZL, ZL + MAP, ZF, and ZF + MAP. The rats received MAP therapy, following which the urethra and pelvic floor muscles were harvested for cell isolation and flow cytometry assay. The freshly isolated cells were evaluated for Pax7, Int-7α, H3P, and EdU expression by flow cytometry. Pelvic floor muscle-derived stem cells (MDSCs) were collected through magnetic-activated cell sorting (MACS), and MAP therapy was administered to the MDSCs to explore the mechanisms associated with stem cell activation. The study results revealed that obesity reduced the number of EdU-LRC and satellite cells in the pelvic floor muscle and urethra. However, MAP therapy significantly enhanced cell proliferation and differentiation, leading to the regeneration of striated muscle cells in the pelvic floor and urethral sphincter, without causing cell death or tissue injury. We also demonstrated that MAP therapy activates the focal adhesion kinase (FAK)/AMP-activated protein kinase (AMPK)/Wnt/β-catenin signaling pathways, which is a potential mechanism for stem cell activation (9,26,27).
To further verify the MAP-induced activation of urethral and pelvic floor tissue-resident stem cells, another animal model of SUI was used. The rats were also randomly assigned into 4 groups: sham, VBDO, VBDO + BAPN, and VBDO + BAPN + MAP. MAP therapy was applied twice a week for four weeks, followed by a one-week washout period. After this, all 24 rats underwent functional and histological evaluations. The urethral vascular plexus was examined by using immunofluorescence staining. Furthermore, the urethral smooth muscle stem/progenitor cells (uSMPCs) were isolated and studied both in vivo and in vitro. The findings revealed that MAP treatment enhanced the regeneration of both urethral and vaginal smooth muscle. In vivo, MAP increased the number of uSMPCs, whereas in vitro, MAP induced uSMPCs to differentiate into smooth muscle and neuron-like cells, demonstrating their “stemness” (12).
Regeneration of musculature in the urethra and pelvic floor
In 2017, it was reported that Li-ESWT promoted skeletal muscle regeneration (28). In this study, the authors observed that Li-ESWT treated muscles had significantly higher levels of pax7-positive satellite cells, mitotically active H3P+ cells, and cells expressing the myogenic regulatory factors myoD and myogenin, indicating increased rates of satellite cell proliferation and differentiation. Meanwhile, Zhang and colleagues [2022] investigated the effects of MAP therapy on a mouse model of unilateral hindlimb ischemia-reperfusion injury (29). The findings indicated that MAP therapy led to a significant increase in muscle weight and centrally nucleated regenerating muscle fiber size, and also showed a trend towards activation of satellite cells. In vitro experiments demonstrated that MAP promoted myoblast proliferation and differentiation as well as endothelial cell migration.
To investigate the potential of using MAP therapy to promote myogenesis of the external urethral sphincter and explore the associated signaling pathways, the urethral muscle-derived stem cells (uMDSCs) were harvested from Zucker Lean rats and exposed to MAP therapy and GSK2656157. The results showed that uMDSCs were capable of forming myotubes in culture, and MAP therapy significantly enhanced myotube formation. Moreover, MAP activated the protein kinase RNA-like ER kinase (PERK) pathway by increasing the phosphorylation levels of PERK and eukaryotic initiation factor 2a (eIF2α), and upregulating activating transcription factor 4 (ATF4). Inhibition of the PERK pathway using GSK2656157 effectively decreased myotube formation in L6 rat myoblast cells and attenuated the myotube formation induced by MAP (27).
The regenerative effect of MAP on the external urinary sphincter was also studied in the obesity-associated stress urinary incontinence (OA-SUI) rat model. The regenerative potential of MAP on the external urinary sphincter was investigated in an OA-SUI rat model. Organ-bath studies showed impaired MCA in Zucker fatty rats, but MAP treatment significantly improved MCA and increased the thickness of the striated muscle layer and the number of neuromuscular junctions (17). In the long-term irreversible BAPN-treated models of SUI, disrupted and attenuated elastic and collagen fibers as well as disorganized striated and smooth muscle fibers were the prominent features. MAP therapy effectively ameliorated the deterioration of elastic fibers, smooth and striated muscles in the urethra and vagina, as well as the submucosal vascular plexus in the urethra (12).
Improvement in biomechanical property of pelvic floor muscles
In addition to improvement of micro-architecture, recent studies also demonstrated that MAP promoted the biomechanical properties of the pelvic floor muscles (11). In this study, rats were randomly divided into four groups: sham, VBDO, VBDO + BAPN, and VBDO + BAPN + MAP also. The viscoelastic properties of the pelvic floor muscles, including iliococcygeus (IC) and pubococcygeus (PC), were examined using a biomechanical assay. The structure of the pelvic floor muscles was also assessed using immunofluorescence and Masson’s trichrome staining. The results showed that in the sham group, the muscular stiffness of IC muscle was higher than that of PC muscle, while the pelvic floor muscle rebound activity of PC muscle was stronger than that of IC muscle. There was a decrease in muscle rebound activity and an increase in muscular stiffness in both IC and PC muscles in VBDO and BAPN groups. The histologic examination revealed increased fibrous tissue and degeneration of muscle fibers while MAP therapy decreased the collagen content and improved the architecture of muscle fibers. The data suggest that the improvement in the structure and function of pelvic floor muscles is likely due to MAP-induced muscle regeneration and improved biomechanical properties.
Modulation of cellular signaling pathways associated with Li-ESWT and MAP therapies of SUI
Extensive research demonstrated that the mechanisms involve neovascularization, tissue regeneration, and inflammation reduction. These effects are believed to result from an increase in the expression of vascular endothelial growth factor, endothelial nitric oxide synthase, and proliferating cell nuclear antigen. Recent studies also found that the following signaling pathways are regulated by MAP therapy: FAK, extracellular-signal-regulated kinase (ERK), Notch, PI3K/AKT, Wnt/beta-catenin, PERK/ATF, vascular endothelial growth factor (VEGF) and brain-derived neurotrophic factor (BDNF) (30).
The regeneration of striated muscle relies on the activation of tissue-resident muscle stem cells (Satellite cells). The activation of these cells is partly mediated by the induced expression of MYOD and myogenic factor 5 (MYF5). These differentiated myoblasts then fuse with each other and remaining myofibers to repair the damaged muscle. Satellite cells that are activated but do not commit to differentiation undergo self-renewing proliferation, which replenishes the muscle satellite cell pool (31). In addition, after muscle injury, quiescent satellite cells are exposed to extrinsic, pro-myogenic stimuli and these stimuli activate intrinsic pathways that stimulate proliferation. The activation of satellite cells involves the p38α/β-MAPK, as well as the symmetric cell division activator, Wnt7a signaling (31).
The striated urethral sphincter muscle is different from other striated muscles in its circular orientation, smaller and shorter fiber size, constrictive function and no skeletal attachment. Therefore, more in-depth studies to define its stem cells and regenerative mechanisms are warranted. Cui and associates identified uSMSCs from female rats with 3 stem cell markers: Pax7, Sox2, and Lin28 by immunofluorescence examination in female rats. They also isolated and purified the uSMSCs. In cell culture, myotube formation by these cells were enhanced by MAP therapy (27). In another study with flowcytometry, Wang et al. [2018] reported that multiple stem cell markers were expressed alone or in combination in the uSMSCs. Additionally, it has been reported that the formation of myotubes in L6 myoblasts, induced by Li-ESWT, may be attributed to PERK signaling (26) (Figure 3).
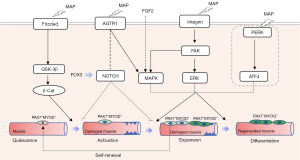
Perspectives
Female stress urinary incontinence is a result of failed closure of the urethral sphincter and inadequate support of the adjacent pelvic floor muscles. The urethral closure mechanism involves striated and smooth muscles, innervating nerves, submucosal vascular plexus, and intact mucosa. Microenergy therapy with Li-ESWT and MAP has shown promising results in limited preclinical and clinical studies. Nevertheless, the studies cited in this brief summary only scratch the surface of a very promising non-invasive regenerative therapy. Further studies on the mechano-biologic effects of the microenergy therapies on all components of the urinary sphincter mechanism including activation of various tissue-resident stem cells are urgently needed.
Acknowledgments
Funding: This study was supported by
Footnote
Provenance and Peer Review: This article was commissioned by the Guest Editor (Eric Chung) for the series “50 Years Anniversary of the Modern Artificial Urinary Sphincter” published in Translational Andrology and Urology. The article has undergone external peer review.
Peer Review File: Available at https://tau.amegroups.com/article/view/10.21037/tau-23-9/prf
Conflicts of Interest: Both authors have completed the ICMJE uniform disclosure form (available at https://tau.amegroups.com/article/view/10.21037/tau-23-9/coif). The series “50 Years Anniversary of the Modern Artificial Urinary Sphincter” was commissioned by the editorial office without any funding or sponsorship. T.F.L. is a consultant to Acoustic Wave Cell Therapy, Inc. T.F.L. serves as the Editor-in-Chief of Translational Andrology and Urology. G.L. serves as an unpaid editorial board member of Translational Andrology and Urology from August 2022 to July 2024. The authors have no other conflicts of interest to declare.
Ethical Statement: The authors are accountable for all aspects of the work in ensuring that questions related to the accuracy or integrity of any part of the work are appropriately investigated and resolved.
Open Access Statement: This is an Open Access article distributed in accordance with the Creative Commons Attribution-NonCommercial-NoDerivs 4.0 International License (CC BY-NC-ND 4.0), which permits the non-commercial replication and distribution of the article with the strict proviso that no changes or edits are made and the original work is properly cited (including links to both the formal publication through the relevant DOI and the license). See: https://creativecommons.org/licenses/by-nc-nd/4.0/.
References
- D'Angelo W, Dziki J, Badylak SF. The challenge of stress incontinence and pelvic organ prolapse: revisiting biologic mesh materials. Curr Opin Urol 2019;29:437-42. [Crossref] [PubMed]
- Bennington J, Williams JK, Andersson KE. New concepts in regenerative medicine approaches to the treatment of female stress urinary incontinence. Curr Opin Urol 2019;29:380-4. [Crossref] [PubMed]
- Lin CS, Lue TF. Stem cell therapy for stress urinary incontinence: a critical review. Stem Cells Dev 2012;21:834-43. [Crossref] [PubMed]
- Gill BC, Damaser MS, Vasavada SP, et al. Stress incontinence in the era of regenerative medicine: reviewing the importance of the pudendal nerve. J Urol 2013;190:22-8. [Crossref] [PubMed]
- Thaker H, Sharma AK. Regenerative medicine based applications to combat stress urinary incontinence. World J Stem Cells 2013;5:112-23. [Crossref] [PubMed]
- Blau HM, Daley GQ. Stem Cells in the Treatment of Disease. N Engl J Med 2019;380:1748-60. [Crossref] [PubMed]
- Amend B, Harland N, Knoll J, et al. Large Animal Models for Investigating Cell Therapies of Stress Urinary Incontinence. Int J Mol Sci 2021;22:6092. [Crossref] [PubMed]
- Lin G, Shindel AW, Banie L, et al. Molecular mechanisms related to parturition-induced stress urinary incontinence. Eur Urol 2009;55:1213-22. [Crossref] [PubMed]
- Kang N, Peng D, Wang B, et al. The effects of microenergy acoustic pulses on animal model of obesity-associated stress urinary incontinence. Part 2: In situ activation of pelvic floor and urethral striated muscle progenitor cells. Neurourol Urodyn 2019;38:2140-50. [Crossref] [PubMed]
- Zhang X, Ruan Y, Wu AK, et al. Delayed Treatment With Low-intensity Extracorporeal Shock Wave Therapy in an Irreversible Rat Model of Stress Urinary Incontinence. Urology 2020;141:187.e1-7. [Crossref] [PubMed]
- Lin G, Van Kuiken M, Wang G, et al. Microenergy acoustic pulse therapy restores function and structure of pelvic floor muscles after simulated birth injury. Transl Androl Urol 2022;11:595-606. [Crossref] [PubMed]
- Tan Y, Reed-Maldonado AB, Wang G, et al. Microenergy acoustic pulse therapy restores urethral wall integrity and continence in a rat model of female stress incontinence. Neurourol Urodyn 2022;41:1323-35. [Crossref] [PubMed]
- Lin G, Huang YC, Shindel AW, et al. Labeling and tracking of mesenchymal stromal cells with EdU. Cytotherapy 2009;11:864-73. [Crossref] [PubMed]
- Albersen M, Fandel TM, Lin G, et al. Injections of adipose tissue-derived stem cells and stem cell lysate improve recovery of erectile function in a rat model of cavernous nerve injury. J Sex Med 2010;7:3331-40. [Crossref] [PubMed]
- Gorodetsky R, Aicher WK. Allogenic Use of Human Placenta-Derived Stromal Cells as a Highly Active Subtype of Mesenchymal Stromal Cells for Cell-Based Therapies. Int J Mol Sci 2021;22:5302. [Crossref] [PubMed]
- Vardi Y, Appel B, Jacob G, et al. Can low-intensity extracorporeal shockwave therapy improve erectile function? A 6-month follow-up pilot study in patients with organic erectile dysfunction. Eur Urol 2010;58:243-8. [Crossref] [PubMed]
- Wang B, Ruan Y, Zhou T, et al. The effects of microenergy acoustic pulses on an animal model of obesity-associated stress urinary incontinence. Part 1: Functional and histologic studies. Neurourol Urodyn 2019;38:2130-9. [Crossref] [PubMed]
- Wang HS, Oh BS, Wang B, et al. Low-intensity extracorporeal shockwave therapy ameliorates diabetic underactive bladder in streptozotocin-induced diabetic rats. BJU Int 2018;122:490-500. [Crossref] [PubMed]
- Wu L, Zhang X, Xiao N, et al. Functional and morphological alterations of the urinary bladder in type 2 diabetic FVB(db/db) mice. J Diabetes Complications 2016;30:778-85. [Crossref] [PubMed]
- Wang G, Lin G, Zhang H, et al. Effects of prolonged vaginal distension and β-aminopropionitrile on urinary continence and urethral structure. Urology 2011;78:968.e13-9. [Crossref] [PubMed]
- Lin KL, Chueh KS, Lu JH, et al. Low Intensity Extracorporeal Shock Wave Therapy as a Novel Treatment for Stress Urinary Incontinence: A Randomized-Controlled Clinical Study. Medicina (Kaunas) 2021;57:947. [Crossref] [PubMed]
- Long CY, Lin KL, Lee YC, et al. Therapeutic effects of Low intensity extracorporeal low energy shock wave therapy (LiESWT) on stress urinary incontinence. Sci Rep 2020;10:5818. [Crossref] [PubMed]
- Sender R, Milo R. The distribution of cellular turnover in the human body. Nat Med 2021;27:45-8. [Crossref] [PubMed]
- Fuchs E, Blau HM. Tissue Stem Cells: Architects of Their Niches. Cell Stem Cell 2020;27:532-56. [Crossref] [PubMed]
- Fu X, Zhuang CL, Hu P. Regulation of muscle stem cell fate. Cell Regen 2022;11:40. [Crossref] [PubMed]
- Wang B, Zhou J, Banie L, et al. Low-intensity extracorporeal shock wave therapy promotes myogenesis through PERK/ATF4 pathway. Neurourol Urodyn 2018;37:699-707. [Crossref] [PubMed]
- Cui K, Kang N, Banie L, et al. Microenergy acoustic pulses induced myogenesis of urethral striated muscle stem/progenitor cells. Transl Androl Urol 2019;8:489-500. [Crossref] [PubMed]
- Zissler A, Steinbacher P, Zimmermann R, et al. Extracorporeal Shock Wave Therapy Accelerates Regeneration After Acute Skeletal Muscle Injury. Am J Sports Med 2017;45:676-84. [Crossref] [PubMed]
- Zhang H, Kim HT, Feeley BT, et al. Microenergy acoustic pulses promotes muscle regeneration through in situ activation of muscle stem cells. J Orthop Res 2022;40:1621-31. [Crossref] [PubMed]
- Liu T, Shindel AW, Lin G, et al. Cellular signaling pathways modulated by low-intensity extracorporeal shock wave therapy. Int J Impot Res 2019;31:170-6. [Crossref] [PubMed]
- Almada AE, Wagers AJ. Molecular circuitry of stem cell fate in skeletal muscle regeneration, ageing and disease. Nat Rev Mol Cell Biol 2016;17:267-79. [Crossref] [PubMed]