A stepwise approach for functional near infrared spectroscopy measurement during natural bladder filling
Highlight box
Key findings
• The study provides a methodological framework that adopts frontal cortex functional near infrared spectroscopy (fNIRS) as a non-invasive technique for future researchers investigating brain-bladder signaling in overactive bladder and other lower urinary tract dysfunction.
What is known and what is new?
• fNIRS has been reported as a tool for measuring bladder sensation; however, most of the published work used an 3D brain model that averages data within the region of interest.
• We propose a methodological approach for optimization of data acquisition and analysis with a preliminary data set of eight participants. The work may aid future research.
What is the implication, and what should change now?
• The current work provides a framework enabling the use of fNIRS as a cost effective and non-invasive technique to correlate bladder sensation with cortical brain activity during natural filling.
• Future research may include larger sample sizes and attempts to a fully automate signal processing.
Introduction
Micturition requires complex coordination of sensory inputs and neural processing in a socially appropriate context. Functional magnetic resonance imaging (fMRI) studies and analysis of deficits from pathologic lesions identified the periaqueductal gray (PAG) and prefrontal cortex as key areas controlling voluntary voiding (1). Moreover, the prefrontal cortex receives inputs from the limbic system and may help determine the emotional and situational contexts for voluntary voiding (2,3). However, studies of the prefrontal cortex using fMRI are expensive and require supine positioning, making it less ideal for investigations of the neural processing of bladder sensation and voiding.
Cortical functional near infrared spectroscopy (fNIRS) measures changes in neuroexcitation based on the chromophores of oxy and deoxy hemoglobin to a cortical depth of 1.5 cm (4,5). Using fNIRS, several investigators have shown increased oxyhemoglobin (O2Hb) concentration in cortical regions associated with bladder filling or pressure over short time intervals (6-8). Thus, fNIRS has the potential to be used as an effective tool for measuring changes in bladder sensation in the cortex.
However, accurate fNIRS measurements can be difficult due to the sensitive nature of the technology. Furthermore, several studies highlighting fNIRS findings in patients with overactive bladder (OAB) or other forms of voiding dysfunction, while highly innovative, have lacked sufficient methodologic details to allow for reproducibility (8-11). Specifically, these early investigations do not clearly delineate how to account for head motion and other artifacts that can make the separation of signal from noise extremely challenging.
Therefore, the purpose of the current investigation was to provide a rigorous methodologic template that can ultimately be used for comparative studies of fNIRS in OAB and other forms of lower urinary tract dysfunction. We present this article in accordance with the MDAR reporting checklist (available at https://tau.amegroups.com/article/view/10.21037/tau-23-275/rc).
Methods
Participants
This study was conducted in accordance with the Declaration of Helsinki (as revised in 2013). The study was approved by the institutional review board at the Virginia Commonwealth University School of Medicine (No. HM20000453). Participants of both male and female biological sex and with no known urinary/bladder conditions were recruited. All participants were at least 21 years old, healthy enough to undergo testing, and had no cognitive impairment. All participants had normal sensation of their bladder filling with no evidence of neurologic injury or disease that could affect the lower urinary tract. Participants were screened with the validated International Consultation on Incontinence Questionnaire (ICIq)-OAB (12), and included only if the urgency score (question 5A: Do you have to rush to the bathroom?) was 0–1 (0–4 scale). Age, race, biological sex, body mass index (BMI) and average daily fluid intake (using a 3-day void diary) were recorded. All participants provided written consent prior to participation.
Hydration protocol
Following our previously published methods (6,13-17). To establish a study baseline, enrolled participants voided prior to initiation of testing and post-void residual volumes were determined with ultrasound. Participants were trained to use our validated Sensation Meter (13-17), which allows recording real-time patient reported bladder sensation through a touch screen tablet (0–100%) with 100% defined as maximum sensory capacity. The Sensation Meter also allows for recording of sensory thresholds (first sensation, first desire, strong desire) (18). Due to the consistency of participants reporting “first desire”, this terminology was used to separate periods of low (time before first desire) vs. high (time after first desire) bladder sensation and relate sensation changes to fNIRS signals. To minimize motion artifacts, participants were instructed to verbally report changing sensation values rather than directly using the tablet interface, which would likely involve a change in head tilt. Study time “0” was defined as the point when the study team started the Sensation Meter. At this point, participants were instructed to drink two liters of room temperature Gatorade G2® (PepsiCo, New York, USA) at an accelerated but comfortable pace. Recording was stopped at sensory capacity (100%) when patients were allowed to void in private bathroom with notation of voided and post-void residual volumes. Sensation data was recorded during filling over two complete fill-empty cycles over two separate visits.
fNIRS protocol
At time “0” participant head circumferences were measured, and they were each fitted with an appropriately sized (according to manufacturer’s guidelines) “Brite” head cap (Artinis Medical Systems, Elst, The Netherlands) with a 24-channel emitter/receiver optode array custom-designed using a neoprene sports cap and a punch tool. Optodes were positioned close to the forehead to maximize prefrontal cortex signal and minimize interference from hair using the manufacturer recommended frontal cortex optode template (24 long channels) (Figure 1). The head cap was aligned along the nasion-inion plane, and optodes were positioned according to anatomic landmarks using a Polhemis magnetic pencil (Artinis Medical Systems). During head cap fitting, we record fNIRS signal to check for signal quality and optode light saturation. Changes in O2Hb were recorded at 10 Hz over two complete bladder filling and emptying cycles. Optical density (OD) raw data were wirelessly collected through a built in Bluetooth module to the manufacturer’s software (Oxysoft, 3.2.72, Artinis Medical Systems). Portable batteries allow for mobility. However, the head cap battery has a practical time span of about 1.5 hours, so a power bank was required. Data was then exported to MATLAB (MathWorks, Natick, MA, USA) for further signal processing. To minimize motion artifact during data acquisition, participants were instructed to limit movements (particularly head motion). A high back chair and neck pillow were used to provide head stability (Figure 1), improve comfort, and decrease movement. Testing was completed during daylight hours in the same room (to standardize ambient light) with the blinds closed.
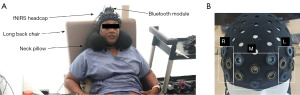
Signal processing
fNIRS data from each participant’s most steady overall fill [based on inertial measurement unit (IMU) showing the fill with the lowest amount of angular fluctuations and most constant IMU signal periods] were analyzed in three steps: (I) importing; (II) low pass filtering; and (III) mono-exponential curve fitting (Figure 2). Each step was applied to the 24 individual channels then grouped to create averaged left (nine channels), middle (six channels) and right (nine channels) cortical regions. In addition, due to wide variations in filling times between participants, the time for each low and high sensation phase was determined, and fNIRS data was interpolated to this reference time to standardize reporting.
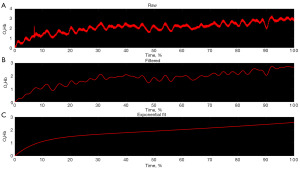
Electrical interference and motion artifact
A fully charged Brite battery lasts only ~1.5 hours, requiring an additional power source. However, connecting directly to a 120 Volt alternating current power outlet impacts signal quality which can be avoided through use of a power bank (Figure 3A), and the manufacturer provides a list of products which limit electrical interference. For consistency, the power bank was connected during all periods of data collection. To compensate for motion artifact, the Brite system is equipped with a built-in IMU which captures spatial orientation. The IMU data (10 Hz sampling) was used to identify motion artifact events, corresponding to rapid, high-amplitude fluctuations in fNIRS signal. A custom algorithm in MATLAB was coded to visually-identify segments of motion artifact (with a 3 s buffer on each end) and then splice the pre- and post-artifact tracings together. Splicing adjusted the post-artifact O2Hb value level to equal the pre-artifact level, and signal interpolation was used to compensate for any data loss. Finally, the signal was low pass filtered to remove heartbeat fluctuations (Figure 3B-3E).
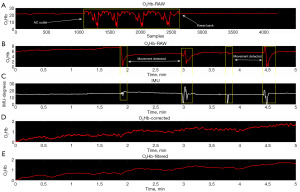
Statistical analysis
Data were compared using Student’s t-tests and MannWhitney U tests where P<0.05 was considered significant. For fNIRS data, the change in O2Hb for early and late sensation was compared.
Results
Eight participants completed the study including four biologic males and four biologic females. There were no differences (P>0.05) for all sociodemographic variables examined between male and female groups. Eighty-seven point five percent were White and 12.5% were Black. Table 1 presents individual participant data as well as median and interquartile range (IQR) for the female and male groups.
Table 1
Characteristics | Patient ID | ||||||||||
---|---|---|---|---|---|---|---|---|---|---|---|
Female | Male | ||||||||||
1 | 2 | 3 | 4 | Median [IQR] | 5 | 6 | 7 | 8 | Median [IQR] | ||
Age (years) | 24 | 24 | 28 | 68 | 26 [34] | 23 | 26 | 63 | 61 | 43.5 [38.75] | |
BMI (kg/m2) | 23.0 | 19.3 | 21.8 | 25.1 | 22.4 [4.6] | 25.1 | 25.1 | 28.6 | 23.7 | 25.1 [3.7] | |
Daily fluid intake (mL) | 1,892.5 | 3,785.0 | 1,774.2 | 946.2 | 1,833.3 [2,158.6] | 3,785.0 | 2,217.8 | 1,774.2 | 946.2 | 1,996.0 [2,239.9] | |
Baseline bladder volume (mL) | 3.3 | 20.0 | 39.0 | 6.3 | 13.2 [30.2] | 35.0 | 36.0 | 23.0 | 1.0 | 29.0 [29.3] | |
Total fill time (min) | 31.0 | 53.4 | 50.1 | 55.0 | 51.8 [18.8] | 43.0 | 65.0 | 56.0 | 66.0 | 60.5 [19.5] | |
Total voided volume (mL) | 700 | 300 | 750 | 300 | 500 [437.5] | 800 | 400 | 400 | 500 | 450 [325] |
IQR, interquartile range; BMI, body mass index.
Signal segmentation during low and high sensation
Initial attempts at signal processing focused on the entire fill (0% to 100% sensation). However, using an iterative process, signal partitioning in two phases (low sensation vs. high sensation) was adopted following the International Continence Society (ICS) recommendation to use three standardized verbal sensory thresholds to define areas of sensation: first sensation of filling, first desire to void, and strong desire to void. Therefore, the term “first desire”, the middle of the three terms, was used as a semi-objective way to separate low from high sensation (Figure 4). Using these combined methods, it was determined that the fNIRS relative change in O2Hb signal decreases with time during low sensation phases and increases during the high sensation phases, compared to their respective baselines (initial time of each section) (Figure 5, P<0.05) in all pre-frontal cortical regions.
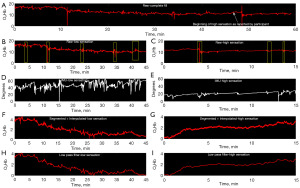
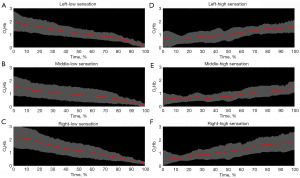
Discussion
This study demonstrates the ability to measure changes in prefrontal O2Hb through natural bladder filling in a physiologic seated position. fNIRS signals are sensitive and prone to signal interference; therefore, we propose a stepwise approach to improve collection and processing of the raw OD output. First, a stable, comfortable patient set-up is needed to minimize head movements. Second, choose a head cap configuration to optimize prefrontal optode placement, avoid hair, and maximize signal. Third, use the IMU channel to identify periods of motion artifact which can be excised. Finally, divide bladder filling data into low and high sensation segments which enables clear comparisons.
Traditionally bladder sensation is quantified during invasive urodynamic studies where the bladder is filled through a catheter in a retrograde fashion. To quantify sensation during filling, the subject is traditionally asked a series of provocative questions from which standard sensory thresholds including “first sensation of filling”, “first desire to void”, and “strong desire to void” as well as bladder capacity are recorded (18). Employing the methodology proposed in this stepwise protocol, bladder sensation is recorded in a continuous fashion using a Sensation Meter which has been validated in studies of natural bladder filling (13,15). The benefit of this approach includes real time, continuous sensation recording during fNIRS data acquisition, thereby increasing the potential to directly correlate changes in bladder sensation with changes in prefrontal cortical fNIRS O2Hb signals.
The majority of available literature for fNIRS related to bladder function presents results using a 3D brain model (9,19). The 3D brain model relies on grouped smoothing and averaging of the optical densities from optodes in the region of interest, and the Artinis Oxysoft processing averages neighboring optode signals. This averaging makes it more challenging to identify specific regions of data irregularity (especially for long periods of data collection) and doesn’t offer any correction for signal alterations due to irrelevant protocol events such as head movements, visual or auditory stimulating events (phone calls, etc.), and optode disconnection or misplacement which are all random events that we have witnessed during testing. To account for these factors, we presented the data in the form of O2Hb OD signals. This enables easy visual identification and removal of artifacts using our stepwise methodology.
Head movement is a significant limiting factor in brain fNIRS. Certain movements alter the hemodynamic characteristics of a tissue and therefore change the measured O2Hb OD signal. This is especially prevalent in for long protocols similar to our own, where it is challenging to keep participants steady for periods of bladder filling that can last more than an hour. Artifacts are also introduced with interference from ambient light, loss of contact with the scalp, and change in optode to emitter distance. Some investigators have attempted to overcome these limitations by completing experimentation in the supine position with a patient’s head resting and their eyes closed (10). Wiener and Kalman filtering techniques (20), have been proposed to remove motion in fNIRS signals, but these techniques use a trained data set with timed, single dimension movement. In contrast, our technique adjusts for random movement in three dimensions using an adaptive algorithm with concurrent real time accelerometer data to process brain fNIRS signals.
fMRI studies have identified several key brain regions and processing pathways that may be different in OAB (increased urgency) as compared to normal voiding. These regions include the anterior cingulate gyrus and prefrontal cortex (21-25), which is the key region of focus in our current fNIRS investigation. Although fMRI enables visualization of the entire brain during filling, there are multiple issues with the technology. Many studies involve artificial bladder filling through a urinary catheter, and, while some investigators have developed natural-filling fMRI protocols (21-24), most are completed in an unnatural supine position. A strength of this protocol is the ability to study subjects in an upright position and during natural bladder filling. The importance of the latter in new study protocols to evaluate lower urinary tract symptoms has been emphasized in the literature (26). In addition, fMRI has a much higher cost and requires dedicated equipment, space, and personnel expertise. Comparison of fMRI and fNIRS has been performed and demonstrated correlation in the detection of neuroexcitation in the anterior cortex (27-29). Thus, fNIRS may serve as an effective tool for measuring bladder sensation in the cortical brain. A pilot study of fNIRS and fMRI in six patients with OAB found agreement on activated cortical areas during urinary urgency (30). Further investigation with direct comparisons between fNIRS and fMRI is needed to continue to improve adoption of fNIRS.
Limitations of the current study include the small sample size and testing only on normal participants (without OAB or other forms of lower urinary tract dysfunction). In addition, our methodology requires post-hoc visual identification of IMU-detected motions and cannot currently be performed in real time. Furthermore, brain fNIRS is limited by depth of signal penetration and can only examine cortical brain regions. In addition, although each participant completed four fill-empty cycles (over two separate visits), we only analyzed one fill per participant with the best IMU data. To overcome some of these limitations, we are currently employing periods of required “stillness” during fNIRS studies and are developing an automated approach to signal processing.
Conclusions
fNIRS is a non-invasive, relatively inexpensive tool that can be used to provide in-vivo measurement of cortical O2Hb and compared during periods of low and high bladder sensation. In this study, we describe the development of a stepwise approach for data acquisition and signal processing based on optimized patient positioning, prefrontal optode placement, and visual identification of IMU-detected regions of motion. The methodological framework provided in this fNIRS investigation may present a reproducible technique for future researchers investigating brain-bladder signaling in OAB and other forms of lower urinary tract dysfunction.
Acknowledgments
Funding: This study was funded by National Institute of Health (No. R21DK128649 to JES, APK, and LS), Virginia Commonwealth University Presidential Research Quest Fund (No. 295166 to JES and APK), and National Institute of Health Building Interdisciplinary Research Careers in Women’s Health (No. K12HD108269 to LSB).
Footnote
Reporting Checklist: The authors have completed the MDAR reporting checklist. Available at https://tau.amegroups.com/article/view/10.21037/tau-23-275/rc
Data Sharing Statement: Available at https://tau.amegroups.com/article/view/10.21037/tau-23-275/dss
Peer Review File: Available at https://tau.amegroups.com/article/view/10.21037/tau-23-275/prf
Conflicts of Interest: All authors have completed the ICMJE uniform disclosure form (available at https://tau.amegroups.com/article/view/10.21037/tau-23-275/coif). LSB is funded by National Institute of Health Building Interdisciplinary Research Careers in Women’s Health (No. K12HD108269). JES, APK, and LS are funded by National Institute of Health (No. R21DK128649). JES and APK are funded by Virginia Commonwealth University Presidential Research Quest Fund (No. 295166). The other authors have no conflicts of interest to declare.
Ethical Statement: The authors are accountable for all aspects of the work in ensuring that questions related to the accuracy or integrity of any part of the work are appropriately investigated and resolved. This study was conducted in accordance with the Declaration of Helsinki (as revised in 2013). The study was approved by the institutional review board at the Virginia Commonwealth University School of Medicine (No. HM20000453). All participants provided written consent prior to participation.
Open Access Statement: This is an Open Access article distributed in accordance with the Creative Commons Attribution-NonCommercial-NoDerivs 4.0 International License (CC BY-NC-ND 4.0), which permits the non-commercial replication and distribution of the article with the strict proviso that no changes or edits are made and the original work is properly cited (including links to both the formal publication through the relevant DOI and the license). See: https://creativecommons.org/licenses/by-nc-nd/4.0/.
References
- Woessner H, Vibhute P, Barrett K. Acute loss of bladder control in a stroke of the frontal cortex. Neurohospitalist 2012;2:129-31. [Crossref] [PubMed]
- Arya NG, Weissbart SJ. Central control of micturition in women: Brain-bladder pathways in continence and urgency urinary incontinence. Clin Anat 2017;30:373-84. [Crossref] [PubMed]
- de Groat WC, Griffiths D, Yoshimura N. Neural control of the lower urinary tract. Compr Physiol 2015;5:327-96. [PubMed]
- Strangman G, Boas DA, Sutton JP. Non-invasive neuroimaging using near-infrared light. Biol Psychiatry 2002;52:679-93. [Crossref] [PubMed]
- Lloyd-Fox S, Blasi A, Elwell CE. Illuminating the developing brain: the past, present and future of functional near infrared spectroscopy. Neurosci Biobehav Rev 2010;34:269-84. [Crossref] [PubMed]
- Macnab AJ, Stothers L, Speich J, et al. Evaluation of cortical neuroexcitation in urinary urgency using simultaneous near infrared spectroscopy of the brain and bladder with quantification of sensation. SPIE 2019;11028:1102839. [Crossref]
- Matsumoto S, Ishikawa A, Matsumoto S, et al. Brain response provoked by different bladder volumes: a near infrared spectroscopy study. Neurourol Urodyn 2011;30:529-35. [Crossref] [PubMed]
- Sakakibara R, Tsunoyama K, Takahashi O, et al. Real-time measurement of oxyhemoglobin concentration changes in the frontal micturition area: an fNIRS study. Neurourol Urodyn 2010;29:757-64. [Crossref] [PubMed]
- Sakakibara R, Tateno F, Yano M, et al. Fesoterodine normalizes the brain function in overactive bladder patients due to central nervous system lesion: A real-time measure of oxyhemoglobin concentration changes during urodynamics. Int J Urol 2019;26:1014-5. [Crossref] [PubMed]
- Pang D, Liao L. Abnormal functional connectivity within the prefrontal cortex in interstitial cystitis/bladder pain syndrome (IC/BPS): A pilot study using resting state functional near-infrared spectroscopy (rs-fNIRS). Neurourol Urodyn 2021;40:1634-42. [Crossref] [PubMed]
- Sakakibara Tateno F. Tolterodine activates the prefrontal cortex during bladder filling in OAB patients: a real-time NIRS-urodynamics study. Neurourol Urodyn 2014;33:1110-5. [Crossref] [PubMed]
- Abrams P, Avery K, Gardener N, et al. The International Consultation on Incontinence Modular Questionnaire: www.iciq.net. J Urol 2006;175:1063-6; discussion 1066.
- Nagle AS, Speich JE, De Wachter SG, et al. Non-invasive characterization of real-time bladder sensation using accelerated hydration and a novel sensation meter: An initial experience. Neurourol Urodyn 2017;36:1417-26. [Crossref] [PubMed]
- Naimi HA, Nagle AS, Vinod NN, et al. An innovative, non-invasive sensation meter allows for a more comprehensive understanding of bladder sensation events: A prospective study in participants with normal bladder function. Neurourol Urodyn 2019;38:208-14. [Crossref] [PubMed]
- Sheen D, Kolli H, Nagle AS, et al. Validation of a real-time bladder sensation meter during oral hydration in healthy adults: Repeatability and effects of fill rate and ultrasound probe pressure. Low Urin Tract Symptoms 2019;11:224-31. [Crossref] [PubMed]
- Sebastian B, Swavely NR, Sethi D, et al. Comparison of Sensation Event Descriptors in Participants with Overactive and Normal Bladders during Non-Invasive Hydration Studies. Arch Nephrol Urol Stud 2021;1:03.
- Kolli H, Nagle AS, Sheen D, et al. Influence of visual and auditory cues about bladder volume on real-time filling sensation in healthy volunteers. Am J Clin Exp Urol 2022;10:44-51. [PubMed]
- Abrams P, Cardozo L, Fall M, et al. The standardisation of terminology in lower urinary tract function: report from the standardisation sub-committee of the International Continence Society. Urology 2003;61:37-49. [Crossref] [PubMed]
- Pang D, Liao L, Chen G, et al. Sacral Neuromodulation Improves Abnormal Prefrontal Brain Activity in Patients with Overactive Bladder: A Possible Central Mechanism. J Urol 2022;207:1256-67. [Crossref] [PubMed]
- Izzetoglu M, Chitrapu P, Bunce S, et al. Motion artifact cancellation in NIR spectroscopy using discrete Kalman filtering. Biomed Eng Online 2010;9:16. [Crossref] [PubMed]
- Griffiths D, Tadic SD, Schaefer W, et al. Cerebral control of the bladder in normal and urge-incontinent women. Neuroimage 2007;37:1-7. [Crossref] [PubMed]
- Tadic SD, Griffiths D, Schaefer W, et al. Abnormal connections in the supraspinal bladder control network in women with urge urinary incontinence. Neuroimage 2008;39:1647-53. [Crossref] [PubMed]
- Griffiths D, Derbyshire S, Stenger A, et al. Brain control of normal and overactive bladder. J Urol 2005;174:1862-7. [Crossref] [PubMed]
- Shy M, Fung S, Boone TB, et al. Functional magnetic resonance imaging during urodynamic testing identifies brain structures initiating micturition. J Urol 2014;192:1149-54. [Crossref] [PubMed]
- Bou Kheir G, Verbakel I, Hervé F, et al. OAB supraspinal control network, transition with age, and effect of treatment: A systematic review. Neurourol Urodyn 2022;41:1224-39. [Crossref] [PubMed]
- Lai HH, Naliboff B, Liu AB, et al. The LURN Research Network Neuroimaging and Sensory Testing (NIST) Study: Design, protocols, and operations. Contemp Clin Trials 2018;74:76-87. [Crossref] [PubMed]
- Cui X, Bray S, Bryant DM, et al. A quantitative comparison of NIRS and fMRI across multiple cognitive tasks. Neuroimage 2011;54:2808-21. [Crossref] [PubMed]
- Irani F, Platek SM, Bunce S, et al. Functional near infrared spectroscopy (fNIRS): an emerging neuroimaging technology with important applications for the study of brain disorders. Clin Neuropsychol 2007;21:9-37. [Crossref] [PubMed]
- Ferrari M, Quaresima V. A brief review on the history of human functional near-infrared spectroscopy (fNIRS) development and fields of application. Neuroimage 2012;63:921-35. [Crossref] [PubMed]
- Clarkson B, Huppert T, Beluk N, et al. Do near infrared spectroscopy (NIRS) and functional mri agree when investigating brain control of the lower urinary tract? Neurourology and Urodynamics 2012;31:abstr 93.