A narrative review on the use of near-infrared spectroscopy to monitor bladder volume and in vitro validation approaches
Introduction
Neurogenic bladder (NB) is a dysfunction of the nervous system resulting in involuntary control of the bladder and in some cases, loss of bladder sensation. Underlying causes of NB can be due to various mechanisms, such as stroke, tumors, birth defects, and other diseases which may affect the spinal cord (1). Management of NB often involves clean intermittent self-catheterization (CISC) at certain intervals throughout the day (2). However, strict adherence to a CISC schedule can negatively impact the quality of life for patients. Some patients attempt to estimate their own urine production cycle by carefully controlling the amount of water and nutrients they intake. However, timing catheterization becomes particularly challenging when diagnosed with the loss of bladder sensation or engaging in activities outdoors. Intaking diuretic foods and liquids, such as by dining at restaurants, can affect body osmolarity, altering the urine production rate, and ultimately render personal estimations inaccurate. At best, patients can increase their CISC frequency. However, this requires convenient access to restrooms, which may be not always be possible in public or remote settings (3). As a result, bladder overfilling may occur, which could lead to leakage, infections, or severe complications (4).
Due to these as well as other factors, such as psychological barriers, many patients tend to avoid extended social or work activities, leading to more secluded lifestyles and a generally lower quality of life. Proper bladder management is integral to quality of life for NB patients, thus non-invasive bladder volume monitoring devices that are reliable and portable are needed (5).
To address the need for a bladder volume monitoring device, several wearables have been proposed using a variety of techniques such as ultrasound (US), electrical bioimpedance (BIM), and near-infrared spectroscopy (NIRS). US-based bladder monitoring devices exploit the properties of ultrasonic sound waves to probe the bladder. By maintaining the bladder in the field of view of the device, the bladder dimensions, and thus an estimation of bladder volume, can be calculated (6). Currently there are several commercialized, non-invasive wearable US devices like SENS-U (7) and DFree (8) to monitor the bladder volume. However, these devices have documented challenges, such as sensitivity to device positioning, which can lead to invalid readings (9). SENS-U has only been validated for use with children. Thus, at the time of writing, DFree remains the only commercialized ultrasonic-based device appropriate for adults. However, the adoption of wearable US devices may be hindered by the need for coupling gel, which may be inconvenient for patients to wear and maintain continuously.
Another promising bladder sensing device is the use of BIM. BIM utilizes high-frequency electrical currents sent through electrodes pairs attached to the skin at several locations. Recent work in this research space demonstrated that by analysis of the electrical conductivity, tomographic data may be able to track with bladder volume (10). However, BIM requires a network of electrodes attached to the abdomen, which is not yet practical as a consumer wearable device. More work is also needed to reduce sensitivity to motion artifacts and noise produced by muscle contractions.
Lastly, NIRS is an emerging candidate for non-invasive monitoring in various applications. NIRS is an optical technique that uses light in the near-infrared wavelength range, approximately 600–1,000 nm (11). In this wavelength region, tissue has relatively low absorption, allowing light to penetrate deeper into tissue and interact with subsurface structures. Physiological information such as hemoglobin, water, and fat can also be calculated (12,13). In addition, NIRS has been widely adopted for studying brain hemodynamics (14,15). In recent years, due to NIRS showing potential to be incorporated into a compact and wearable device, several studies have been performed that utilized NIRS for bladder monitoring. Early works with NIRS in bladder monitoring looked at hemoglobin fluctuations during urodynamic studies (UDS) of NB patients (16-18). As for monitoring the bladder volume, there are only a few studies that have been conducted.
In this review, a comprehensive literature overview of usage and validation of NIRS devices for bladder volume monitoring was discussed. Specifically, validation of such devices using bladder simulating phantoms was the focus of this paper. As a foundational step, robust in vitro validation should be established in this area first as progress is made towards clinical validation. In addition, we suggested a potential in-vitro validation approach by combining studies performed in the field of US and NIRS. We present this article in accordance with the Narrative Review reporting checklist (available at https://tau.amegroups.com/article/view/10.21037/tau-23-297/rc).
Methods
A literature search was conducted in February 2023 using PubMed and Google Scholar as the primary search engines. Keywords such as, “NIRS and bladder volume”, “NIRS and bladder monitoring”, “bladder filling and NIRS” was used. Any publications showing the use and in-vitro validation of near infrared spectroscopy for bladder volume monitoring was considered. A more detailed list of the search criteria is shown in Table 1.
Table 1
Items | Specification |
---|---|
Date of search | 02/02/2023 |
Databases and other sources searched | PubMed, Google Scholar |
Search terms used (including MeSH and free text search terms and filters) | “NIRS and bladder volume”, “NIRS and bladder monitoring”, “bladder filling and NIRS”, “NIRS bladder volume monitoring with AI”, or “non-invasive bladder volume monitoring”” |
Timeframe | 01/01/2000–02/02/2023 |
Inclusion and exclusion criteria | Inclusion: |
• Published articles | |
• Any articles supporting the idea of a NIRS-based bladder monitoring device | |
Exclusion: | |
• Any NIRS-based device articles that were relevant but was not officially published in a journal | |
• Any articles not written in English | |
Number of articles found related to bladder volume monitoring with near-infrared spectroscopy and excluded articles | Number of articles found: 4 |
Number of articles excluded: 2 | |
Reason: both articles were dissertations and not officially published | |
Number of supporting articles: 6 | |
Selection process | Authors K.J.T. and J.H.L. conducted the selection process and consensus was made by all authors |
MeSH, Medical Subject Headings; NIRS, near-infrared spectroscopy; AI, artificial intelligence.
Results
Overview of bladder volume monitoring using light
As the result of our literature search, only two studies were found highlighting the usage of NIRS to monitor the bladder volume. Due to the novelty of this application, no clinical trial has been reported yet. The devices presented in these studies have only performed feasibility testing with variable validation methods (Table 2). In this section, the two studies which used NIRS for bladder volume monitoring will be discussed in-depth regarding their in-vitro experimental setup, their validation method, their results and any limitations that were present.
Table 2
Device name/authors | Description | Measured units | In-vitro validation method | Validation criteria |
---|---|---|---|---|
Molavi et al. (19) | A wearable device consisting of one pair of light source (950 nm) and detector. The data was transferred back to a PC for analysis | Attenuation (optical density) | A plastic bottle was filled with intralipid with abdomen-like scattering properties along with a non-specified dye for the absorption. They then attached their device with tape to the bottle for analysis. Water was injected into a submerged balloon to serve as a bladder substitute. Balloon inflation dynamics were measured | Balloon inflation dynamics |
Fong et al. (20) | A wearable device consisting of 7 pairs of LEDs and detectors with a 4 cm distance between the source and detector pair. The acquired data is also calculated with the help of AI | Intensity (Volts) | Ground pork was layered in a large flat plastic container to serve as an abdomen substitute. Another smaller container filled with water was placed on the ground pork. They then scanned their device across the phantom, measuring through the larger container. Contrast relative to background was calculated | Contrast |
NIRS, near-infrared spectroscopy; LEDs, light emitting diodes; AI, artificial intelligence.
In 2014, Molavi et al. were the first group of researchers that published a paper presenting a NIRS-based wireless bladder volume monitoring device (19). To validate their custom-made device, they conducted an in-vitro and in-vivo experiment. The in-vitro experiment comprised of a custom phantom composed of 20% intralipid and a non-specified ink to obtain optical parameters similar to abdominal tissue (Figure 1). The results showed promising evidence that NIRS-based devices could potentially be sensitive to bladder filling by measuring through the abdomen. In addition, they presented in-vivo data, that showed sensitivity to bladder filling but was limited to a single healthy subject.
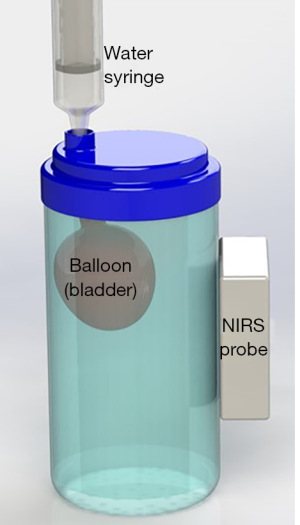
In 2018, Fong et al. proposed a NIRS-based device with 7 pairs of light emitters and photodetectors (20). They first performed a Monte Carlo simulation, showing a change in detected photons when the bladder was full compared to an empty bladder. To make the phantom for their in-vitro test, ground pork was used to mimic abdomen tissue and placed inside a plastic container. While another plastic container of water was placed in the center of the ground pork to represent liquid in the bladder (Figure 2). By sweeping their device over the phantom, a change in light attenuation was detected, showing contrast between the background tissue and the embedded water. Lastly, an in-vivo experiment was performed on a single healthy human subject. Although the results of the in-vivo experiment did show some feasibility by showing a decrease in light intensity while the bladder is filling, it was limited to a single subject without cross-validation by another method.
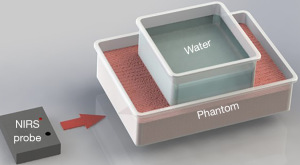
While each of these research groups provided meaningful results focusing on the feasibility of using NIRS for bladder monitoring, it was observed that each of them had their own method on validating their device. Currently to our knowledge, there is no standard in-vitro validation method to evaluate the accuracy of NIRS devices for bladder volume monitoring.
How can optical bladder monitoring be validated in an in-vitro setting?
In the optical spectroscopy field, including NIRS, strong validation procedures typically include a robust tissue-simulating phantom with opportunities for cross-validation with a gold-standard method (21). Among the reviewed literature, one common limitation observed was the absence of a suitable model that contained all the following characteristics: (I) optically manipulated to mimic the absorption and scattering properties of abdominal tissue, (II) can be inflated or deflated to mimic a dynamic bladder, (III) can be validated by another established technology, such as US, (IV) is non-degradable so that results are reproducible over time. To meet all of these criteria, one solution we propose is the construction of a bladder-simulating phantom using flexible ballistics gel and an embedded balloon to mimic the bladder.
Many types of materials have been reported for the construction of tissue simulating phantoms (Table 3). Collagen gelatin and agar are flexible hydrated matrices which can be appropriate as tissue-simulating phantoms (21). However, like liquid materials such as intralipid or emulsion, they are also susceptible to bacterial growth and degrade over time within room temperature. In addition, degradation by contamination can occur. Lastly, silicone has historically been a staple material for optical phantoms (22), but has limited contrast when used with US (23). In comparison to these materials, a bladder phantom incorporating ballistics gel could be a potential material in the validation of NIRS based bladder monitoring devices.
Table 3
Phantom material | Key features | Shelf life | Compatibility |
---|---|---|---|
Silicone | PDMS, non-degradable, semi-flexible | Years | NIRS |
Ballistics gel | Synthetic polymer, non-degradable, flexible, tissue-like mechanical properties | Years | NIRS, ultrasound |
Agar | Algae derived, degradable, flexible | Weeks | NIRS, ultrasound |
Gelatin | Pig skin derived, degradable, flexible | Weeks | NIRS, ultrasound |
Liquid | Usually intralipid, degradable | Days | NIRS, ultrasound |
Ex-vivo tissue | Usually porcine or bovine tissue, degradable, flexible | Hours | NIRS, ultrasound |
PDMS, polydimethylsiloxane; NIRS, near-infrared spectroscopy.
Discussion
The potential of using ballistics gel for a bladder phantom
US compatibility of ballistics gel has already been demonstrated as many researchers have used ballistics gel for US training applications (24,25). Made of synthetic polymers, ballistics gelatin is also shelf-stable (26). In addition, the mechanical properties of ballistics gel were found to be similar to human tissue, making it durable, elastic, and re-usable. In terms of flexibility, a hollow mold of ballistics gelatin could potentially be inflated and deflated to mimic the bladder, but more research is needed to study the compliance of the material. While there is no record of using ballistics gel as part of a phantom for NIRS applications, incorporating the ballistic gel as shown in Figure 3, could be useful for validating NIRS-based bladder monitoring devices against US. In our proposed phantom, one side could be customized for optical performance, while the other could be used for US. While it could be assumed that the amount of water injected into the phantom is accurate, we think it is still important for the phantom to be compatible with the real-world Gold-standard. This could be useful for comparison between technologies, such as Bland Altman analysis. By allowing the phantom to be compatible with both US and NIRS, the accuracy as well as margin of error of both methods can be compared. We hope that by proposing the use of ballistics gelatin as the basis material for a bladder phantom, validation for NIRS bladder monitoring devices can be more standardized, allowing researchers to better compare approaches and advance the technology.
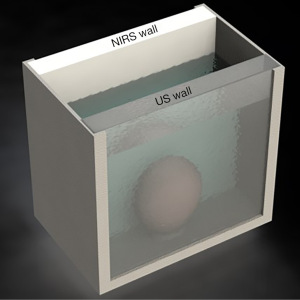
Utilizing artificial intelligence (AI) in NIRS-based bladder volume monitoring devices
There was one article that appeared this year building upon the research done by Fong et al. incorporating AI with NIRS. Pascal Fechner et al. reported a machine learning (ML) and deep learning (DL) models and the feasibility of applying it into NIRS technology to predict bladder volume (27). While they did mention utilizing a NIRS-based prototype device to test their ML and DL models effectiveness, the device itself was not highlighted. In addition, since they did not perform any in-vitro tests, we decided to omit the article in the results section. However, their research focusing on the idea of using AI in helping NIRS-based bladder monitoring devices to accurately monitor the volume of the bladder should be highlighted. By incorporating AI with NIRS, it could possibly be a potential route in creating a commercialized NIRS device, but more research incorporating them together needs to be conducted. Therefore, it would be very interesting to see how their best ML and DL models would behave against a suitable in-vitro method and how the results from the in-vitro studies can be translated into in-vivo results.
Conclusions
NIRS-based devices are a relatively new innovation in the bladder monitoring space. This type of device has the potential to increase the quality of life of patients with NB allowing them to properly manage their bladder and avoid severe complications. While there are a few studies which have demonstrated feasibility, each of them also has limitations during the validation step. By outlining a more standardized in-vitro validation model based on ballistics gelatin, we hope to facilitate development in this field towards a more accurate and robust NIRS-based bladder monitoring device.
Acknowledgments
Funding: This research was supported by
Footnote
Reporting Checklist: The authors have completed the Narrative Review reporting checklist. Available at https://tau.amegroups.com/article/view/10.21037/tau-23-297/rc
Peer Review File: Available at https://tau.amegroups.com/article/view/10.21037/tau-23-297/prf
Conflicts of Interest: All authors have completed the ICMJE uniform disclosure form (available at https://tau.amegroups.com/article/view/10.21037/tau-23-297/coif). S.K. is a Chief Technology Officer of Medithings Co., Ltd. The other authors have no conflicts of interest to declare.
Ethical Statement: The authors are accountable for all aspects of the work in ensuring that questions related to the accuracy or integrity of any part of the work are appropriately investigated and resolved.
Open Access Statement: This is an Open Access article distributed in accordance with the Creative Commons Attribution-NonCommercial-NoDerivs 4.0 International License (CC BY-NC-ND 4.0), which permits the non-commercial replication and distribution of the article with the strict proviso that no changes or edits are made and the original work is properly cited (including links to both the formal publication through the relevant DOI and the license). See: https://creativecommons.org/licenses/by-nc-nd/4.0/.
References
- Samson G, Cardenas DD. Neurogenic bladder in spinal cord injury. Phys Med Rehabil Clin N Am 2007;18:255-74. vi. [Crossref] [PubMed]
- Broom R, Fiander N, Measures A. Trust Guideline for the Management of Teaching Clean Intermittent Self-Catheterization (CISC). Norfolk Norwish Univ Hosp. 2020.
- Seth JH, Haslam C, Panicker JN. Ensuring patient adherence to clean intermittent self-catheterization. Patient Prefer Adherence 2014;8:191-8. [PubMed]
- Tate DG, Wheeler T, Lane GI, et al. Recommendations for evaluation of neurogenic bladder and bowel dysfunction after spinal cord injury and/or disease. J Spinal Cord Med 2020;43:141-64. [Crossref] [PubMed]
- Wheeler TL. Translating promising strategies for bowel and bladder management in spinal cord injury. Exp Neurol 2018;306:169-76. [Crossref] [PubMed]
- Ghani KR, Pilcher J, Rowland D, et al. Portable ultrasonography and bladder volume accuracy--a comparative study using three-dimensional ultrasonography. Urology 2008;72:24-8. [Crossref] [PubMed]
- SENS-U Website [Internet]. Available online: https://novioscan.com/
- DFree [Internet]. Available online: https://www.dfreeus.biz/
- Hafid A, Difallah S, Alves C, et al. State of the Art of Non-Invasive Technologies for Bladder Monitoring: A Scoping Review. Sensors (Basel) 2023;23:2758. [Crossref] [PubMed]
- Leonhäuser D, Castelar C, Schlebusch T, et al. Evaluation of electrical impedance tomography for determination of urinary bladder volume: comparison with standard ultrasound methods in healthy volunteers. Biomed Eng Online 2018;17:95. [Crossref] [PubMed]
- Ferrari M, Mottola L, Quaresima V. Principles, techniques, and limitations of near infrared spectroscopy. Can J Appl Physiol 2004;29:463-87. [Crossref] [PubMed]
- Shah N, Cerussi A, Eker C, et al. Noninvasive functional optical spectroscopy of human breast tissue. Proc Natl Acad Sci U S A 2001;98:4420-5. [Crossref] [PubMed]
- Ohmae E, Yoshizawa N, Yoshimoto K, et al. Comparison of Lipid and Water Contents by Time-domain Diffuse Optical Spectroscopy and Dual-energy Computed Tomography in Breast Cancer Patients. Appl Sci 2019;9:1482. [Crossref]
- Holper L, Scholkmann F, Wolf M. Between-brain connectivity during imitation measured by fNIRS. Neuroimage 2012;63:212-22. [Crossref] [PubMed]
- Pierro ML, Hallacoglu B, Sassaroli A, et al. Validation of a novel hemodynamic model for coherent hemodynamics spectroscopy (CHS) and functional brain studies with fNIRS and fMRI. Neuroimage 2014;85:222-33. [Crossref] [PubMed]
- Macnab AJ, Stothers L. Development of a near-infrared spectroscopy instrument for applications in urology. Can J Urol 2008;15:4233-40. [PubMed]
- Macnab AJ, Gagnon RE, Stothers L. Clinical NIRS of the urinary bladder – A demonstration case report. Spectroscopy 2005;19:207-12. [Crossref]
- Macnab AJ, Stothers L. Near-infrared spectroscopy: validation of bladder-outlet obstruction assessment using non-invasive parameters. Can J Urol 2008;15:4241-8. [PubMed]
- Molavi B, Shadgan B, Macnab AJ, et al. Noninvasive Optical Monitoring of Bladder Filling to Capacity Using a Wireless Near Infrared Spectroscopy Device. IEEE Trans Biomed Circuits Syst 2014;8:325-33. [Crossref] [PubMed]
- Fong DD, Yu X, Mao J, et al. Restoring the sense of bladder fullness for spinal cord injury patients. Smart Health 2018;9–10:12-22. [Crossref]
- Pogue BW, Patterson MS. Review of tissue simulating phantoms for optical spectroscopy, imaging and dosimetry. J Biomed Opt 2006;11:041102. [Crossref] [PubMed]
- Cerussi AE, Warren R, Hill B, et al. Tissue phantoms in multicenter clinical trials for diffuse optical technologies. Biomed Opt Express 2012;3:966-71. [Crossref] [PubMed]
- Zell K, Sperl JI, Vogel MW, et al. Acoustical properties of selected tissue phantom materials for ultrasound imaging. Phys Med Biol 2007;52:N475-84.
- Morrow DS, Broder J. Cost-effective, Reusable, Leak-resistant Ultrasound-guided Vascular Access Trainer. J Emerg Med 2015;49:313-7. [Crossref] [PubMed]
- Alves N, Kim A, Tan J, et al. Cardiac Tissue-Mimicking Ballistic Gel Phantom for Ultrasound Imaging in Clinical and Research Applications. Ultrasound Med Biol 2020;46:2057-69. [Crossref] [PubMed]
- Hummic Medical Ballistics Gel [Internet]. Available online: https://humimic.com
- Fechner P, König F, Kratsch W, et al. Near-Infrared Spectroscopy for Bladder Monitoring: A Machine Learning Approach. ACM Trans Manag Inf Syst 2023;14:1-23. [Crossref]