CCL5 promotes the proliferation and metastasis of bladder cancer via the JAK2/STAT3 signaling pathway
Highlight box
Key findings
• C-C chemokine ligand 5 (CCL5) promotes proliferation and metastasis of bladder cancer (BC) cells in vitro and in vivo.
What is known and what is new?
• CCL5 are expressed abnormally and activated in solid tumors and hematological malignancies.
• This study showed that CCL5 promotes proliferative, migratory, and tumor-growing BC cells in vitro and tumor metastasizing BC cells in vivo. Moreover, we found that the tumor-promotive role of CCL5 is dependent on activation of the JAK2/STAT3 signaling pathway.
What is the implication, and what should change now?
• CCL5 may play an oncogenic role in BC and may also serve as a potential diagnostic and prognostic biomarker.
Introduction
Urothelial carcinoma is one of the most common cancers of the urinary system with an incidence of nearly 550,000 cases annually and is attributable to around 200,000 deaths annually (1-3). There are two types of bladder cancer (BC) based on the depth of tumor invasion: non-muscle invasive tumors, which account for approximately 70–80% of cases, and muscle-invasive tumors, which make up 20–30% of cases. Non-muscle invasive bladder cancer (NMIBC) is a type of BC that forms in the innermost lining of the bladder, but does not invade the deeper layers of the bladder wall. Although the primary tumor can be surgically resected, rates of recurrence and metastasis remain high, especially in advanced disease, leading to a poor prognosis and associated treatment challenges (4). In the past few decades, despite the emergence of numerous novel treatments for advanced BC, clinical benefits have remained limited (5-9). Therefore, further clarification regarding specific mechanisms of BC metastasis and recurrence and exploration of more effective therapeutic targets remains an important objective.
The C-C chemokine ligand 5 (CCL5) is a member of the C-C-motif chemokine family, which also includes macrophage inflammatory protein 1α (MIP-1α) and macrophage inflammatory protein 1β (MIP-1β) (10-12). With high affinity, CCL5 mainly binds to its receptor C-C chemokine receptor type 5 (CCR5), as well as CCR1, CCR3, CCR4, CD44, and GPR75 (13-15). CCL5 is also involved in B cell proliferation via activation of the nuclear factor κ-light chain enhancer (NF-κB) (16). Expression of this protein is found in T lymphocytes, macrophages, platelets, synovial fibroblasts, tubule epithelial cells, and tumor cells (17). According to recent studies, CCL5 promotes tumor progression by enhancing tumor metastasis (18) and remodeling the extracellular matrix, which results in supporting tumor stem cell expansion (19), causing tumor cells drug-resistant (20), reducing the cytotoxicity of DNA damage factors, decompressing cellular metabolic reprogramming (21), increasing angiogenesis, mobilizing immune cells (22), and inducing macrophage polarization to suppress immune response (23). However, the underlying mechanism of CCL5 in BC remains unclear.
In this study, we explored the role of CCL5 in BC. The role of CCL5 in BC cell proliferation, migration, and tumor growth and metastasis was demonstrated in vitro and in vivo. Moreover, the tumor-promotive role of CCL5 appeared to be dependent on activation of the JAK2/STAT3 signaling pathway. We conclude that BC progression and metastasis may partially be driven by the CCL5 pathway, which offers a valuable potential therapeutic target or prognostic biomarker for BC. We present this article in accordance with the ARRIVE reporting checklist (available at https://tau.amegroups.com/article/view/10.21037/tau-23-540/rc).
Methods
Reagents and antibodies
The anti-GAPDH antibody (G8795), TRIzol (T9424) and polyvinylidene difluoride (PVDF) membrane (3010040001) were purchased from Sigma Aldrich (St. Louis, MO, USA). The anti-CCL5 antibody (ab9679) was purchased from Abcam (MA, USA). The anti-phospho-JAK1 (74129), anti-JAK1 (3332), anti-phospho-JAK2 (3771), anti-JAK2 (3230), anti-phospho-STAT3 (9145), and anti-STAT3 (9139) were purchased from CST (MA, USA). Transwell (3422) and Matrigel Matrix (356231) were purchased from Corning (NY, USA). ChamQ Universal SYBR qPCR Master Mix was purchased from Vazyme (Nanjing, China).
Cell culture
We purchased the human BC cell lines [T24(SCSP-536), 5637 (TCHu 1), and J82 (TCHu218)], and a normal human bladder cell line (SV-HUC-1) from Chinese Academy of Sciences, Shanghai Institutes for Biological Sciences (Shanghai, China). The cells were cultured in Dulbecco’s Modified Eagle Medium (DMEM) (Hyclone, Logan, UT, USA) supplemented with 10% fetal bovine serum (FBS) (Gibco, Billings, MT, USA) in the presence of 1.0% penicillin-streptomycin (Solarbio, Beijing, China), and were incubated under standard culture conditions (5% CO2, 37 ℃).
Stable CCL5 knockdown and knockout cell line establishment
To establish stable cell lines with CCL5 knocked-down or over-expression, short hairpin RNA (shRNA) or CCL5 coding sequence (CDS) containing constructs, along with psPAX and pMD2G plasmids, were co-transfected into 293T cells for RNA interference. After a 48-hour incubation period, the culture supernatant was collected and filtered using a 0.22-μm membrane. Subsequently, the virus liquid was concentrated via filtration through a 100-kD millipore filter and added to the cancer cell culture medium, allowing for virus infection to occur for 24 hours. The cells that expressed CCL5 CDS or shRNAs successfully were identified using puromycin treatment at a concentration of 5 μg/mL (MCE, NJ, USA).
RNA extraction and real-time polymerase chain reaction (RT-PCR) analysis
For the RT-PCR assay, a total of 1×107 cells were digested and harvested. The total RNA was extracted by TRIzol reagent (Thermo Fisher Scientific, Waltham, MA, USA). The ABI vii7 system (Applied Biosystems, Waltham, MA, USA) was used to conduct RT-PCR, and SYBR green was used to identify DNA in the sample. Glyceraldehyde 3-phosphate dehydrogenase (GAPDH) was chosen as the housekeeping gene in humans. Relative gene expression was normalized to GAPDH messenger RNA (mRNA) and analyzed using the comparative Ct method (ΔΔCt). Primers were synthesized as follows:
- CCL5: forward, 5'-GCTTTGCCTACATTGCCCGC-3'; reverse, 5'-GGCGGTTCTTTCGGGTGACA-3';
- GAPDH: forward, 5'-TGACTTCAACAGCGACACCCA-3'; reverse, 5'-CACCCTGTTGCTGTAGCCAAA-3'.
Western blotting analysis
For the western blotting assay, a total of 1×107 cells were digested and harvested. The total protein was extracted with RIPA lysis buffer (P0013C, Beyotime, Shanghai, China). Using 12% sodium dodecyl sulfate polyacrylamide gel electrophoresis (SDS-PAGE), 40 mg of protein extracts were separated, transferred to a PVDF membrane, and probed with anti-CCL5 (1:1,000; ab9679; Abcam), the anti-GAPDH antibody (1:5,000; Sigma Aldrich) overnight at 4 ℃. Following extensive washing with tris-buffered saline with Tween 20 (TBST), membranes were incubated with goat anti-mouse secondary antibodies (1:8,000; Sigma Aldrich) that were horseradish peroxidase (HRP)-labeled for 1 hour at room temperature. Using the ECL Plus detection system (GE Healthcare, Milwaukee, WI, USA), the proteins were visualized.
Immunofluorescence (IF) staining
Glass coverslips with cultured cells were subjected to three washes with phosphate-buffered saline (PBS), followed by fixation with 4% paraformaldehyde for a duration of 10 minutes. To enable permeabilization, the cells were treated with PBS containing 0.5% Triton X-100 for 10 minutes. Subsequently, the cells were blocked using 1% BSA for 1 hour, followed by overnight incubation with the specified antibody at a temperature of 4 ℃. Afterward, the cells were incubated with DAPI and goat anti-rabbit or mouse secondary antibodies (Alexa Flour 568 or 488, diluted at a ratio of 1:200) for 1 hour at room temperature. Images were captured using a Zeiss LSM 780 confocal microscope equipped with ZEN 2010 software (Carl Zeiss GmbH, Jena, Germany). For each group, a minimum of 50 cells were randomly selected and quantified. The area displaying positive signals for METTL3 intensity levels was measured using Image J (version 1.52p) and normalized against the area showing positive signals for DAPI intensity levels. The same intensity threshold was applied across different cell lines.
Cell proliferation assay
An examination of the proliferation of cells was carried out using Cell Counting Kit-8 (CCK-8, Dojindo, Japan). Each well of a 96-well plate was seeded with approximately 1,000 cells. At various intervals following cell seeding, the wells were replenished with fresh medium containing 10% CCK-8 substrate and incubated at 37 ℃ for 2 hours. The microplate reader was then utilized to determine the light absorbance value of the plate at 490 nm, and subsequently compute the cell proliferation rate.
Migration assay
An assessment of the migration of cells was conducted using a wound healing assay. After trypsinization and cell counting, 5×105 cells were seeded into each well of a 6-well plate, which had previously been marked with an evenly spaced horizontal line. Once the cells had reached confluence, perpendicular scratches were made across the marked line. Subsequently, PBS was used to remove any cellular debris, and the cells were then cultured in McCoy’s 5A medium with low serum at 37 ℃ and 5% CO2. The widths of the scratches at 0, 24, and 48 hours post-scratching were observed and documented using an inverted biological microscope (CX53, Olympus, Japan), with three fields of view captured at each time point.
Invasion assay
A transwell system with an 8-μm pore size (Corning) was employed for the experiment. The upper chamber of the transwell was seeded with 2×104 cells cultured in 500 μL of serum-free medium, while the bottom well was supplemented with 1 mL of complete culture medium containing 10% serum. Following a 48-hour incubation period in a dedicated incubator, the cells were fixed with 4% paraformaldehyde and subsequently stained using crystal violet solution. To eliminate any remaining cells that had not migrated, a cotton swab was used, and the remaining cells were then observed and imaged under a microscope.
Subcutaneous transplantation model study
A total of 20 female Balb/c nude mice [4–6 weeks old, purchased from Cavens Biogle (Suzhou) Model Animal Research Co., Ltd., Suzhou, China] were bred in aseptic conditions and maintained at a constant humidity and temperature (25–28 ℃) for later allocation to four groups. In 0.2-mL PBS, J82 BC cells (1×107 per mouse) from the indicated groups were injected subcutaneously into the right inguinal region of mice that were naked. A caliper was used to measure the size of tumors every 5 days, and to calculate the tumor volume using the formula: length × width2 ×0.5. A growth curve was constructed. A protocol was prepared before the study without registration. Animal experiments were performed under a project license granted by ethics committee of the Third Affiliated Hospital of Soochow University, in compliance with the institutional guidelines for the care and use of animals.
Statistical analysis
GraphPad Prism 9.0 (GraphPad Software Inc., San Diego, CA, USA) was used for all statistical analyses, and all experiments were repeated three times. The data in all figures were represented as a mean ± standard deviation. Comparisons between and among groups were performed using the Student’s t-test, one-way analysis of variance (ANOVA), and two-way ANOVA. Statistical significance was considered at P<0.05 (bilateral).
Results
CCL5 knockdown inhibited the proliferation, migration, and invasion in vitro
To explore the role of CCL5 in BC, we knocked down the level of CCL5 in BC cells. First, we analyzed the expression level of CCL5 in BC patients from the Gene Expression Omnibus (GEO) database (GSE13507). We found that the level of CCL5 expression was significantly lower in primary BC tissues and recurrent non-muscle invasive tumor tissues than that in normal bladder tissues (Figure 1A). We then evaluated the expression pattern of CCL5 in BC cell lines and found that the level of CCL5 mRNA was lower in J82 cells as compared to T24 cells (Figure 1B), which was further confirmed by western blot (Figure 1C). We then selected the T24 cell line and knocked down the level of CCL5 by small interfering RNA (siRNA). The knockdown efficiency of CLL5 were analyzed by RT-PCR (Figure 2A, P<0.01) and verified by western blot (Figure 2B, P<0.01). Interestingly, as shown in Figure 2C, the CCL5 knockdown significantly inhibited the cell proliferation ability, which was evaluated by CCK-8 assay (Figure 2C, P<0.05). Consistently, the migration and invasion abilities of BC cells were also impaired by CCL5 knockdown, as indicated by wound healing assay (Figure 2D, P<0.05) and transwell invasion assay (Figure 2E, P<0.05), respectively. Moreover, the results of IF also confirmed that CCL5 knockdown significantly decreased the intensity of Ki67, which indicated an impaired proliferation ability (Figure 2F). In vitro, these results suggest that CCL5 knockdown inhibits proliferation, migration, and invasion.
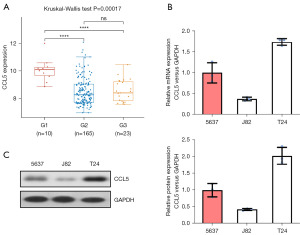
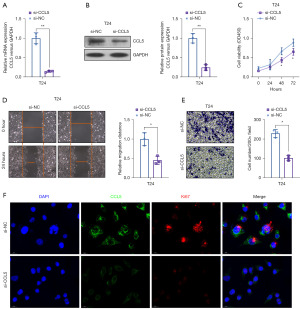
CCL5 over-expression improved the proliferation, migration, and invasion in vitro
To further evaluate the oncogenic role of CCL5 in BC, we over-expressed CCL5 in a low expressed BC cell line J82. In the overexpressed (OE)-CCL5 J82 cells, the level of CCL5 mRNA and protein had a 3.31±0.45- (Figure 3A, P<0.01) and 2.64±0.33-fold change (Figure 3B, P<0.01), respectively. In Figure 3C, we found that CCL5 over-expression improved the proliferation ability of BC cells, which was evaluated by CCK-8 assay (Figure 3C, P<0.05). Furthermore, CCL5 over-expression dramatically enhanced the migration and invasion abilities, as indicated by wound healing assay (Figure 3D, P<0.01) and transwell invasion assay (Figure 3E, P<0.01), respectively. Moreover, the results of IF confirmed that CCL5 over-expression significantly increased the intensity of Ki67, which suggested an improved proliferation ability (Figure 3F). These results suggested that CCL5 over-expression improves the proliferation, migration, and invasion of BC in vitro.
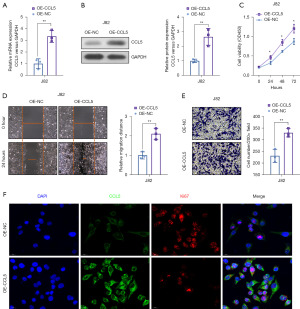
CCL5 significantly activated the JAK2-STAT3 signaling pathway
To explore whether CCL5 affects the activity of the JAK2-STAT3 signaling pathway, we analyzed the total and phosphorylated protein levels of JAK1, JAK2, and STAT3. In the CCL5 knockdown T24 BC cell line, total and phosphorylated protein levels for JAK1 remained unchanged, and although those for JAK2 and STAT3 remained unchanged, their phosphorylated levels were significantly lower (Figure 4A, P<0.05). Meanwhile, CCL5 over-expression significantly elevated the phosphorylated protein level of JAK2 and STAT3 (Figure 4B, P<0.05), which indicated an activation of the JAK2-STAT3 signaling pathway. These results demonstrated that CCL5 significantly activates the JAK2-STAT3 signaling pathway.
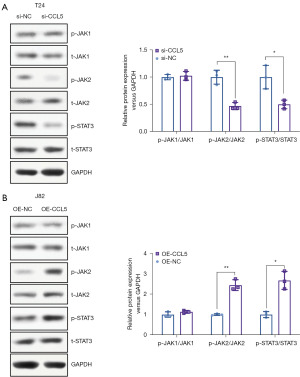
CCL5 over-expression improved the proliferation, migration, and invasion via activation of the JAK2-STAT3 signaling pathway
To further identify whether the JAK2-STAT3 signaling pathway is critical to the oncogenic role of CCL5 in BC, we used a specific JAK2 inhibitor, AG490, to block the activation of the JAK2-STAT3 signaling pathway. As shown in Figure 5A, AG490 treatment not only significantly impaired the cell proliferation ability in normal J82 BC cells, but also reversed the promotive effect of CCL5 over-expression on the cell proliferation ability (Figure 5A, P<0.05). Consistently, AG490 treatment significantly impaired the migration (Figure 5B, P<0.05) and invasion (Figure 5C, P<0.05) abilities of normal or CCL5 over-expressed J82 BC cells. These results demonstrated that over-expression of CCL5 enhanced proliferation, migration, and invasion through activation of the JAK2-STAT3 pathway.
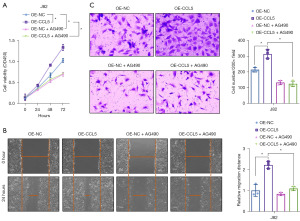
CCL5 over-expression improved the tumor growth and metastasis via activation of the JAK2-STAT3 signaling pathway
To verify whether the JAK2-STAT3 signaling pathway is critical to the oncogenic role of CCL5 in BC in vivo, we injected AG490 to block activation of the JAK2-STAT3 signaling pathway in the subcutaneous transplantation tumor model and tail vein injection pulmonary metastasis tumor model. As shown in Figure 6A,6B, CCL5 over-expression was found to significantly increase the J82 tumor growth (P<0.05), whereas AG490 treatment not only significantly impaired the tumor growth in normal J82 BC cells, but also reversed the promotive effect of CCL5 over-expression on the tumor growth. Similar changes were also shown in the tumor weight of generated tumors (Figure 6C, P<0.05). In the tail vein injection pulmonary metastasis tumor model, CCL5 over-expression significantly increased the lung metastasis number of J82 BC cells (Figure 6D,6E, P<0.05), which was obviously reversed by JAK2 inhibition. These results demonstrated that CCL5 over-expression improved the tumor growth and metastasis via activation of the JAK2-STAT3 signaling pathway.
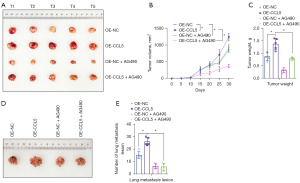
Discussion
NMIBC is a type of BC that affects the innermost lining of the bladder without invading deeper layers of the bladder wall. NMIBC accounts for the majority of all BC cases diagnosed annually. While NMIBC often responds well to treatment, recurrence is common and presents a major challenge in patient management. The main treatment for NMIBC is transurethral resection of the bladder tumor (TURBT), which involves removing the tumor through the urinary channel using a cystoscope. After TURBT, patients may undergo intravesical therapy, which involves placing medication directly into the bladder to prevent recurrence of the cancer. However, even with these interventions, NMIBC recurrence rates are high, with up to 50% of patients experiencing recurrence within 5 years of initial diagnosis (1,6). Given the high rates of recurrence, there is a pressing need to identify new therapeutic strategies for NMIBC. Recent studies have explored the use of immunotherapy and targeted therapies as potential options. Immunotherapy agents like Bacillus Calmette-Guerin (BCG) and checkpoint inhibitors have shown promise in treating NMIBC, but response rates vary widely among patients. Targeted therapies, which aim to block specific molecular pathways involved in tumor growth, have also emerged as a potential treatment option. For example, a study has shown that targeting the JAK/STAT pathway, which regulates cell proliferation and survival, may be an effective therapeutic approach for NMIBC. However, the underlying mechanism largely remains unclear. Emerging evidence indicates that CCL5/CCR5 signaling promotes cancer cell proliferation, survival, tumor initiation, and progression (16). One of the notable functions of CCL5/CCR5 signaling in BC is its involvement in tumor cell migration and invasion. CCL5, also known as RANTES (regulated on activation, normal T cell expressed and secreted), acts as a chemoattractant for immune cells expressing CCR5 (24). However, CCR5 is also expressed on BC cells, enabling autocrine signaling and promoting their motility and invasiveness. A study has shown that blocking CCR5 or silencing CCL5 expression can significantly reduce the migratory and invasive capabilities of BC cells, highlighting the importance of this signaling pathway in tumor progression (17). Furthermore, CCL5/CCR5 signaling has been implicated in angiogenesis, the process by which new blood vessels are formed to support tumor growth (25). CCL5 secreted by BC cells can recruit endothelial progenitor cells expressing CCR5, leading to increased angiogenesis within the tumor microenvironment. This enhanced blood vessel formation provides essential nutrients and oxygen to fuel tumor growth and facilitates the dissemination of cancer cells. Another function of CCL5/CCR5 signaling in BC is its involvement in immune evasion. CCL5 can attract immune cells, such as cytotoxic T cells and natural killer cells, to the tumor site (26). However, BC cells can hijack this signaling pathway to promote immune escape (27). By secreting high levels of CCL5, tumors create a chemotactic gradient that attracts immunosuppressive regulatory T cells expressing CCR5 (28). These regulatory T cells suppress the anti-tumor immune response, creating an immunosuppressive tumor microenvironment that facilitates tumor growth and progression. Additionally, CCL5/CCR5 signaling has been associated with drug resistance in BC. A study has shown that elevated CCR5 expression is correlated with resistance to chemotherapy drugs, such as cisplatin (18). The activation of CCR5 signaling pathways promotes cell survival and inhibits apoptosis, thus conferring resistance to cytotoxic agents. Targeting CCL5/CCR5 signaling may, therefore, hold promise as a strategy to overcome drug resistance and improve treatment outcomes in BC patients. According to our study, CCL5 promotes BC cell proliferation, migration, and invasive growth in vitro, as well as cancer cell growth and metastasis in vivo.
The chemokines are a large group of small cytokines whose molecular weights range from 7 to 15 kDa. About 50 chemokines have been identified so far (29). The chemokine CCL5, also classified as C-C-motility chemokine 5, is a potent chemokine that attracts white blood cells (30,31). It has been discovered that CCL5, along with CCL2, serves as a versatile inflammatory mediator in breast cancer cells through attraction of macrophages, T cells, granulocytes, mesenchymal stem cells, and promotion of angiogenesis (32). CCL5 is a protein that is mainly expressed on CD8+ T cells that has been associated with human immunodeficiency virus (HIV) (33). It most often binds to the homologous receptor CCR5, a receptor shared by HIV entry into CD4+ T cells (29), but also binds G-protein-coupled, such as CCR1 and CCR3 (34). Additionally, CD44 and GPR75 are non-traditional or co-receptors for CCL5. CCL5’s ability to bind multiple ligands highly efficiently allows for interaction with multiple signaling pathways resulting in a diverse range of physiological and pathological functions, including tumorigenesis (35).
A number of cancer subtypes, including colorectal, lung, prostate, breast, and cervical, have demonstrated high levels of CCL5 (24). Additionally, elevated tissue or plasma CCL5 has been associated with poor prognosis in cervical cancer, prostate cancer (18), stomach cancer (17), breast cancer (36), pancreatic cancer (37), and glioblastoma (22) where either cancer cells themselves or stromal cells express and secrete CCL5 (38). However, prior to the present study, the role of CCL5/CCR5 signaling in BC progression has been relatively unexplored. One possible explanation for CCL5’s ability to promote tumor growth and progression is its promotion of JAK2, an important intracellular mediator of hormone signal transduction and cytokines (39). JAK2 activates a series of signaling pathways is activated, including the STAT pathway (40). The JAK2/STAT3 pathway plays an important role in lung cancer cell growth, migration, and metastasis (41). When JAK2/STAT3 phosphorylation is inhibited in cancer cells, it may reduce their proliferation and metastasis both in vitro (42) and in vivo (43).
In this study, we generated a CCL5 knocked-down T24 BC cell line and CCL5 over-expressed J82 BC cell line, demonstrating that CCL5 promotes BC cell proliferation and migration in vitro, as well as tumor growth and metastasis in vivo. Moreover, we found CCL5 activation of tumor progression may be related to the JAK2/STAT3 pathways. This association between CCL5 and the JAK2/STAT3 pathway with malignancies, including BC as indicated by our results, offers intriguing options for diagnostic indicators and prognostic biomarkers, as well as potential therapeutic targets for BC and other diseases (44,45). For example, an entry inhibitor based on CCR5 approved by the United States Food and Drug Administration in 2007 known as maraviroc (MRV) appears to promote the internalization of CCR5, does not rely on cellular signaling, and is clinically beneficial for HIV patients with mild side effects. Furthermore, in our study, AG490 (a JAK2 inhibitor) inhibited tumor proliferation, migration, invasion, and metastasis both in vitro and in vivo, even when CCL5 was over-expressed.
Though these results are encouraging and provide evidence of the role of CCL5 in BC, this study has some limitations that need to be acknowledged. One limitation is that the study was conducted using only two BC cell lines, which may not fully represent the complexity and heterogeneity of human BC. Additionally, the study did not investigate the effect of inhibiting other components of the JAK/STAT pathway, which may play a role in BC progression.
Conclusions
CCL5 contributes to the development of BC, likely related to activation of the JAK2/STAT3 pathway. CCL5 may be used as a marker for BC diagnosis or prognosis and inhibition of its interactions and associated pathways offer potential therapeutic targets.
Acknowledgments
We would like to thank Professor Takuya Koie (Department of Urology, Gifu University Graduate School of Medicine, Japan), for his valuable revisions to the article.
Funding: This work was supported by grants from
Footnote
Reporting Checklist: The authors have completed the ARRIVE reporting checklist. Available at https://tau.amegroups.com/article/view/10.21037/tau-23-540/rc
Data Sharing Statement: Available at https://tau.amegroups.com/article/view/10.21037/tau-23-540/dss
Peer Review File: Available at https://tau.amegroups.com/article/view/10.21037/tau-23-540/prf
Conflicts of Interest: All authors have completed the ICMJE uniform disclosure form (available at https://tau.amegroups.com/article/view/10.21037/tau-23-540/coif). L.S.L. receives honorarium from Bayer, Bristol Meyer Squibb, AstraZeneca for educational events, payment for expert testimony for medicolegal advise. The other authors have no conflicts of interest to declare.
Ethical Statement: The authors are accountable for all aspects of the work in ensuring that questions related to the accuracy or integrity of any part of the work are appropriately investigated and resolved. Animal experiments were performed under a project license granted by ethics committee of the Third Affiliated Hospital of Soochow University, in compliance with the institutional guidelines for the care and use of animals.
Open Access Statement: This is an Open Access article distributed in accordance with the Creative Commons Attribution-NonCommercial-NoDerivs 4.0 International License (CC BY-NC-ND 4.0), which permits the non-commercial replication and distribution of the article with the strict proviso that no changes or edits are made and the original work is properly cited (including links to both the formal publication through the relevant DOI and the license). See: https://creativecommons.org/licenses/by-nc-nd/4.0/.
References
- Tran L, Xiao JF, Agarwal N, et al. Advances in bladder cancer biology and therapy. Nat Rev Cancer 2021;21:104-21. [Crossref] [PubMed]
- Bundell S, Petrić Howe N. Why bladder cancer cells that shed their Y chromosome become more aggressive. Nature 2023; Epub ahead of print. [Crossref] [PubMed]
- Feng M, Matoso A, Epstein G, et al. Identification of Lineage-specific Transcriptional Factor-defined Molecular Subtypes in Small Cell Bladder Cancer. Eur Urol 2023; Epub ahead of print. [Crossref]
- Chen W, Zheng R, Baade PD, et al. Cancer statistics in China, 2015. CA Cancer J Clin 2016;66:115-32. [Crossref] [PubMed]
- Siegel RL, Miller KD, Jemal A. Cancer statistics, 2020. CA Cancer J Clin 2020;70:7-30. [Crossref] [PubMed]
- Siegel RL, Miller KD, Fuchs HE, et al. Cancer Statistics, 2021. CA Cancer J Clin 2021;71:7-33. [Crossref] [PubMed]
- Allahyari M, Motavalizadeh-Kakhky AR, Mehrzad J, et al. Cellulose nanocrystals derived from chicory plant: an un-competitive inhibitor of aromatase in breast cancer cells via PI3K/AKT/mTOP signalling pathway. J Biomol Struct Dyn 2023; Epub ahead of print. [Crossref] [PubMed]
- Malek-Esfandiari Z, Rezvani-Noghani A, Sohrabi T, et al. Molecular Dynamics and Multi-Spectroscopic of the Interaction Behavior between Bladder Cancer Cells and Calf Thymus DNA with Rebeccamycin: Apoptosis through the Down Regulation of PI3K/AKT Signaling Pathway. J Fluoresc 2023;33:1537-57. [Crossref] [PubMed]
- Wu Q, Chen P, Li J, et al. Inhibition of bladder cancer growth with homoharringtonine by inactivating integrin α5/β1-FAK/Src axis: A novel strategy for drug application. Pharmacol Res 2023;188:106654. [Crossref] [PubMed]
- Festa BP, Siddiqi FH, Jimenez-Sanchez M, et al. Microglial cytokines poison neuronal autophagy via CCR5, a druggable target. Autophagy 2023; Epub ahead of print. [Crossref] [PubMed]
- Festa BP, Siddiqi FH, Jimenez-Sanchez M, et al. Microglial-to-neuronal CCR5 signaling regulates autophagy in neurodegeneration. Neuron 2023;111:2021-2037.e12. [Crossref] [PubMed]
- Gauthier M, Kale SL, Oriss TB, et al. CCL5 is a potential bridge between type 1 and type 2 inflammation in asthma. J Allergy Clin Immunol 2023;152:94-106.e12. [Crossref] [PubMed]
- Labaki C, Saad E, Choueiri TK, et al. Oligometastatic Bladder Cancer: Defining a Novel Entity. Eur Urol 2023;84:390-2. [Crossref] [PubMed]
- Rawat K, Tewari A, Li X, et al. CCL5-producing migratory dendritic cells guide CCR5+ monocytes into the draining lymph nodes. J Exp Med 2023;220:e20222129. [Crossref] [PubMed]
- Wu S, Yang S, Li R, et al. HSV-1 infection-induced herpetic neuralgia involves a CCL5/CCR5-mediated inflammation mechanism. J Med Virol 2023;95:e28718. [Crossref] [PubMed]
- Aldinucci D, Borghese C, Casagrande N. The CCL5/CCR5 Axis in Cancer Progression. Cancers (Basel) 2020;12:1765. [Crossref] [PubMed]
- Aldinucci D, Casagrande N. Inhibition of the CCL5/CCR5 Axis against the Progression of Gastric Cancer. Int J Mol Sci 2018;19:1477. [Crossref] [PubMed]
- Huang R, Guo L, Gao M, et al. Research Trends and Regulation of CCL5 in Prostate Cancer. Onco Targets Ther 2021;14:1417-27. [Crossref] [PubMed]
- Huang R, Wang S, Wang N, et al. CCL5 derived from tumor-associated macrophages promotes prostate cancer stem cells and metastasis via activating beta-catenin/STAT3 signaling. Cell Death Dis 2020;11:234. [Crossref] [PubMed]
- Parry H, Martin K. Single-dose i.v. dexamethasone--an effective anti-emetic in cancer chemotherapy. Cancer Chemother Pharmacol 1991;28:231-2. [Crossref] [PubMed]
- Korbecki J, Grochans S, Gutowska I, et al. CC Chemokines in a Tumor: A Review of Pro-Cancer and Anti-Cancer Properties of Receptors CCR5, CCR6, CCR7, CCR8, CCR9, and CCR10 Ligands. Int J Mol Sci 2020;21:7619. [Crossref] [PubMed]
- Kranjc MK, Novak M, Pestell RG, et al. Cytokine CCL5 and receptor CCR5 axis in glioblastoma multiforme. Radiol Oncol 2019;53:397-406. [Crossref] [PubMed]
- Marques RE, Guabiraba R, Russo RC, et al. Targeting CCL5 in inflammation. Expert Opin Ther Targets 2013;17:1439-60. [Crossref] [PubMed]
- Zeng Z, Lan T, Wei Y, et al. CCL5/CCR5 axis in human diseases and related treatments. Genes Dis 2022;9:12-27. [Crossref] [PubMed]
- Wang SW, Liu SC, Sun HL, et al. CCL5/CCR5 axis induces vascular endothelial growth factor-mediated tumor angiogenesis in human osteosarcoma microenvironment. Carcinogenesis 2015;36:104-14. [Crossref] [PubMed]
- Raghu H, Lepus CM, Wang Q, et al. CCL2/CCR2, but not CCL5/CCR5, mediates monocyte recruitment, inflammation and cartilage destruction in osteoarthritis. Ann Rheum Dis 2017;76:914-22. [Crossref] [PubMed]
- Wang Z, Wu Z, Wang H, et al. An immune cell atlas reveals the dynamics of human macrophage specification during prenatal development. Cell 2023;186:4454-4471.e19. [Crossref] [PubMed]
- Lin J, Xu Y, Guo P, et al. CCL5/CCR5-mediated peripheral inflammation exacerbates blood-brain barrier disruption after intracerebral hemorrhage in mice. J Transl Med 2023;21:196. [Crossref] [PubMed]
- Harlin H, Meng Y, Peterson AC, et al. Chemokine expression in melanoma metastases associated with CD8+ T-cell recruitment. Cancer Res 2009;69:3077-85. [Crossref] [PubMed]
- Borsig L, Wolf MJ, Roblek M, et al. Inflammatory chemokines and metastasis--tracing the accessory. Oncogene 2014;33:3217-24. [Crossref] [PubMed]
- de Oliveira CE, Oda JM, Losi Guembarovski R, et al. CC chemokine receptor 5: the interface of host immunity and cancer. Dis Markers 2014;2014:126954. [Crossref] [PubMed]
- Fang H, Jin J, Huang D, et al. PAI-1 induces Src inhibitor resistance via CCL5 in HER2-positive breast cancer cells. Cancer Sci 2018;109:1949-57. [Crossref] [PubMed]
- Halama N, Zoernig I, Berthel A, et al. Tumoral Immune Cell Exploitation in Colorectal Cancer Metastases Can Be Targeted Effectively by Anti-CCR5 Therapy in Cancer Patients. Cancer Cell 2016;29:587-601. [Crossref] [PubMed]
- Pervaiz A, Zepp M, Georges R, et al. Antineoplastic effects of targeting CCR5 and its therapeutic potential for colorectal cancer liver metastasis. J Cancer Res Clin Oncol 2021;147:73-91. [Crossref] [PubMed]
- Song S, He X, Wang J, et al. A novel long noncoding RNA, TMEM92-AS1, promotes gastric cancer progression by binding to YBX1 to mediate CCL5. Mol Oncol 2021;15:1256-73. [Crossref] [PubMed]
- Derossi DR, Amarante MK, Guembarovski RL, et al. CCL5 protein level: influence on breast cancer staging and lymph nodes commitment. Mol Biol Rep 2019;46:6165-70. [Crossref] [PubMed]
- Wang X, Li X, Wei X, et al. PD-L1 is a direct target of cancer-FOXP3 in pancreatic ductal adenocarcinoma (PDAC), and combined immunotherapy with antibodies against PD-L1 and CCL5 is effective in the treatment of PDAC. Signal Transduct Target Ther 2020;5:38. [Crossref] [PubMed]
- Zhang S, Zhong M, Wang C, et al. CCL5-deficiency enhances intratumoral infiltration of CD8(+) T cells in colorectal cancer. Cell Death Dis 2018;9:766. [Crossref] [PubMed]
- Qian CJ, Yao J, Si JM. Nuclear JAK2: form and function in cancer. Anat Rec (Hoboken) 2011;294:1446-59. [Crossref] [PubMed]
- Lu R, Zhang YG, Sun J. STAT3 activation in infection and infection-associated cancer. Mol Cell Endocrinol 2017;451:80-7. [Crossref] [PubMed]
- Gao Y, Yang J, Cai Y, et al. IFN-γ-mediated inhibition of lung cancer correlates with PD-L1 expression and is regulated by PI3K-AKT signaling. Int J Cancer 2018;143:931-43. [Crossref] [PubMed]
- Park SY, Lee CJ, Choi JH, et al. The JAK2/STAT3/CCND2 Axis promotes colorectal Cancer stem cell persistence and radioresistance. J Exp Clin Cancer Res 2019;38:399. [Crossref] [PubMed]
- Wang S, Liang K, Hu Q, et al. JAK2-binding long noncoding RNA promotes breast cancer brain metastasis. J Clin Invest 2017;127:4498-515. [Crossref] [PubMed]
- Xu J, Shi Q, Lou J, et al. Chordoma recruits and polarizes tumor-associated macrophages via secreting CCL5 to promote malignant progression. J Immunother Cancer 2023;11:e006808. [Crossref] [PubMed]
- Zhang XN, Yang KD, Chen C, et al. Pericytes augment glioblastoma cell resistance to temozolomide through CCL5-CCR5 paracrine signaling. Cell Res 2021;31:1072-87. [Crossref] [PubMed]