Ramelteon attenuates renal ischemia and reperfusion injury through reducing mitochondrial fission and fusion and inflammation
Highlight box
Key findings
• Ramelteon improved renal ischemia-reperfusion injury (IRI).
• Ramelteon reduced IRI-induced renal apoptosis and oxidative stress.
• Ramelteon abated the IRI-induced renal inflammatory response.
• Ramelteon inhibited cell apoptosis and mitochondrial damage in the hypoxia/reoxygenation (H/R) model.
• Ramelteon inhibits H/R-induced inflammatory response.
What is known and what is new?
• Renal IRI induced renal dysfunction and pro-inflammatory cytokine production.
• Ramelteon improved renal IRI and abated the IRI-induced renal inflammatory response.
What is the implication, and what should change now?
• These data elucidated that ramelteon may be a candidate for prevention of acute kidney injury.
Introduction
Acute kidney injury (AKI) is associated with noticeable morbidity and mortality in the clinic and limited effective treatment options are available (1). Ischemia-reperfusion injury (IRI) occurs frequently during renal transplantation procedures and is a leading cause of AKI (2,3). Tubular atrophy and interstitial fibrosis occur during renal IRI, resulting in various clinical manifestations (4). The molecular mechanisms of renal IRI include ion accumulation, oxidative stress, mitochondrial dysfunction, and inflammatory reaction (5,6). When the internal environment becomes hypoxic, the electron transport chain in mitochondria is imbalanced, leading to lower levels of adenosine triphosphate (ATP) and elevated levels of reactive oxygen species (ROS) (7). The triggered apoptotic pathways result in cellular dysfunction, and the mitochondria become fragmented (8). Although the pathogenesis of renal IRI has been extensively studied, there are no efficient therapeutic measures for renal IRI.
Ramelteon is a highly selective melatonin (MT) subtypes 1 and 2 (MT1 and MT2) receptor agonist and the first non-addictive agent for insomnia (9). Previous studies have shown that ramelteon attenuates injury induced by oxidative stress and lipopolysaccharide (LPS). For example, ramelteon protects the brain via relieving inflammation and oxidative stress though the Nrf2 signaling pathway (10). Ramelteon attenuates LPS-induced experimental acute ocular inflammation though hypoxia-inducible factor-1α/vascular endothelial growth factor/endothelial nitric oxide synthase signaling (11). Imai et al. elucidated that ramelteon may alleviate the risk of vancomycin-induced nephrotoxicity (12). However, whether ramelteon exerts useful roles in renal IRI is still unknown.
In this study, we used IRI to treat mice and hypoxia/reoxygenation (H/R) to treat HK-2 cells to establish in vivo and in vitro AKI models, respectively. We aimed to elaborate the potentially therapeutic benefits of ramelteon against AKI. We present this article in accordance with the ARRIVE reporting checklist (available at https://tau.amegroups.com/article/view/10.21037/tau-23-543/rc).
Methods
Animal model of IRI
Twenty-five Male C57BL/6 mice, aged 10 weeks and weighing 21–25 g, were purchased from Jinan Pengyue Experimental Animal Breeding Co., Ltd. (Jinan, China). Mice were placed in a constant climate with abundant food and water. All mice were randomly divided into five groups (n=5): (I) Sham; (II) IRI; (III) IRI + ramelteon (0.3 mg/kg); (IV) IRI + ramelteon (1 mg/kg); and (V) IRI + ramelteon (3 mg/kg). The concentrations of ramelteon were chosen as described in a previous study (13). Ramelteon (HY-A0014; MCE) was dissolved in saline containing 0.5% carboxymethylcellulose. Each mouse in group (III), (IV), and (V) was intraperitoneally (i.p.) treated with ramelteon (0.3, 1, and 3 mg/kg/day) for 7 days. Each mouse in group (I) and (II) was treated with an equal volume of saline. The IRI mice model was established as described in a previous study (14). Mice were anesthetized by sodium pentobarbital (50 mg/kg, i.p.), placed on a heat surgical pad (37 ℃), followed by midline laparotomy. Both sides of the renal artery were occluded with clamps (RS-5459; Roboz Surgical Instrument Company, Gaithersburg, MD, USA) for 30 minutes. Then, the clamp was removed for blood reperfusion. When the color of the kidney surface changes from dark purple to blood, it indicates that the blood flow is restored (15). At 24 hours after reperfusion had been initiated, the mice were euthanized, and kidney samples were collected. Animal experiments were performed under a project license (No. 2022S1066) granted by ethics committee of The First Affiliated Hospital of Shandong First Medical University & Shandong Provincial Qianfoshan Hospital, in compliance with national guidelines for the care and use of animals. A protocol was prepared before the study without registration.
Cell culture and treatment
HK-2 cells (Procell, Wuhan, China) were cultured in minimal essential medium non-essential amino acids (MEM NEAA) (PM150410; Procell) supplemented with 10% fetal bovine serum (FBS) (12483020; Gibco, Waltham, MA, USA) and 1% penicillin-streptomycin (P1400; Solarbio, Beijing, China). HK-2 cells were randomly divided into five groups: (I) control; (II) H/R; (III) H/R + ramelteon (10 nM); (IV) H/R + ramelteon (30 nM); and (V) H/R + ramelteon (60 nM). Ramelteon concentrations were determined based on previous studies (16,17). HK-2 cells were prophylactically treated with ramelteon and then exposed to H/R. For hypoxia, HK-2 cells were cultured in a 3-gas incubator (5% CO2, 94% N2, and 1% O2) for 24 hours. For reoxygenation, cells were cultured in a regular incubator for 12 hours. CRX-527 (TLR4 agonist; TLRL-CRX527, InvivoGen, Toulouse, France) were added to cells for 24 hours.
Assessment of kidney injury
Concentrations of serum creatinine (Cr) (C011-2-1; Nanjing Jiancheng Bioengineering Institute, Nanjing, China), blood urea nitrogen (BUN) (C013-2-1, Nanjing Jiancheng Bioengineering Institute), and kidney injury molecule-1 (KIM-1) (ab213477, Abcam, Cambridge, UK) were evaluated according to manufacturer’s protocols. Tissues were fixed in 4% paraformaldehyde, embedded in paraffin, and cut into 5-µm slices. Hematoxylin and eosin (H&E)- and periodic acid-Schiff (PAS)-stained renal slices were photographed under a microscope (Olympus, Tokyo, Japan). Two blinded pathologists assessed the sections. The scoring was performed by grading the tubular injury, epithelial cell apoptosis, intraluminal cast, and brush border loss. Renal tubular injury was scored as follows: normal [0]; damage <10% [1]; 10–25% [2]; >25–75% [3]; and >75% [4].
Enzyme-linked immunosorbent assay (ELISA)
The levels of interleukin 6 (IL-6) (PI326; Beyotime, Beijing, China), tumor necrosis factor-α (TNF-α) (SEKM-0034, Solarbio), interleukin 1β (IL-1β) (SEKM-0002, Solarbio) in serum were detected using ELISA kits.
Superoxide dismutase (SOD), catalase (CAT), and glutathione (GSH) analysis
Mice renal samples were homogenized and centrifuged. The supernatant was then collected for SOD, CAT, and GSH analysis using kits (A001-1-2, A007-2-1, and A006-1-1, Nanjing Jiancheng Bioengineering Institute) according to the manufacturer’s protocols.
ATP detection
ATP content was assessed using ATP assay kits (BC0305; Solarbio) according to the manufacturer’s protocols.
Apoptosis
The apoptosis of cells was assessed using an apoptosis detection kit (C1062S; Beyotime). Cells were incubated with 5 µL Annexin V-fluorescein isothiocyanate (FITC) and 10 µL propidium iodide (PI) staining solutions for 15 minutes protected from light at 25 ℃. Samples were determined using flow cytometry [Becton, Dickinson, and Co. (BD) Biosciences, Franklin Lakes, USA].
Mitochondrial membrane potential (MMP)
According to the operation instructions of the MMP assay kit (C2006; Beyotime). The cells incubated with JC-1 solution for 30 minutes, washed with JC-1 staining buffer, and applied to flow cytometer (BD Biosciences) for analysis.
Intracellular ROS
Intracellular ROS level was determined using ROS Assay Kit (S0033S; Beyotime). After 10 µM dichloro-dihydro-fluorescein diacetate (DCFH-DA) was added at 37 ℃ for 20 minutes, the cells were washed, and detected within 30 minutes using a flow cytometer (BD Biosciences).
Immunofluorescence staining
Sections were added with anti-cytochrome C (cyt-c) (1:200; ab133504, Abcam) at 4 ℃ overnight. After washing using phosphate-buffered saline (PBS) three times, sections were incubated with a secondary antibody (1:200, SA00013-2; Proteintech, Wuhan, China) for 50 minutes. 4',6-diamidino-2-phenylindole (DAPI) was added to the sections for 10 minutes. Then, sections were visualized under a microscope (BX51, Olympus).
Western blotting
Tissues and cells were lysed in radioimmunoprecipitation assay (RIPA) buffer (Solarbio). Proteins were subjected to 12% sodium dodecyl sulfate polyacrylamide gel electrophoresis (SDS-PAGE) and transferred to polyvinylidene fluoride (PVDF) membranes. Membranes were incubated with Bax (1:2,000; 50599-2-Ig; Proteintech), Bcl-2 (1:2,000; 26593-1-AP; Proteintech), TLR4 (1:2,000; 66350-1-Ig; Proteintech), MyD88 [1:1,000; 4283; Cell Signaling Technology (CST), Danvers, MA, USA], p-IκBα (1:1,000; ab133462; Abcam), IκBα (1:1,000; ab32518; Abcam), p-p65 NF-κB (1:1,000; 3033; CST), p65 NF-κB (1:1,000; 8242; CST), Drp1 (1:1,000; 8570; CST), Fis1 (1:1,000; 10956-1-AP; Proteintech), Mff (1:5,000; 17090-1-AP; Proteintech), Mfn1 (1:1,000; 13798-1-AP; Proteintech), Mfn2 (1:2,000; 12186-1-AP; Proteintech), and β-actin (1:2,000; 20536-1-AP; Proteintech) at 4 ℃. The membranes were then incubated with a secondary antibody (1:5,000; SA00001-2; Proteintech) for 1 hour. The relative quantity of proteins was determined by enhanced chemiluminescence (ECL) reagents.
Real-time quantitative polymerase chain reaction (RT-qPCR)
The RNA isolater Total RNA Extraction Reagent (R401-01; Vazyme, Nanjing, China) was used to extract total RNA from cells according to the manufacturer’s instructions. HiScript II Q Select RT SuperMix (R223-01; Vazyme) was used to reverse complementary DNA (cDNA). ChamQ SYBR qPCR Master Mix (Q311-02; Vazyme) was used to measure the relative messenger RNA (mRNA) levels. The primer sequences used for PCR were as follows: IL-6, forward: 5'-TCCTTCTCCACAAACATGTAACAA-3' and reverse: 5'-TCACCAGGCAAGTCTCCTCA-3'; TNF-α forward: 5'-TCTCCTTCCTGATCGTGGCA-3' and reverse: 5'-CAGCTTGAGGGTTTGCTACAAC-3'; IL-1β forward: 5'-ATGATGGCTTATTACAGTGGCAA-3' and reverse: 5'-CCTTGCTGTAGTGGTGGTCG-3'; β-actin forward: 5'-CCGCCGCCAGCTCAC-3' and reverse: 5'-TCGTCGCCCACATAGGAATC-3'.
Statistical analysis
All data were expressed as mean ± standard deviation (SD). The software GraphPad Prism 8 (GraphPad Software, San Diego, CA, USA) was used for data analysis. One way analysis of variance (ANOVA) with Tukey’s post-hoc was used to compare multiple groups. Statistical significance was considered at P<0.05.
Results
Ramelteon improved renal IRI
H&E- and PAS-stained renal slices were used to detect kidney injury. Treatment of ramelteon prior to IRI improved renal morphology, and showed less necrosis and swelling of the tubular cells (Figure 1A,1B). When the kidneys endure IRI, renal excretion of Cr and BUN are lowered; thus, they are enhanced in the blood stream (18). Furthermore, KIM-1 is a renal tubular damage biomarker (19). As revealed in Figure 1C-1E, serum Cr, BUN, and KIM-1 levels were all noticeably higher in the IRI group in relation to the Sham group; ramelteon administration significantly weakened the increased levels of Cr, BUN, and KIM-1. These results indicate that ramelteon treatment prior to IRI may effectively alleviate renal IRI.
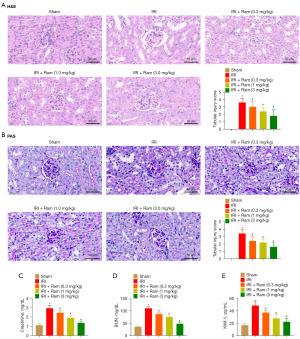
Ramelteon reduced IRI-induced renal apoptosis and oxidative stress
Subsequently, we tested the expression of Bax and Bcl-2, which are apoptotic markers. Bax expression was increased and Bcl-2 expression was decreased in the IRI group, whereas ramelteon administration reversed these changes (Figure 2A). To detect the oxidative stress during IRI, many antioxidant factors were measured. The current study indicated that in IRI mice, the content of SOD, CAT, and GSH were dramatically reduced, whereas ramelteon treatment elevated SOD, CAT, and GSH levels in IRI-induced mice (Figure 2B-2D).
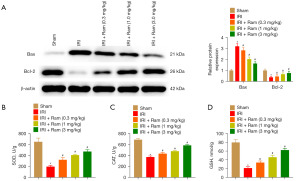
Ramelteon abated the IRI-induced renal inflammatory response
To estimate the anti-inflammatory effect of ramelteon, the levels of cytokine and TLR4/MyD88/NF-κB pathway-related proteins were detected. IRI led to increased levels of IL-6, TNF-α, and IL-1β. These alterations were considerably blunted by ramelteon treatment in IRI mice (Figure 3A-3C). The expression of TLR4, MyD88, p-IκBα, and p-p65 NF-κB was increased in the IRI group, and ramelteon treatment observably decreased the expression of these proteins in the IRI model (Figure 3D). These results indicated that ramelteon alleviates renal IRI by inhibiting the TLR4/MyD88/NF-κB pathway.
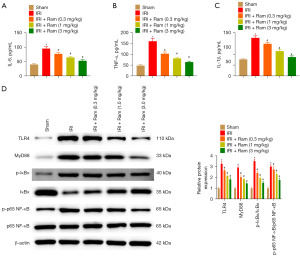
Ramelteon inhibited cell apoptosis and mitochondrial damage in the H/R model
The results of flow cytometry revealed that ramelteon treatment reduced H/R-induced apoptosis (Figure 4A). In H/R mediated cells, ramelteon treatment declined Bax and enhanced Bcl-2 (Figure 4B). Next, we evaluated the effect of ramelteon on mitochondrial damage, which can regulate ROS level and energy metabolism. Ramelteon administration decreased ROS production (Figure 4C). MMP collapse plays a vital role in mitochondrial dysfunction. Ramelteon administration was shown to prevent H/R-induced decline in MMP (Figure 4D). In the H/R model, excessive ROS was accumulated because of ATP depletion. Ramelteon administration markedly elevated the ATP levels (Figure 4E). Cyt-c is a key marker of mitochondrial electron transport chain (20). H/R elevated the translocation of cyt-c to cytoplasm, whereas, ramelteon administration blunted H/R-induced cyt-c release (Figure 4F). Then, Drp1, Fis1, Mff, Mfn1, and Mfn2 expression were detected to evaluate mitochondrial dynamics. We found that Drp1, Fis1, and Mff expression was increased whereas Mfn1 and Mfn2 expression was inhibited by H/R. Ramelteon administration partly abated these changes (Figure 4G).
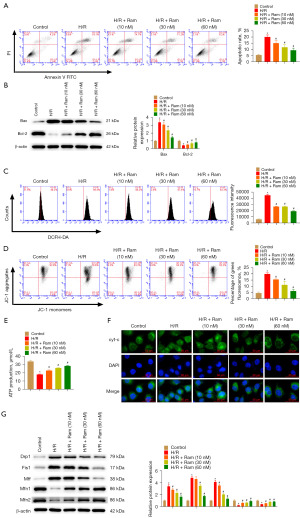
Ramelteon inhibits H/R-induced inflammatory response
RT-qPCR revealed that ramelteon down-regulated the mRNA expression of IL-6, TNF-α, and IL-1β after H/R treatment (Figure 5A-5C). Moreover, TLR4, MyD88, p-IκBα, and p-p65 NF-κB protein levels evidently decreased in response to ramelteon (Figure 5D). We detected whether ramelteon ameliorated the inflammatory response by inhibiting TLR4/MyD88/NF-κB. Therefore, we used TLR4 agonist CRX-527 to promote TLR4 expression. H/R-induced IL-6, TNF-α, and IL-1β mRNA expression were abrogated by ramelteon administration. However, CRX-527 treatment suppressed the effects of ramelteon on these changes (Figure 5E-5G).
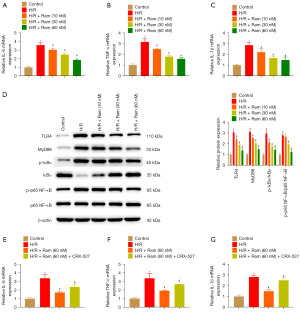
Discussion
IRI has become recognized as a common factor that affects renal function. Since it usually occurs during clinical renal transplant surgery, there are no measures to prevent it from happening (21). It is vitally important to develop drugs for alleviation of this kind of injury. Increasingly, research has reported that ROS, mitochondrial injury, and the inflammatory response play essential roles in renal IRI (22,23). Ramelteon is a selective MT1/2 receptor agonist and is predominantly used to treat difficulty falling asleep (24). In recent years, numerous studies have suggested that ramelteon protects against injury on human tissues induced by oxidative stress and LPS (17,25,26). The current study specified that ramelteon attenuates renal IRI and H/R-induced apoptosis by mitigating ROS generation, mitochondrial injury, and inflammatory response. We found that 3 mg/kg and 60 nM ramelteon showed the maximal efficacy in renal IRI and H/R-induced HK-2 cells. Thus, ramelteon may be a novel agent for alleviating AKI.
Numerous studies have shown a relationship between ramelteon and apoptosis. Kandezi et al. showed that ramelteon effectively counteracted apoptosis induced by cocaine (27). Wu et al. found that ramelteon has a protective effect against apoptosis induced by ventilator use (28). Similarly, we found that ramelteon decreased cell apoptosis. Furthermore, ramelteon administration increased Bcl-2 expression but decreased Bax expression in mice and cells models. These data suggest that ramelteon protects tubular epithelial cells against IRI-induced apoptosis.
Mitochondria are the site of ROS production (29). It is widely accepted that excessive ROS production aggravates renal IRI and decreases the level of MMP (30,31). Iwata et al. indicated that d-alanine inhibits ROS production and enhances MMP via N-methyl-d-aspartate receptor in AKI (32). Song et al. highlighted that dexmedetomidine attenuates changes in MMP of H/R-induced cells (33). Our research demonstrated that ramelteon increased MMP of HK-2 cells treated with H/R. As critical components of mitochondrial fission and fusion, Drp1, Fis1, Mff, Mfn1, and Mfn2 are involved in the modulation of renal function during H/R (15). We found that Drp1, Fis1, and Mff expression was increased whereas Mfn1 and Mfn2 expression was inhibited by H/R. Ramelteon administration partly abated these changes. These data suggest that ramelteon exerted its kidney protective effect by modulating mitochondrial fission and fusion.
The inflammatory response has a major role in the pathological progression of renal IRI (34). In the progression of renal IRI, HK-2 cells produce inflammatory factors that promote the inflammatory response (35). In our study, pro-inflammatory cytokines were declined in mice and cells following ramelteon administration. This result elucidates that ramelteon alleviates IRI by mitigating the inflammatory response. TLR4 is involved in the pathophysiology of inflammation and AKI induced by renal IRI (36). Yang et al. showed that MT promotes autophagy and ameliorates renal IRI though TLR4/MyD88/MEK/ERK/mTORC1 (37). Another study verified that activation of NF-κB exacerbates tubular injury and the inflammatory response in renal IRI (38). Ramelteon inhibits the NF-κB pathway by suppressing the phosphorylation of IκBα in LPS-induced astrocytes (39). We found that TLR4, MyD88, p-IκBα, and p-p65 NF-κB protein levels evidently decreased in response to ramelteon. Ramelteon administration decreased H/R-induced IL-6, TNF-α, and IL-1β mRNA expression; however, CRX-527 (TLR4 agonist) treatment suppressed the effects of ramelteon on these changes. It is reasonable to suggest that the ramelteon-induced reduction of the inflammatory response in renal IRI is partially mediated by the TLR4/MyD88/NF-κB pathway.
Conclusions
In conclusion, ramelteon treatment reduced apoptotic processes and inflammatory responses by regulating the TLR4/MyD88/NF-κB pathways following renal IRI. These data elucidated that ramelteon may be a candidate for prevention of AKI.
Acknowledgments
Funding: None.
Footnote
Reporting Checklist: The authors have completed the ARRIVE reporting checklist. Available at https://tau.amegroups.com/article/view/10.21037/tau-23-543/rc
Data Sharing Statement: Available at https://tau.amegroups.com/article/view/10.21037/tau-23-543/dss
Peer Review File: Available at https://tau.amegroups.com/article/view/10.21037/tau-23-543/prf
Conflicts of Interest: All authors have completed the ICMJE uniform disclosure form (available at https://tau.amegroups.com/article/view/10.21037/tau-23-543/coif). The authors have no conflicts of interest to declare.
Ethical Statement: The authors are accountable for all aspects of the work in ensuring that questions related to the accuracy or integrity of any part of the work are appropriately investigated and resolved. Animal experiments were performed under a project license (No. 2022S1066) granted by Ethics Committee of The First Affiliated Hospital of Shandong First Medical University & Shandong Provincial Qianfoshan Hospital, in compliance with national guidelines for the care and use of animals.
Open Access Statement: This is an Open Access article distributed in accordance with the Creative Commons Attribution-NonCommercial-NoDerivs 4.0 International License (CC BY-NC-ND 4.0), which permits the non-commercial replication and distribution of the article with the strict proviso that no changes or edits are made and the original work is properly cited (including links to both the formal publication through the relevant DOI and the license). See: https://creativecommons.org/licenses/by-nc-nd/4.0/.
References
- Gumbert SD, Kork F, Jackson ML, et al. Perioperative Acute Kidney Injury. Anesthesiology 2020;132:180-204. [Crossref] [PubMed]
- Saat TC, van den Akker EK, IJzermans JN, et al. Improving the outcome of kidney transplantation by ameliorating renal ischemia reperfusion injury: lost in translation? J Transl Med 2016;14:20. [Crossref] [PubMed]
- Topdağı Ö, Tanyeli A, Akdemir FNE, et al. Preventive effects of fraxin on ischemia/reperfusion-induced acute kidney injury in rats. Life Sci 2020;242:117217. [Crossref] [PubMed]
- Wang F, Otsuka T, Adelnia F, et al. Multiparametric magnetic resonance imaging in diagnosis of long-term renal atrophy and fibrosis after ischemia reperfusion induced acute kidney injury in mice. NMR Biomed 2022;35:e4786. [Crossref] [PubMed]
- Nieuwenhuijs-Moeke GJ, Pischke SE, Berger SP, et al. Ischemia and Reperfusion Injury in Kidney Transplantation: Relevant Mechanisms in Injury and Repair. J Clin Med 2020;9:253. [Crossref] [PubMed]
- Granata S, Votrico V, Spadaccino F, et al. Oxidative Stress and Ischemia/Reperfusion Injury in Kidney Transplantation: Focus on Ferroptosis, Mitophagy and New Antioxidants. Antioxidants (Basel) 2022;11:769. [Crossref] [PubMed]
- Su L, Zhang J, Gomez H, et al. Mitochondria ROS and mitophagy in acute kidney injury. Autophagy 2023;19:401-14. [Crossref] [PubMed]
- Wang S, Zhu H, Li R, et al. DNA-PKcs interacts with and phosphorylates Fis1 to induce mitochondrial fragmentation in tubular cells during acute kidney injury. Sci Signal 2022;15:eabh1121. [Crossref] [PubMed]
- Borja NL, Daniel KL. Ramelteon for the treatment of insomnia. Clin Ther 2006;28:1540-55. [Crossref] [PubMed]
- Wang J, Jiang C, Zhang K, et al. Melatonin receptor activation provides cerebral protection after traumatic brain injury by mitigating oxidative stress and inflammation via the Nrf2 signaling pathway. Free Radic Biol Med 2019;131:345-55. [Crossref] [PubMed]
- Usta Sofu G, Erzurumlu Y, Karaca U, et al. Melatonin receptor agonist ramelteon alleviates experimental acute ocular inflammation via HIF-1A/VEGF/E-NOS signaling. Eur J Ophthalmol 2022; Epub ahead of print. [Crossref] [PubMed]
- Imai S, Kadomura S, Miyai T, et al. Using Japanese big data to investigate novel factors and their high-risk combinations that affect vancomycin-induced nephrotoxicity. Br J Clin Pharmacol 2022;88:3241-55. [Crossref] [PubMed]
- Wu XL, Lu SS, Liu MR, et al. Melatonin receptor agonist ramelteon attenuates mouse acute and chronic ischemic brain injury. Acta Pharmacol Sin 2020;41:1016-24. [Crossref] [PubMed]
- Zhang Y, Liu M, Zhang Y, et al. Urolithin A alleviates acute kidney injury induced by renal ischemia reperfusion through the p62-Keap1-Nrf2 signaling pathway. Phytother Res 2022;36:984-95. [Crossref] [PubMed]
- Du Y, Chu CM, Zhuo D, et al. The inhibition of TRIM35-mediated TIGAR ubiquitination enhances mitochondrial fusion and alleviates renal ischemia-reperfusion injury. Int J Biol Macromol 2022;209:725-36. [Crossref] [PubMed]
- Liu Y, Wang L, Du N, et al. Ramelteon Ameliorates LPS-Induced Hyperpermeability of the Blood-Brain Barrier (BBB) by Activating Nrf2. Inflammation 2021;44:1750-61. [Crossref] [PubMed]
- Yang W, Zhang Y, Lu D, et al. Ramelteon protects against human pulmonary microvascular endothelial cell injury induced by lipopolysaccharide (LPS) via activating nuclear factor erythroid 2-related factor 2 (Nrf2)/heme oxygenase-1 (HO-1) pathway. Bioengineered 2022;13:1518-29. [Crossref] [PubMed]
- Erdem KTO, Bedir Z, Kuyrukluyildiz U, et al. Effect of tocilizumab on ischemia-reperfusion-induced oxido-inflammatory renal damage and dysfunction in rats. Exp Anim 2022;71:491-9. [Crossref] [PubMed]
- Al-Bataineh MM, Kinlough CL, Mi Z, et al. KIM-1-mediated anti-inflammatory activity is preserved by MUC1 induction in the proximal tubule during ischemia-reperfusion injury. Am J Physiol Renal Physiol 2021;321:F135-48. [Crossref] [PubMed]
- Kalpage HA, Bazylianska V, Recanati MA, et al. Tissue-specific regulation of cytochrome c by post-translational modifications: respiration, the mitochondrial membrane potential, ROS, and apoptosis. FASEB J 2019;33:1540-53. [Crossref] [PubMed]
- Thapa K, Singh TG, Kaur A. Targeting ferroptosis in ischemia/reperfusion renal injury. Naunyn Schmiedebergs Arch Pharmacol 2022;395:1331-41. [Crossref] [PubMed]
- Zhao M, Wang Y, Li L, et al. Mitochondrial ROS promote mitochondrial dysfunction and inflammation in ischemic acute kidney injury by disrupting TFAM-mediated mtDNA maintenance. Theranostics 2021;11:1845-63. [Crossref] [PubMed]
- Shen H, Holliday M, Sheikh-Hamad D, et al. Sirtuin-3 mediates sex differences in kidney ischemia-reperfusion injury. Transl Res 2021;235:15-31. [Crossref] [PubMed]
- McGechan A, Wellington K. Ramelteon. CNS Drugs 2005;19:1057-65; discussion 1066-7. [Crossref] [PubMed]
- Aslankoc R, Savran M, Doğuç DK, et al. Ameliorating effects of ramelteon on oxidative stress, inflammation, apoptosis, and autophagy markers in methotrexate-induced cerebral toxicity. Iran J Basic Med Sci 2022;25:1183-9. [PubMed]
- Xu H, Mu X, Ding Y, et al. Melatonin alleviates benzo(a)pyrene-induced ovarian corpus luteum dysfunction by suppressing excessive oxidative stress and apoptosis. Ecotoxicol Environ Saf 2021;207:111561. [Crossref] [PubMed]
- Kandezi N, Majdi F, Davoudizadeh R, et al. Preventive Properties of Ramelteon against Cocaine-Induced Autophagia and Apoptosis: A Hypothetic Role of TNF-α Receptor Involvement and JNK/Bcl-2-Beclin1 or Bcl-2/Bax Signaling Pathway. Int J Prev Med 2020;11:36. [Crossref] [PubMed]
- Wu GC, Peng CK, Liao WI, et al. Melatonin receptor agonist protects against acute lung injury induced by ventilator through up-regulation of IL-10 production. Respir Res 2020;21:65. [Crossref] [PubMed]
- Preiser JC. Oxidative stress. JPEN J Parenter Enteral Nutr 2012;36:147-54. [Crossref] [PubMed]
- Malek M, Nematbakhsh M. Renal ischemia/reperfusion injury; from pathophysiology to treatment. J Renal Inj Prev 2015;4:20-7. [PubMed]
- Tan X, Zhang L, Jiang Y, et al. Postconditioning ameliorates mitochondrial DNA damage and deletion after renal ischemic injury. Nephrol Dial Transplant 2013;28:2754-65. [Crossref] [PubMed]
- Iwata Y, Nakade Y, Kitajima S, et al. Protective effect of d-alanine against acute kidney injury. Am J Physiol Renal Physiol 2022;322:F667-79. [Crossref] [PubMed]
- Song YC, Liu R, Li RH, et al. Dexmedetomidine Exerts Renal Protective Effect by Regulating the PGC-1α/STAT1/IRF-1 Axis. Nephron 2021;145:528-39. [Crossref] [PubMed]
- Sen P, Helmke A, Liao CM, et al. SerpinB2 Regulates Immune Response in Kidney Injury and Aging. J Am Soc Nephrol 2020;31:983-95. [Crossref] [PubMed]
- Li Y, Xu B, Yang J, et al. Liraglutide protects against lethal renal ischemia-reperfusion injury by inhibiting high-mobility group box 1 nuclear-cytoplasmic translocation and release. Pharmacol Res 2021;173:105867. [Crossref] [PubMed]
- Awad AS, Elariny HA, Sallam AS. Colchicine attenuates renal ischemia-reperfusion-induced liver damage: implication of TLR4/NF-κB, TGF-β, and BAX and Bcl-2 gene expression. Can J Physiol Pharmacol 2022;100:12-8. [Crossref] [PubMed]
- Yang J, Liu H, Han S, et al. Melatonin pretreatment alleviates renal ischemia-reperfusion injury by promoting autophagic flux via TLR4/MyD88/MEK/ERK/mTORC1 signaling. FASEB J 2020;34:12324-37. [Crossref] [PubMed]
- Barati A, Rahbar Saadat Y, Meybodi SM, et al. Eplerenone reduces renal ischaemia/reperfusion injury by modulating Klotho, NF-κB and SIRT1/SIRT3/PGC-1α signalling pathways. J Pharm Pharmacol 2023;75:819-27. [Crossref] [PubMed]
- Yang S, Wang J, Wang D, et al. Melatonin Receptor Agonist Ramelteon Suppresses LPS-Induced Neuroinflammation in Astrocytes. ACS Chem Neurosci 2021;12:1498-505. [Crossref] [PubMed]