Addressing the need for preclinical study of penile prosthesis infection: a new animal model and narrative review
Introduction
Erectile dysfunction (ED) affects one-half of men aged 40–70 years, a third of whom will eventually fail conservative treatment (1,2). Penile prostheses (PPs), including inflatable PPs (IPPs) and semi-rigid prostheses, are the mainstay treatment for refractory ED, although only 5% of eligible patients receive them (3,4). The most feared complication following PP placement is device infection, which is associated with severe morbidity, pain, penile length loss, and financial costs (5,6). Infection often requires reoperation for PP removal, after which reimplantation may be difficult due to fibrosis (7).
Prior to the addition of antibiotic and hydrophilic coatings to IPPs, infection rates ranged from 5–9% (8,9). With the use of these coatings, infection rates have fallen but remain relatively stable at 0.5–3%; incidence is modulated by surgical technique, patient demographics, and implant type (5,10,11). Urologic societies have issued few guidelines for infection prevention in PP operations, and those that do exist contain significant variability, leading to inconsistency in clinical practice amongst providers (12-14).
Novel infection prevention strategies for PP infections may be explored in the laboratory setting. However, bacterial device colonization, biofilm formation, and clinical device infections are complicated phenomena that involve device interactions with the changing milieu of the surgical site, type of bacteria, type of antibiotic prophylaxis, and ultimately the immune system. Thus, while in vitro study is critical for scholarly understanding, modeling of PP infection is greatly limited without in vivo options.
Given the practical and ethical limitations of studying device infections in human patients, representative in vivo animal models are needed to better understand how to treat these devastating infections and provide crucial clinical feasibility and safety information. In this manuscript, we review previously reported in vivo PP studies and describe a novel rabbit model containing a functional IPP. We present this article in accordance with the Narrative Review reporting checklist (available at https://tau.amegroups.com/article/view/10.21037/tau-23-353/rc).
Methods
Literature review
A literature search was performed using the PubMed and Scopus databases following narrative review methodology (Table 1). All publications available through the search date (March 21, 2023) were considered. Inclusion criteria included publication in a peer-reviewed journal and study of PP while exclusion criteria included non-English publications, lack of preclinical in vivo models, or data derived from human subjects. Search terms included (‘penile implant’ OR ‘penile prosthesis’) AND (‘animal’ OR ‘in vivo’ OR ‘rabbit’ OR ‘rat’ OR ‘mouse’ OR ‘rodent’ OR ‘dog’ OR ‘canine’). Database search, result screening, and study selection were completed by three independent reviewers, with conflicting decisions resolved by majority. On final review, 12 papers from 1992–2021 were included in our review (Table 2).
Table 1
Items | Specification |
---|---|
Date of search | March 21, 2023 |
Databases and other sources searched | PubMed, Scopus |
Search terms used | (‘penile implant’ OR ‘penile prosthesis’) AND (‘animal’ OR ‘in vivo’ OR ‘rabbit’ OR ‘rat’ OR ‘mouse’ OR ‘rodent’ OR ‘dog’ OR ‘canine’) |
Timeframe | Before March 21, 2023 |
Inclusion and exclusion criteria | Inclusion criteria: publication in a peer-reviewed journal; study of penile prosthesis |
Exclusion criteria: human subjects, lacks preclinical animal model, non-English publication | |
Selection process | Three independent reviewers completed the search and study selection; conflicting decisions were resolved by majority |
Table 2
Study | Year | Model [n] | PI type | Findings |
---|---|---|---|---|
Paick et al. | 1992 | Dog [13] | Implantable penile venous compression device | Successful acute erection after venous occlusion |
Donatucci et al. | 1993 | Dog [14] | Implantable penile venous compression device | Successful device cycling without local injury or atrophy |
Knoll et al. | 1994 | Dog [6] | Inflatable cuff and pump reservoir | Mechanical reliability and efficacy without damage or venous thromboembolism risk |
Teichman et al. | 1994 | Rat [87] | Silicone pellet implant with protamine sulfate and vancomycin wound irrigation | Potentiation of vancomycin bactericidal effect |
Acar et al. | 2000 | Rat [45] | Silicone prosthesis section with irrigation and postoperative antibiotics | Reduction in S. epidermidis |
Darouiche et al. | 2002 | Rabbit [11] | Silicone pump bulb sections with minocycline/rifampin coating | Reduction in S. aureus |
Hellstrom et al. | 2003 | Rabbit [28] | Substrate discs with hydrophilic coating and gentamicin/bacitracin soak | Reduction in S. epidermidis |
Culha et al. | 2004 | Rat [45] | Silicone prosthesis section with S. epidermidis introduced into leg at 6 months post-implant | Hematogenous seeding is not responsible for prosthetic infection |
Rajpurkar et al. | 2004 | Rat [30] | Hydrophilic-coated polyurethane soaked in vancomycin/gentamicin | Reduction in S. epidermidis |
Arica et al. | 2008 | Rabbit [70] | Antibiotic-loaded hydrogel system with prosthesis | Reduction in infection risk |
Mansouri et al. | 2009 | Rabbit [8] | Uncoated, vancomycin-soaked hydrophilic-coated, and minocycline/rifampin-impregnated IPP cylinder segments | Inhibition of S. aureus with both products until day 2, but only with minocycline/rifampin product until day 14 |
Lima et al. | 2021 | Rabbit [30] | Intracavernous bacterial cellulose (semi-rigid) gel | Compatibility and biointegration of filling material |
Shah et al. | Present manuscript | Rabbit [4] | Functional Coloplast Titan IPP | Animal safety and device functionality |
PI, penile implant; IPP, inflatable penile prosthesis; S. epidermidis, Staphylococcus epidermidis; S. aureus, Staphylococcus aureus.
Animal model
Our study involved placement of functioning Coloplast Titan® IPPs (Coloplast, Minneapolis, MN, USA) into the flanks of two cadaveric and two live male NZW rabbits (6–12 months, 4–5 kg; Charles River, Wilmington, MA, USA). The flanks were chosen due to the overall size of IPPs. Cadaveric rabbits were utilized to evaluate surgical technique and ultrasound parameters prior to the in vivo study. Live rabbits were premedicated with ketamine 30–40 mg/kg, xylazine 3–5 mg/kg, and acepromazine 0.25–1 mg/kg via intubation with 1–4% isoflurane. Following anesthesia and surgical site preparation, dissection was performed down to the plane between the panniculus carnosus and underlying muscle fascia. A subcutaneous pouch was developed to accommodate the implant. For each animal, one functioning implant, including a single 14 cm cylinder, pump, and 75 mL Cloverleaf reservoir, was placed.
Following implantation, unrestricted ambulation was permitted, and the rabbits were observed for activity and recovery. Wounds were inspected daily for drainage, erythema, warmth, and swelling. IPP cycling and device imaging using an Aplio i800 scanner (Canon Medical Systems, Tustin, CA, USA) were performed on post-implantation day 3 under isoflurane nose cone sedation. Surveillance was conducted for 14 days, after which the animals were euthanized via pentobarbital 0.22–0.44 mL/kg. Primary endpoints included clinical signs of pain, sepsis, mobility, and ability to thrive. Signs of pain or distress were defined as gait disturbance, hypoactivity, restlessness, weight loss, dehydration, or reduced eating/drinking.
Experiments were performed under a project license granted by the Institutional Board of Thomas Jefferson University in compliance with national guidelines for the care and use of animals. Experimental protocols were approved by the Institutional Animal Care and Use Committee (No. 21-08-418).
Results
Early studies evaluating ED devices
Venous compression devices
The earliest study in our review, performed by Paick et al. in 1992, reported the initial design of an inflatable venous compression device which was placed in 13 dogs and produced acute erection via temporary venous outflow occlusion (15). Two pioneering studies in 1993 and 1994, performed by Donatucci et al. and Knoll et al., respectively, reported successful placement of this device in the canine penis and demonstrated safety and erectile functionality (16,17). These studies are regarded as the earliest demonstrations of a feasible implant-based ED treatment in animals.
Donatucci et al. surgically placed a prosthetic inflatable venous compression device at the penile base in 14 dogs to assess functionality and chronic effects on local tissue (16). Successful intracavernous pressure elevation following neurostimulation was demonstrated, and neural injury, vascular compromise, or local tissue atrophy were not observed over a 7-month span. Knoll et al. implanted the cuff and pump reservoir from an inflatable cavernosal compression device in six male dogs (17). Implantation was completed around the corpus cavernosum near the crura and excluded the corpus spongiosum. Cycling for 2 months showed mechanical functionality without resulting chronic pathology in penile tissue. These dog models allowed functional testing, but their use has been limited. Importantly, canine models lack the low cost, ethical considerations, and practical nature of smaller animal models. More recent canine or non-canine animal models of functional IPP implantation eluded our searches.
Overall, surgical placement of venous compression devices is not utilized in modern clinical practice; one reason is their restriction to treating vascular ED, with little utility in patients with ED of metabolic, neurologic, or psychologic etiologies. Iatrogenic venous compression also holds theoretical concern of thromboembolic events, though this has not been reported in penile devices in the literature (18,19).
Injectable semi-rigid PP
The only in vivo report of an injectable semi-rigid prosthesis within our review was published by Lima et al. in 2021. Authors injected bacterial cellulose gel, which would act similarly to a malleable prosthesis but permit injection in lieu of surgical implantation, into the corpora cavernosa of 30 New Zealand White (NZW) rabbits. The study demonstrated gel biocompatibility and biointegration for 6 months (20). Four injections were performed within a 1-week interval, 3 weeks after bilateral orchiectomy to eliminate erectile function. Previous researchers had reported use of bacterial cellulose gel with sugarcane molasses substrate as a stable filling agent in several contexts outside ED; this gel leads to immediate erection from mass effect and eventual incorporation within host tissue (20).
Early infection control experiments
As urologists better appreciated the risks of prosthetic implantation, efforts were made to utilize animal models to develop techniques to mitigate infection. In 1994, Teichman et al. explored the use of protamine sulfate irrigation, aiming to increase the antibiotic activity of vancomycin. Their study implanted a silicone pellet inoculated with Staphylococcus epidermidis (S. epidermidis) subcutaneously in the dorsum of 87 rats. When the pellets were explanted and cultured after 28 days, the authors found that infections were reduced from 77% to 50% with vancomycin irrigant alone, and to 19% with combined irrigation using vancomycin and protamine sulfate (21).
Acar et al. placed silicone prosthesis pellets, which had been incubated with S. epidermidis for 24 hours in tryptic soy broth, in the scrotum of 45 rats to compare infection rates. They followed three groups for 20 days: teicoplanin/ofloxacin systemically via injection, intraoperative irrigation with teicoplanin/amikacin solution, and a control group with no antibiotic treatment. Both injection and irrigation were found to reduce S. epidermidis growth over 20 days, ranging from 86.7% in the control group to 33.3% in the systemic treatment group and 13.3% in the irrigation treatment group (22). However, it is important to note that as appreciation of antibiotic stewardship evolves, recent clinical studies have argued against postoperative antibiotics unless particular risk factors are identified, as the benefits to their use are limited (23).
Development of antibiotic-coated PPs
Because of unpredictable IPP infection rates, antibiotic coatings for prosthetics were proposed. These coatings were designed to achieve high local concentrations during the critical post-operative period in the hopes of reducing infection rates. This local effect posed an advantage over a prolonged course of systemic antibiotics. Specific antibiotic and coating material selection have evolved as preclinical and clinical studies were performed and microbiological profiles changed.
Today, there are two coated IPPs on the market: Boston Scientific AMS 700TM with InhibiZoneTM (Marlborough, MA, USA) and Coloplast Titan® with HydroVANTAGETM (Minneapolis, MN, USA). First introduced in 2000, the InhibiZoneTM coating contains minocycline/rifampin and is applied directly onto the implant. The HydroVANTAGETM hydrophilic coating was introduced on a silicone and Bioflex® biopolymer material in 2002 with an expectation that antibiotics could be readily adsorbed to the surface (5,24). These developments were closely linked to the following studies by Darouiche et al. and Hellstrom et al.
Darouiche et al. incubated InhibiZoneTM coated silicone sections of IPP pump bulbs with Staphylococcus aureus (S. aureus) and implanted them in 11 rabbits (25). They compared InhibiZoneTM to unimpregnated sections 2 days postoperatively, finding that S. aureus colonization was reduced sixfold when devices were retrieved and organisms recovered using sonication followed by culturing.
Hellstrom and colleagues studied the efficacy of HydroVANTAGETM hydrophilic coating, proposing it could retard bacterial adherence and allow operators to absorb appropriate antibiotics that would subsequently elute. Bioflex® substrate discs coated with HydroVANTAGETM were soaked in a gentamicin/bacitracin solution, followed by subcutaneous implantation in rabbits (26). Researchers explanted the discs at regular time points. Antibiotic remaining on the disc was measured by the area of zones of inhibition associated with antibiotic elution onto a microorganism-seeded agar plate. Using this disc, S. epidermidis growth was inhibited over a 3-day study period. These two studies provided valuable data on present-day IPP antibiotic selection and supported the marketing of antibiotic- and hydrophilic-coated IPPs.
A later study performed by Mansouri et al. used a similar methodology in rabbits where the antibiotic and hydrophilic coatings were compared to each other. Although both coatings effectively reduced S. aureus growth, minocycline/rifampin impregnation of InhibiZoneTM was significantly more likely to result in 14-day growth inhibition than the vancomycin-dipped HydroVANTAGETM material. The zone of inhibition was also much larger with the former product, and the authors concluded that this option may result in broader spectrum, more durable antimicrobial activity, in addition to its increased practicality for surgeons (10). Although this is one of the stronger studies included in our review, the authors appraised the zone of inhibition identified in their experimental results based on previously reported drug-eluting urethral catheter studies (10,27). The urethral catheter studies identified a zone of inhibition of ≥10–15 mm as the benchmark needed to reduce clinical catheter-associated urinary tract infection. This zone of inhibition was then translated into the authors’ IPP study as the zone of inhibition needed to reduce penile implant infection. Notably, this zone of inhibition benchmark was only exceeded until day 2 of the 14-day IPP study.
Evolution of antibiotics for PPs
InhibiZoneTM was shown to limit bacterial colonization, particularly from Staphylococcus (7,28). This came after testing in vitro, in animal models, and finally in clinical settings (24). With HydroVANTAGETM, surgeons could personalize antibiotics to individualized risk factors, patient allergies, and evolving local antibiograms. However, the HydroVANTAGETM flexibility in antibiotic choice also limits standardization and relies on individual surgeon gestalt and experience. Most common antibiotic choices include rifampin/gentamicin or vancomycin/gentamicin. Adsorption of 0.05% chlorhexidine gluconate solution has also been reported due to its broad-spectrum action against bacteria, fungi, and viruses (29).
Using vancomycin/gentamicin, Rajpurkar et al. reported a study of the benefits of polyvinylpyrrolidone hydrophilic coating on Bioflex® in 2004. In their study, Bioflex® strips were incubated in a S. epidermidis suspension for 10 minutes followed by subcutaneous implantation in the flanks of 60 rats. They concluded that the coating reduced bacterial count by 55% over 7 days (30).
To further enhance antimicrobial prophylaxis, Arica et al. [2008] studied antibiotic-loaded hydrogel as a drug delivery tool on PP. In their study, hydroxyethylmethacrylate and poly(ethylene glycol)-methacrylate copolymer PPs were placed in the corpus cavernosum and inoculated with S. aureus and Escherichia coli (E. coli). They loaded three antibiotics (ceftriaxone, vancomycin, and gentamicin) with the hydrogel and investigated microbial culture and antibiotic susceptibility one month after implantation via clinical, histopathological, and microbiological assessment of infection. They found merit to using this method in lieu of parenteral antibiotics in terms of clinical signs of infection and bacterial count (8). Particularly, they demonstrated that although parenteral antibiotics were effective, hydrogel-loaded antibiotics may achieve higher concentrations and successfully prevent infection. This was an important study published years after the introduction of the first hydrophilic coating, aimed at further investigating the optimal way to deliver antibiotics.
It must be noted that although these coatings were introduced approximately two decades ago, there has been significant shift in infection sources without a corresponding adaptation in clinical practice. Traditionally, infections largely arose from S. epidermidis and S. aureus, while gram-negative species including E. coli, Serratia spp., and Proteus mirabilis (P. mirabilis) were common secondary culprits (14,28,31,32). Contemporary literature shows a shift towards gram-negative and fungal species that may not be covered using traditional antibiotic strategies (28,32). The American Urological Association (AUA) has now added aminoglycosides in response to this shift in bacterial species (9). Yet a 2017 study found that AUA and European Association of Urology guidelines did not cover responsible bacteria in 14–38% of cases (14). The most recent study, performed in 2023, broadly found that AUA recommendations do not accomplish their goal of infection reduction (33). Despite the antibiotic coatings and an improved understanding of causative organisms, patients continue to face infections.
Mechanisms of PP infection
Understanding of the mechanisms of PP infection has evolved over time because of preclinical and clinical studies. Surgical contamination via microbial entry through an open wound, is considered the most common origin of infection, and several preparation techniques and surgical strategies have reduced infection rates (34). Hematogenous seeding has rarely been reported and is typically attributed to late PP infections which can occur greater than 8 months after surgery (35).
Our review identified only one study, completed by Culha et al., which studied pathophysiology of PP infection. Their study, in 45 rats which received small pieces of silicone implants within their scrotum for 6 months, involved placement of S. epidermidis-infected discs in the leg to resemble a thigh abscess. While some rats demonstrated positive blood cultures, none experienced an implant infection. The authors suggested that hematogenous seeding does not represent a significant mechanism for PP infection (36). Of note, though, S. epidermidis is a relatively indolent microorganism, and it is possible that colonization may have occurred without infection. Though this study offers one model of hematogenous spread, there are various other hypotheses and treatment prospects which remain untested.
Contemporary rabbit model for IPP
We present a new study utilizing the NZW rabbit with intact IPP placement subcutaneously in the flank. All rabbits remained viable after implantation and IPPs were fully functional upon radiologic evaluation, indicating that this model may be utilized for further study. Following a 3-day post-implantation recovery period, IPP cycling mimicking the inflation protocol was successfully completed. All IPP components were successfully visualized via ultrasound, which demonstrated the superficial position of the implant and confirmed proper inflation-deflation cycling (Figure 1). Over the 14-day observation period, no concerns regarding the primary endpoints were identified, and signs of pain or distress were not observed. Our study supports the feasibility of functional penile implants placed into the flanks of the rabbits, and further, the lack of redness and maintenance of mobility suggest that the NZW rabbit exhibits good tolerance to this placement. This is the first report of an in vivo PP model which may facilitate preclinical study of the functionality and interactions of IPPs with novel infection control methods outside of the pioneering canine studies in the twentieth century, which used early compression devices.
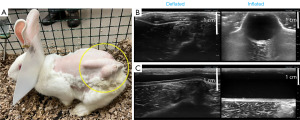
Discussion
Although antibiotic coatings for IPPs exist, there is a need for further innovation to reduce infection rates. Based on our review, functional PP placement into an animal has not been described in over two decades. The earliest published studies described placement of functional venous compression devices and were limited to canines. All other studies included in our review used only partial sections of PPs and involved locations outside the penis. Therefore, they may not capture potential mechanical and functional factors, possibly compromising representativeness and scalability to the complete, larger device. Similarly, although in vitro laboratory experiments may certainly elucidate how antibiotic coatings are affected by the mechanics of device inflation or their biochemical interactions with the implant material, in vivo testing is ultimately necessary to reliably study infection control in an environment that most closely represents patients. While the studies all spoke to the ability of immobilized antibiotics to lessen bacterial colonization, the effects were modest, and ultimately the AUA recommendations for infection control appear to be inadequate. We believe that limitation in the availability of in vivo models explains the lack of other mechanistic studies of PP infection within our review.
To facilitate more rigorous in vivo research, we have proposed implantation of a functional IPP in the flank of rabbits. The NZW rabbit is economical, conveniently handled, and has an appropriate lifespan for IPP-related studies. Subcutaneous medical device placement in rabbits has been previously performed in other settings. A study comparing implanted dermal matrices and polypropylene mesh for pelvic floor dysfunctions found numerous advantages to using NZW rabbits in comparison to those using sheep, pigs, and dogs. The authors outlined practical and ethical concerns with larger animal models (37). Another study implanted cardiac pacemakers into rabbit backs to study infection rates and control strategies (38). More generally, the utility of rabbit models in evaluating efficacy, safety, and infection rates for various medical devices is well-established. Nonetheless, considering the uniqueness of penile implants in comparison to other medical devices, a proof-of-concept study was necessary to justify future use of this model with a working IPP.
Future uses of in vivo PP models
PP infection rates remain clinically significant. Although there are several proposed solutions to reduce this feared complication, representative preclinical testing is necessary before any introduction into patients. For instance, in vitro studies have explored a variety of genitourinary device coatings, including different antibiotic combinations, nanoparticles, and elemental metals (39-41). Our new model appears to be the sole example of modern, functional IPP placement in vivo.
Importantly, normal infection may take several weeks, while our study endpoint was at 14 days. However, our primary goal was not to evaluate the normal infectious process, and no infections were observed, but instead to create a model which includes a functional IPP for future evaluation of early biofilm formation. Over time, this model may be used to induce infection at the time of surgery and subsequently evaluate new strategies to fight infection in the early post-operative period.
This model with a functioning implant may allow exploration of new ways to treat infection. Investigators may consider new perioperative antibiotic strategies including PP coatings which specifically attack evolving microorganism profiles or elute greater concentrations of antibiotics (24). Safety of new drug combinations or hydrophilic coatings for antibacterial functionality may be tested.
Furthermore, we may glean an improved understanding of infectious mechanisms and biofilm, allowing modern PPs which limit infection through evolving design, texture, or inherent biomaterials. For instance, Staphylococcus spp., particularly S. epidermidis, produce biofilms which extend survival and increase bacterial tolerance to anti-infective strategies. Moreover, biofilm bacteria are sequestered within a matrix and adherent to tissues/implants, which may explain why one-third of PP infections yield negative cultures (42,43). Because of this adherent state, PP infections are currently treated by device explantation or irrigation. High-risk patients may require additional prophylaxis to prevent biofilm formation (8). Of note, we did not evaluate capsule formation upon device explantation. This represents an important area for future study as studies of other implants, particularly in the breast, have shown variance in encapsulation (44,45).
Working with a functioning implant may help to understand antibiotic coating efficacy and biofilm prevention as a function of the different parts of the device. For example, dynamic surfaces, such as elicited in shape memory polymers, may decrease bacterial adhesion and increase antibiotic sensitivity (46-49). This type of surface deformation has been applied to urinary catheters through inflation and deflation of small intra-wall lumens for E. coli and P. mirabilis removal to reduce urinary tract infections and may be translatable to IPP (48).
In addition, this model provides an opportunity to evaluate the role of capsule formation, which has been theorized to harbor bacteria from the systemic circulation and immune response. The utility of various perioperative infection control measures may be further analyzed as well (50). It has been proposed that treatment with signal inhibitors may block biofilm formation (24,51). Mechanical disruption using microbubbles and contrast-enhanced ultrasound imaging can hydrodynamically interfere with biofilm interfaces on surrounding fluid and tissue (51). We anticipate that these and many more infection control strategies will be developed as the field moves forward.
Finally, this model may also further appreciation of patient-specific factors and comorbidities modulating IPP infection when utilized in combination with disease-specific models. While the study of risk factors has been limited to conflicting studies of small, retrospective patient cohorts, a preclinical in vivo model may allow manipulation of proposed variables to improve understanding of risk stratification and preventative strategies (50).
Strengths and limitations
This manuscript has several strengths. In terms of the review of the literature, a validated narrative review methodology was followed, and three independent reviewers conducted study selection. Limitations include the inability to perform further data compilation and statistical analysis, as the number of available studies is low and study endpoints are diverse. Relevant non-English studies may have been missed.
Although our rabbit model offers a new in vivo option, there are limitations. Namely, this study involved a limited sample size, and long-term integrity and safety are unclear, as data was only collected for 14 days. Hence, our use of this model was limited to study of peri-operative mechanisms. Additionally, only the safety and tolerability of IPP placement was evaluated, not the safety and efficacy in a setting of IPP infection, which is the next phase of developing this model. It bears noting that there are differences between rabbit and human microbiomes, limiting direct extrapolation of results from any animal study to clinical use. Finally, placement in the flank using a subcutaneous pocket limits comparisons with penile placement, as there are differences in blood flow and tissue apposition.
Conclusions
We review a range of previously reported studies, finding that contemporary in vivo PP models are lacking. We also present a new rabbit model which may prove effective in evaluating novel infection prevention strategies following penile implantation for ED. Preclinical in vivo models with an intact implant such as ours may serve as a representative, efficient, and practical way to test risk factors and preventative strategies for PP infection. Ultimately, improved infection-control strategies would be highly beneficial in mitigating morbidity and improving patient experiences.
Acknowledgments
This work was supported by Coloplast (material support) to P.H.C.
Funding: None.
Footnote
Provenance and Peer Review: This article was commissioned by the editorial office, Translational Andrology and Urology, for the series “Genitourinary Prosthesis Infection”. The article has undergone external peer review.
Reporting Checklist: The authors have completed the Narrative Review reporting checklist. Available at https://tau.amegroups.com/article/view/10.21037/tau-23-353/rc
Peer Review File: Available at https://tau.amegroups.com/article/view/10.21037/tau-23-353/prf
Conflicts of Interest: All authors have completed the ICMJE uniform disclosure form (available at https://tau.amegroups.com/article/view/10.21037/tau-23-353/coif). The series “Genitourinary Prosthesis Infection” was commissioned by the editorial office without any funding or sponsorship. P.H.C. served as the unpaid Guest Editor of the series and serves as an unpaid editorial board member of Translational Andrology and Urology. P.H.C. consults for and receives research support from Coloplast and Boston Scientific. C.E.W. is a clinical consultant for Bracco Diagnostics and Canon Medical Systems USA. N.J.H. consults for SINTX technologies. F.F. receives research support from Bracco, the Butterfly Network, Canon Medical Systems USA, GE HealthCare, Lantheus Medical Imaging and Siemens Healthineers. He is also a consultant or advisor for Exact Therapeutics, Lantheus, Longeviti Neuro Solutions and Sonothera. The authors have no other conflicts of interest to declare.
Ethical Statement: The authors are accountable for all aspects of the work in ensuring that questions related to the accuracy or integrity of any part of the work are appropriately investigated and resolved. Experiments were performed under a project license granted by the institutional board of Thomas Jefferson University in compliance with national guidelines for the care and use of animals. Experimental protocols were approved by the Institutional Animal Care and Use Committee (No. 21-08-418).
Open Access Statement: This is an Open Access article distributed in accordance with the Creative Commons Attribution-NonCommercial-NoDerivs 4.0 International License (CC BY-NC-ND 4.0), which permits the non-commercial replication and distribution of the article with the strict proviso that no changes or edits are made and the original work is properly cited (including links to both the formal publication through the relevant DOI and the license). See: https://creativecommons.org/licenses/by-nc-nd/4.0/.
References
- Kim S, Cho MC, Cho SY, et al. Novel Emerging Therapies for Erectile Dysfunction. World J Mens Health 2021;39:48-64. [Crossref] [PubMed]
- Hatzimouratidis K, Hatzichristou D. Phosphodiesterase type 5 inhibitors: the day after. Eur Urol 2007;51:75-88; discussion 89. [Crossref] [PubMed]
- Rodriguez KM, Kohn TP, Davis AB, et al. Penile implants: a look into the future. Transl Androl Urol 2017;6:S860-6. [Crossref] [PubMed]
- Lee DJ, Najari BB, Davison WL, et al. Trends in the Utilization of Penile Prostheses in the Treatment of Erectile Dysfunction in the United States. J Sex Med 2015;12:1638-45. [Crossref] [PubMed]
- Wang VM, Levine LA. Safety and Efficacy of Inflatable Penile Prostheses for the Treatment of Erectile Dysfunction: Evidence to Date. Med Devices (Auckl) 2022;15:27-36. [Crossref] [PubMed]
- Baird BA, Parikh K, Broderick G. Penile implant infection factors: a contemporary narrative review of literature. Transl Androl Urol 2021;10:3873-84. [Crossref] [PubMed]
- Chung E. Penile prosthesis implant: scientific advances and technological innovations over the last four decades. Transl Androl Urol 2017;6:37-45. [Crossref] [PubMed]
- Arica MY, Tuğlu D, Başar MM, et al. Preparation and characterization of infection-resistant antibiotics-releasing hydrogels rods of poly[hydroxyethyl methacrylate-co-(poly(ethylene glycol)-methacrylate]: biomedical application in a novel rabbit penile prosthesis model. J Biomed Mater Res B Appl Biomater 2008;86:18-28. [Crossref] [PubMed]
- Dropkin BM, Kaufman MR. Antibiotics and Inflatable Penile Prosthesis Insertion: A Literature Review. Sex Med Rev 2021;9:174-80. [Crossref] [PubMed]
- Mansouri MD, Boone TB, Darouiche RO. Comparative assessment of antimicrobial activities of antibiotic-treated penile prostheses. Eur Urol 2009;56:1039-45. [Crossref] [PubMed]
- Pan S, Rodriguez D, Thirumavalavan N, et al. The Use of Antiseptic Solutions in the Prevention and Management of Penile Prosthesis Infections: A Review of the Cytotoxic and Microbiological Effects of Common Irrigation Solutions. J Sex Med 2019;16:781-90. [Crossref] [PubMed]
- Katz DJ, Stember DS, Nelson CJ, et al. Perioperative prevention of penile prosthesis infection: practice patterns among surgeons of SMSNA and ISSM. J Sex Med 2012;9:1705-12; quiz 712-4. [Crossref] [PubMed]
- Wosnitzer MS, Greenfield JM. Antibiotic patterns with inflatable penile prosthesis insertion. J Sex Med 2011;8:1521-8. [Crossref] [PubMed]
- Gross MS, Phillips EA, Carrasquillo RJ, et al. Multicenter Investigation of the Micro-Organisms Involved in Penile Prosthesis Infection: An Analysis of the Efficacy of the AUA and EAU Guidelines for Penile Prosthesis Prophylaxis. J Sex Med 2017;14:455-63. [Crossref] [PubMed]
- Paick JS, Marc B, Suh JK, et al. Implantable penile venous compression device: initial experience in the acute canine model. J Urol 1992;148:188-91. [Crossref] [PubMed]
- Donatucci CF, Trigo-Rocha F, Paick JS, et al. Implantable penile venous compression device: initial experience in the chronic canine model. J Urol 1993;149:1152-5. [Crossref] [PubMed]
- Knoll LD, Benson RC Jr, Furlow WL, et al. Inflatable cavernosal body device: feasibility and acute safety study in the canine model. Urology 1994;44:143-8. [Crossref] [PubMed]
- Mathur M, Shafi I, Alkhouli M, et al. Surgical hardware-related iatrogenic venous compression syndrome. Vasc Med 2015;20:162-7. [Crossref] [PubMed]
- Shiraev T, Graham A. Venous thromboembolism secondary to penile prosthesis. Ann Vasc Surg 2014;28:1934.e1-2. [Crossref] [PubMed]
- Lima SVC, Chagas HM, Monteiro CCP, et al. Injectable semi rigid penile prosthesis: study in rabbits and future perspectives. Transl Androl Urol 2021;10:841-50. [Crossref] [PubMed]
- Teichman JM, Abraham VE, Stein PC, et al. Protamine sulfate and vancomycin are synergistic against Staphylococcus epidermidis prosthesis infection in vivo. J Urol 1994;152:213-6. [Crossref] [PubMed]
- Acar O, Mutlu B, Cimen K, et al. The role of intraoperative antibiotic irrigation and postoperative antibiotic therapy for contaminated implantable prosthesis: in a rat model in vivo. Int J Impot Res 2000;12:285-8. [Crossref] [PubMed]
- Dropkin BM, Chisholm LP, Dallmer JD, et al. Penile Prosthesis Insertion in the Era of Antibiotic Stewardship-Are Postoperative Antibiotics Necessary? J Urol 2020;203:611-4. [Crossref] [PubMed]
- Muench PJ. Infections versus penile implants: the war on bugs. J Urol 2013;189:1631-7. [Crossref] [PubMed]
- Darouiche RO, Mansouri MD, Raad II. Efficacy of antimicrobial-impregnated silicone sections from penile implants in preventing device colonization in an animal model. Urology 2002;59:303-7. [Crossref] [PubMed]
- Hellstrom WJ, Hyun JS, Human L, et al. Antimicrobial activity of antibiotic-soaked, Resist-coated Bioflex. Int J Impot Res 2003;15:18-21. [Crossref] [PubMed]
- Raad I, Darouiche R, Hachem R, et al. The broad-spectrum activity and efficacy of catheters coated with minocycline and rifampin. J Infect Dis 1996;173:418-24. [Crossref] [PubMed]
- Dinerman BF, Telis L, Eid JF. New Advancements in Inflatable Penile Prosthesis. Sex Med Rev 2021;9:507-14. [Crossref] [PubMed]
- Griggs R, Karpman E, Jones L, et al. Effect of 0.05% chlorhexidine gluconate in water on the hydrophilic inflatable penile prosthesis: biocompatibility, adherence, and dip time. J Sex Med 2023;20:113-7. [Crossref] [PubMed]
- Rajpurkar A, Fairfax M, Li H, et al. Antibiotic soaked hydrophilic coated bioflex: a new strategy in the prevention of penile prosthesis infection. J Sex Med 2004;1:215-20. [Crossref] [PubMed]
- Chung PH, Leong JY, Phillips CD, et al. Microorganism Profiles of Penile Prosthesis Removed for Infection, Erosion, and Mechanical Malfunction Based on Next-Generation Sequencing. J Sex Med 2022;19:356-63. [Crossref] [PubMed]
- Lokeshwar SD, Bitran J, Madhusoodanan V, et al. A Surgeon's Guide to the Various Antibiotic Dips Available During Penile Prosthesis Implantation. Curr Urol Rep 2019;20:11. [Crossref] [PubMed]
- Barham DW, Gross MS, Lentz AC, et al. AUA-recommended Antibiotic Prophylaxis for Primary Penile Implantation Results in a Higher, Not Lower, Risk for Postoperative Infection: A Multicenter Analysis. J Urol 2023;209:850-1. Reply. [Crossref] [PubMed]
- Mulcahy JJ. Current approach to the treatment of penile implant infections. Ther Adv Urol 2010;2:69-75. [Crossref] [PubMed]
- Carson CC, Robertson CN. Late hematogenous infection of penile prostheses. J Urol 1988;139:50-2. [Crossref] [PubMed]
- Culha M, Acar O, Mutlu B, et al. Prosthesis culture 6 months after implantation and the effect of haematogenous seeding in a rat model. Int J Impot Res 2004;16:231-4. [Crossref] [PubMed]
- Peró M, Casani L, Castells-Sala C, et al. Rabbit as an animal model for the study of biological grafts in pelvic floor dysfunctions. Sci Rep 2021;11:10545. [Crossref] [PubMed]
- Hansen LK, Brown M, Johnson D, et al. In vivo model of human pathogen infection and demonstration of efficacy by an antimicrobial pouch for pacing devices. Pacing Clin Electrophysiol 2009;32:898-907. [Crossref] [PubMed]
- Yang BY, Deng GY, Zhao RZ, et al. Porous Se@SiO2 nanosphere-coated catheter accelerates prostatic urethra wound healing by modulating macrophage polarization through reactive oxygen species-NF-κB pathway inhibition. Acta Biomater 2019;88:392-405. [Crossref] [PubMed]
- Towe M, Huynh LM, Osman MM, et al. Impact of Antimicrobial Dipping Solutions on Postoperative Infection Rates in Patients With Diabetes Undergoing Primary Insertion of a Coloplast Titan Inflatable Penile Prosthesis. J Sex Med 2020;17:2077-83. [Crossref] [PubMed]
- Cazalini EM, Miyakawa W, Teodoro GR, et al. Antimicrobial and anti-biofilm properties of polypropylene meshes coated with metal-containing DLC thin films. J Mater Sci Mater Med 2017;28:97. [Crossref] [PubMed]
- Dawn LE, Henry GD, Tan GK, et al. Biofilm and Infectious Agents Present at the Time of Penile Prosthesis Revision Surgery: Times Are a Changing. Sex Med Rev 2017;5:236-43. [Crossref] [PubMed]
- Welliver RC Jr, Hanerhoff BL, Henry GD, et al. Significance of biofilm for the prosthetic surgeon. Curr Urol Rep 2014;15:411. [Crossref] [PubMed]
- Santanelli di Pompeo F, Sorotos M, Canese R, et al. Study of the Effect of Different Breast Implant Surfaces on Capsule Formation and Host Inflammatory Response in an Animal Model. Aesthet Surg J 2023;43:506-15. [Crossref] [PubMed]
- Bayston R. Capsule formation around breast implants. JPRAS Open 2021;31:123-8. [Crossref] [PubMed]
- Gu H, Lee SW, Buffington SL, et al. On-Demand Removal of Bacterial Biofilms via Shape Memory Activation. ACS Appl Mater Interfaces 2016;8:21140-4. [Crossref] [PubMed]
- Lee SW, Gu H, Kilberg JB, et al. Sensitizing bacterial cells to antibiotics by shape recovery triggered biofilm dispersion. Acta Biomater 2018;81:93-102. [Crossref] [PubMed]
- Levering V, Cao C, Shivapooja P, et al. Urinary catheter capable of repeated on-demand removal of infectious biofilms via active deformation. Biomaterials 2016;77:77-86. [Crossref] [PubMed]
- Shivapooja P, Wang Q, Orihuela B, et al. Bioinspired surfaces with dynamic topography for active control of biofouling. Adv Mater 2013;25:1430-4. [Crossref] [PubMed]
- Isguven S, Chung PH, Machado P, et al. Minimizing Penile Prosthesis Implant Infection: What Can We Learn From Orthopedic Surgery? Urology 2020;146:6-14. [Crossref] [PubMed]
- Leong JY, Capella CE, D'Amico MJ, et al. A scoping review of penile implant biofilms-what do we know and what remains unknown? Transl Androl Urol 2022;11:1210-21. [Crossref] [PubMed]