Prenatal DEHP exposure induces lifelong testicular toxicity by continuously interfering with steroidogenic gene expression
Highlight box
Key findings
• The lifelong testicular toxicity of prenatal di-(2-ethylhexyl) phthalate (DEHP) exposure.
• Interfering with steroidogenic gene expression is the critical toxicological mechanism.
What is known and what is new?
• Prenatal DEHP exposure induced testicular toxicity.
• The lifelong testicular toxicity and continuous interference of steroidogenic gene expression prenatal DEHP exposure are presented in this study.
What is the implication, and what should change now?
• This study indicates the association between prenatal exposure and disorders of sex development, adult male disorders, and reproductive aging, thus preventing prenatal exposure is worthy of attention.
Introduction
The treatment of disorders of sex development (DSD) and adult male disorders (infertility, low testosterone) is full of challenges with poor therapeutic effects (1,2). More and more epidemiologic studies suggest that prenatal exposure to environmental endocrine disruptors (EEDs) is associated with lifelong testicular toxicity, such as DSD, adult male disorders, and even reproductive aging (3-5). However, the evidence is still weak, and the animal study that confirms the lifelong testicular toxicity of prenatal exposure is absent.
Di-(2-ethylhexyl) phthalate (DEHP), the most widely used plasticizer, is a typical EED. Mono-(2-ethylhexyl) phthalate (MEHP) is the main bioactive metabolite of DEHP (6). Previous studies have shown that prenatal DEHP exposure affected the puberal development of the genital system, adult reproduction (poor semen quality and damaged testicular histopathology), and serum testosterone of aged mice (7-10). Therefore, prenatal DEHP exposure may lead to lifelong testicular toxicity, but the evidence is not strong enough. It is essential to determine the lifelong testicular toxicity of prenatal DEHP exposure through animal study (I) as an experimental model to explain the long-term impacts (DSD, infertility, low testosterone, and reproductive aging) of prenatal exposure to EEDs in humans; (II) to attach importance to the prevention of prenatal exposure.
Prenatal DEHP/MEHP exposure reduced serum testosterone in mice offspring (10-13). However, the underlying mechanism of inhibiting testosterone synthesis is unclear. Testosterone is mainly synthesized by testicular Leydig cells (LCs) (14). Testosterone synthesis is a sequential process involving many enzymes to convert cholesterol into testosterone (15). The initial enzymes of testosterone synthesis include cytochrome P450 family 11 subfamily A member 1 (CYP11A1), cytochrome P450 family 17 subfamily A member 1 (CYP17A1), hydroxy-delta-5-steroid dehydrogenase, 3 beta- and steroid delta-isomerase 2 (HSD3B2) and (hydroxysteroid 17-beta dehydrogenase 3 (HSD17B3) (15), whose activity and expression would be impacted by DEHP and MEHP (16-18). Luteinizing hormone/human chorionic gonadotropin receptor (LHCGR) receives the signal of luteinizing hormone/human chorionic gonadotropin (LH/HCG) and then initiates testosterone synthesis of LCs (19). Steroidogenic acute regulatory (STAR) promotes the transfer of cholesterol into the mitochondria of LCs (20,21). Some EEDs reduce testosterone synthesis by interfering with the expression of LHCGR and STAR (22,23). Therefore, the interference of steroidogenic gene expression may be the main mechanism of testosterone synthesis inhibition by prenatal DEHP exposure.
Previous studies mainly used quantitative polymerase chain reaction (qPCR) detection to explore the interference of steroidogenic gene expression of prenatal DEHP exposure. Still, the results were sometimes contradictory and may be attributed to individual variation (16-18,20,21). In addition, these results were rarely verified by Western blot (WB) analysis. In this study, we not only utilized qPCR and WB analysis simultaneously, but also explored testosterone synthesis and steroidogenic gene expression in the neonatal testis and TM3 cells to verify mechanism. It was considered that the testicular toxicity of DEHP was dose-dependent, and exposure to different doses of DEHP induced different degrees of testicular injury (24,25). However, whether prenatal exposure to varying doses of DEHP leads to different interference of steroidogenesis gene expression remains uncertain. Lastly, whether prenatal DEHP exposure induces lifelong testicular toxicity through continuously interfering with steroidogenesis gene expression has not been determined. Therefore, the first aim of this study was to explore the lifelong testicular toxicity of prenatal DEHP exposure; the second was to investigate the critical role of interfering with steroidogenesis gene expression on testicular toxicity in vivo (DEHP) and in vitro (MEHP). We present this article in accordance with the ARRIVE reporting checklist (available at https://tau.amegroups.com/article/view/10.21037/tau-23-503/rc).
Methods
Animal treatment, measurement, and sample harvesting
Animal treatment
C57BL/6JGpt female and male mice (6–8 weeks old, wild type) were purchased from Guangdong Yaokang Biotechnology Corporation (Foshan, China). Mice were housed in the specific pathogen-free (SPF) animal center at Sun Yat-sen University under 12-hour light/dark cycles and allowed to acclimate to the facility for at least 2 weeks before treatment. Female and male mice were mated together (1:2) in separate cages. The presence of vaginal sperm plug and ponderal growth confirmed successful mating. Then the pregnant mice were housed separately and treated daily by gavage with the vehicle (corn oil) alone or with the vehicle containing 100, 500, and 1,000 mg/kg/d of DEHP (Sigma-Aldrich, 36735-1G, St. Louis, MO, USA) from gestational day 10 to delivery day [which covers a critical period for male genital development and differentiation in mice (26)]. Doses were adjusted daily according to body weight. The male offspring were divided into DEHP 0 (n=40, from ten litters), 100 (n=30, from eight litters), 500 (n=30, from eight litters), and 1,000 mg/kg (n=40, from ten litters) groups, accordingly. Male offspring were sacrificed (anesthetized by pentobarbital sodium and then received cervical dislocation) and observed in the neonatal [postnatal day 1 (PND1)], post-puberal (PND56), and middle-aged [postnatal month 6 (PNM6)] stages (Figure 1). The female offspring were abandoned at PND21.
Measurement and sample harvesting
Body weight, anogenital distance (AGD; measured by electronic vernier calipers), weights of genital organs (testis, epididymis, seminal vesicle), the weight of epididymal fat, and penile length were measured.
At PND1, 20 male offspring were measured and sacrificed in each group; the body and testis weight, AGD, and penile length were recorded; the bilateral testes were harvested; testes of five mice were prepared for intratesticular testosterone detection; the remaining testes of 15 mice were for qPCR and WB analysis, and every three testes from three individual mice were put together as one sample; five mixed samples from right testes were for qPCR analysis, and five composite samples from right testes were for WB analysis. The remaining mice were fed till PND56.
At PND56, ten male offspring in each group were sacrificed; the body weight, AGD, weights of genital organs (testis, epididymis, seminal vesicle), and penile length were recorded; the blood samples were collected from inferior vena cava and prepared for testosterone detection; the right testis was prepared for qPCR (n=10), and the left was for WB (n=5) and HE staining (n=5). The remaining mice were fed till PNM6.
At PNM6, ten male offspring in DEHP 0 and 1,000 mg/kg groups were sacrificed. The methods of measurement and sample harvesting were identical to that at PND56.
Experiments were performed under a project license (No. SYSU-IACUC-2022-000208) granted by the Institutional Ethics Board of Sun Yat-sen University, in compliance with the regulations of Sun Yat-sen University for the care and use of animals. A protocol was prepared before the study without registration.
TM3 cells culture, treatment, and sample harvesting
TM3 cell line was purchased from the Cell Bank of Life Technology Corporation (Priscilla, Wuhan, China). TM3 cells were cultured in Dulbecco’s modified Eagle’s medium (DMEM)/F-12 medium (Gibco, 10565018, Grand Island, CA, USA), supplemented with 10% horse serum (Gibco, S9050), 5% fetal bovine serum (Gibco, C04001-500), and 1% penicillin-streptomycin (Sangon Biotech, Shanghai, China). The cell line was maintained in a humidified incubator at 37 ℃ with 5% CO2. MEHP (MCE, HY-W018392, Monmouth Junction, NJ, USA), a major bioactive metabolite of DEHP in vivo (27,28), was used for TM3 cells treatment in vitro.
TM3 cells were inoculated in six-well plates at the concentration of 1×106 cells/well for 6 hours till adherence. The serum-containing medium was changed to add 200 µmol/L MEHP (dissolved in 0.1% DMSO); the control group was added 0.1% dimethyl sulfoxide (DMSO) simultaneously. Fifty mIU/mL HCG (Ruige, Ningbo, China) was added to simulate the intrauterine environment and promote testosterone synthesis. After incubation for 24 hours in both test reagents, cell morphology was examined under an inverted phase contrast microscope (Olympus, Tokyo, Japan). After incubation for 72 hours with MEHP or vehicle, the cell sample was collected for qPCR and WB analysis, and the medium was for testosterone detection.
RNA isolation and RT-qPCR analysis
NucleoZOL (MN, 740404.200, Düren, Germany) was used to extract the total RNA of testis and TM3 cells following the manufacturer’s instructions. The concentration of RNA was measured and adjusted using the Nanodrop ONE System (Thermo Scientific, Waltham, MA, USA). Complementary DNA was then synthesized using a PrimeScriptTM RT Master Mix (Takara, RR036A, Beijing, China). Subsequent RT-qPCR analysis of steroidogenic genes (Lhcgr, Star, Cyp11a1, Cyp17a1, Hsd17b3, and Hsd3b2) was performed using a Hieff® qPCR SYBR® Green Master Mix (High Rox) (Yeasen, 11203ES08, Shanghai, China) on the StepOnePlus Real-Time PCR System (Applied Biosystems, Waltham, MA, USA). The relative expression of target genes was calculated using the 2−ΔΔCt algorithm. We used Gapdh as the reference gene for all target genes. The primers for the genes are listed in Table 1.
Table 1
Primer name | Primer sequences | Length |
---|---|---|
Lhcgr-F | AATGAGTCCATCACGCTGAAAC | 22 |
Lhcgr-R | CCTGCAATTTGGTGGAAGAGA | 21 |
Star-F | ATGTTCCTCGCTACGTTCAAG | 21 |
Star-R | CCCAGTGCTCTCCAGTTGAG | 20 |
Cyp17a1-F | GCCCAAGTCAAAGACACCTAAT | 22 |
Cyp17a1-R | GTACCCAGGCGAAGAGAATAGA | 22 |
Hsd17b3-F | ATGGGCAGTGATTACCGGAG | 20 |
Hsd17b3-R | ACAACATTGAGTCCATGTCTGG | 22 |
Cyp11a1-F | AGGTCCTTCAATGAGATCCCTT | 22 |
Cyp11a1-R | TCCCTGTAAATGGGGCCATAC | 21 |
Hsd3b2-F | GGTTTTTGGGGCAGAGGATCA | 21 |
Hsd3b2-R | GGTACTGGGTGTCAAGAATGTCT | 23 |
Gapdh-F | AGGTCGGTGTGAACGGATTTG | 21 |
Gapdh-R | TGTAGACCATGTAGTTGAGGTCA | 23 |
Protein extraction and WB analysis
Protein extraction from the testis and TM3 cells was performed using radioimmunoprecipitation assay (RIPA) lysis buffer and a 10% protease inhibitor cocktail (MCE, HY-K0010). Lysates were centrifuged for 30 min at 12,000 g at 4 ℃, and supernatants were collected. Protein concentration was measured using the PierceTM BCA Protein Assay Kit (Thermo Scientific, 23227) on a microplate reader (562 nm, BioTech Epoch, Winooski, VT, USA). The protein sample was adjusted and prepared with a concentration of 30 µg/20 µL by water and 5× loading buffer (Beyotime, P0015, Shanghai, China). Each track loaded 30 µg/20 µL proteins. The proteins were separated by sodium dodecyl sulfate polyacrylamide gel electrophoresis (SDS-PAGE) gel electrophoresis and transferred to polyvinylidene fluoride (PVDF) membranes (0.45 µm, Merck Millipore, IPVH00010, Darmstadt, Germany). The membranes were sealed with 5% milk and then washed five times using Tris-buffered saline with Tween 20 (TBST). For WB analysis, the membranes were incubated with primary antibodies against LHR (SAB, 44352, Burlington, VT, USA), HSD3B2 (Affinity, DF6639, Montgomery, AL, USA), HSD17B3 (SAB, 31304), or β-tubulin (SAB, 48659) at 4 ℃ overnight. The primary antibody was diluted at a ratio of 1:1,000. The membranes were washed with TBST five times. They were then incubated with corresponding secondary antibodies at room temperature for 1 hour. The secondary antibody was diluted at a ratio of 1:5,000. Finally, an Immobilon Western Kit (Merck Millipore, WBKLS0500) was used to exhibit the protein band. All target proteins used β-tubulin as the reference protein.
Testosterone detection
The blood sample was centrifuged for 10 min at 5,000 g at 4 ℃ to acquire serum. The medium of TM3 cells was also centrifuged for 5 min at 5,000 g at 4 ℃, after which the supernatants were collected. The bilateral testes (about 4 mg) of neonatal mice were lysed in 100 µL RIPA lysis buffer for 30 min and then underwent centrifugation for 30 min at 12,000 g at 4 ℃, after which the supernatants were collected. The serum and supernatants were sent to the corporation (KingMed Diagnostics Group Co., Ltd., Guangzhou, China) for testosterone detection. Chemiluminescence Microparticle Immuno Assay (CMIA) was applied using ARCHITECT 2nd Generation Testosterone Reagent Kit (Abbott, 2P13.23, Shanghai, China, USA) following the manufacturer’s instructions. The coefficient of variation (CV) of CMIA is 2–5.1% for intra-assay precision and 2.6–5.2% for inter-assay precision. The minimum detectable dose of testosterone is 0.01 ng/mL.
HE staining of testis
The resected testes were fixed overnight in Bouin’s solution (Biosharp, P0111, Hefei, China), dehydrated in 75% ethanol, embedded in paraffin, and sectioned at 5 µm. The sections were deparaffinized with xylene, hydrated with graded ethanol, and stained with hematoxylin and eosin for histological analysis using an inverted phase contrast microscope (Olympus, IX83).
Semen analysis
After dissecting the mice, the caudal epididymis was shredded and incubated in the sperm incubation solution at 37 ℃ for 30 min. Then, the sperm suspension was placed in the counting chamber. Sperm count, concentration, and motility were measured using a computer-assisted sperm analysis system (Sperm Class Analyzer, Baoxing Medical Equipment Company, Xuzhou, China).
Statistical analysis
Statistical analysis was performed using the unpaired t-test or one-way analysis of variance (ANOVA) followed by the Dunnett posttest from the statistical program of the GraphPad Prism (version 4.02) package from GraphPad, Inc. (Boston, MA, USA). The mean and standard error of the mean (SEM) for the combined experiment results are presented. P<0.05 was considered statistically significant.
Results
Prenatal DEHP exposure induced immediate testicular injury in the neonatal stage by interfering with steroidogenic gene expression
The male offspring in the neonatal stage (PND1) were observed to determine the immediate testicular injury of prenatal DEHP exposure. As a result, the number of live birth, male/female ratio, litter size, appearance (data not shown), and birth weight (Figure 2A) of neonatal mice in four groups had no noticeable differences. Compared with the 0 mg/kg group, the 100, 500, and 1,000 mg/kg groups showed reduced AGD, testis weight, penile length, and intratesticular testosterone content in different degrees. The male mice recently exposed to DEHP, therefore, these results indicated that prenatal DEHP exposure induced immediate testicular injury (Figure 2B-2E).
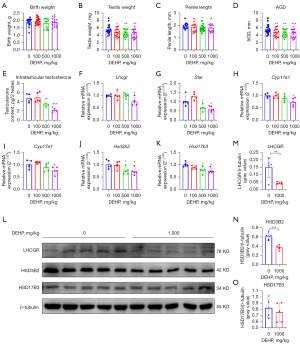
The messenger RNA (mRNA) expression of Star, Hsd3b2, and Hsd17b3 in 500 and 1,000 mg/kg groups was down-regulated; mRNA expression of Lhcgr, Cyp11a1, and Cyp17a1 in 1,000 mg/kg group was also down-regulated (Figure 2F-2K). The protein expression of LHCGR and HSD3B2 was down-regulated after DEHP exposure (Figure 2L-2O). Partial results were similar to our previous study (29). Therefore, DEHP induced immediate testicular injury in the neonatal stage by interfering with steroidogenic gene expression.
Prenatal DEHP exposure induced DSD in the post-puberal stage by interfering with steroidogenic gene expression
The male offspring in the post-puberal stage (PND56) were observed to determine the association between prenatal DEHP exposure and DSD. This study found that the unilateral testis weight in adult mice without DEHP exposure was >85 mg. If the testis was poorly developed, the whole genital organs (epididymis, seminal vesicle, and prostate) were poorly developed. Therefore, if the unilateral testis weight <85 mg, DSD was defined. Compared with the 0 mg/kg group, the mice in 500 (30%; P=0.21) and 1,000 mg/kg (50%; P=0.03) groups appeared to have higher incidences of DSD and poor development of the genital system (reduced testis, epididymis, and seminal vesicle weight; reduced AGD and penile length; Figure 3A-3G). Interestingly, the transient low dose (100 mg/kg/d) of prenatal exposure showed nearly normal genital development in the post-puberal stage. All in all, prenatal DEHP (high dose) exposure induced DSD in male offspring.
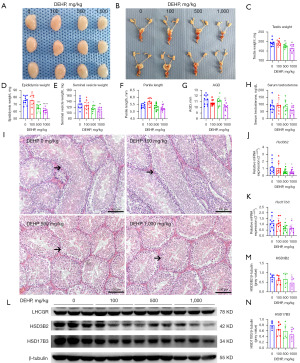
Compared with the 0 mg/kg group, only the 1,000 mg/kg group showed lower serum testosterone (Figure 3H). The semen quality and fertility in the four groups were normal (data not shown). Through HE staining of testes, we found that the testes were smaller, and the seminiferous tubules were hypogenetic, which manifested as reduced tubular diameter and length, in the 500 and 1,000 mg/kg groups (Figure 3I). According to the qPCR analysis, the mRNA expression of Hsd3b2 and Hsd17b3 was down-regulated in the 500 and 1,000 mg/kg group (Figure 3J,3K). These results were verified by WB analysis (Figure 3L-3N). Partial results were similar to our previous study (29). These results indicated that prenatal DEHP exposure induced DSD and adult male disorders by interfering with steroidogenic gene expression.
Prenatal DEHP exposure induced reproductive aging in the middle-aged stage by interfering with steroidogenic gene expression
The male offspring in the middle-aged stage (PND56) were observed to determine the association between reproductive aging and prenatal DEHP exposure. The middle-aged mice in the 1,000 mg/kg group showed obesity (increased weight of body and epididymal fat, Figure 4A-4D), reduced AGD, increased epididymis and seminal vesicle weight (Figure 4E-4G), and reduced serum testosterone (Figure 4H). Curiously, the testis weight and penile length in 0 and 1,000 mg/kg groups had no statistical differences (data not shown). In addition, the semen quality was also poorer in the 1,000 mg/kg group, which presented with low sperm density and impaired motility (Figure 4I-4K). Furthermore, the seminiferous tubules in the 1,000 mg/kg group were somewhat atrophic with thin and vacant tubes (Figure 4L). Partial results were similar to our previous study (29). These results indicated that prenatal DEHP exposure induced reproductive aging.
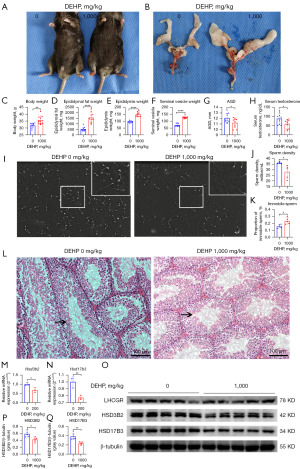
The mRNA expression of Hsd3b2 and Hsd17b3 in the 1,000 mg/kg group was down-regulated (Figure 4M,4N), as well as the protein expression of HSD3B2 and HSD17B3 (Figure 4O-4Q). In consequence, prenatal DEHP exposure induced reproductive aging in the middle-aged stage by interfering with steroidogenic gene expression.
MEHP inhibited testosterone synthesis by interfering with steroidogenic gene expression
Through microscopic examination, we found that 200 µmol/L MEHP exposure rarely induced apoptosis of TM3 cells, but 400 µmol/L MEHP exposure induced apoptosis. According to previous studies (30,31) and our findings (29), 200 µmol/L MEHP was determined as the experimental group. Our cell experiments showed that MEHP inhibited the testosterone synthesis of LCs (Figure 5A). The mRNA expression of Lhcgr, Hsd3b2, and Hsd17b3 was down-regulated; mRNA expression of Star, Cyp11a1, and Cyp17a1 was up-regulated inversely (Figure 5B-5G), which may be a response to exposure. WB analysis showed that the MEHP downregulated the expressions of LHCGR, HSD3B2, and HSD17B3 (Figure 5H-5K). Partial results were similar to our previous study (29). In conclusion, MEHP inhibited testosterone synthesis by interfering with steroidogenic gene expression.
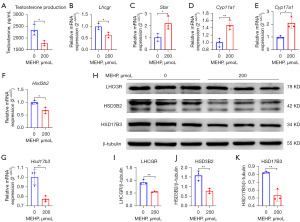
Discussion
This study systematically explored and determined the lifelong testicular toxicity of prenatal DEHP exposure. Epidemiologic studies suggested that male DSD or genital malformations (hypospadias, undescended testis, and micropenis) were associated with prenatal exposure to EEDs (5,32). In the fetal stage, especially the masculinization programming window, the testis is sensitive to EEDs (33). Prenatal exposure to EEDs interferes the testicular differentiation and development, which causes genital malformations. Infertility and low testosterone syndrome are two major adult male disorders, but the underlying pathogenesis remains unclear. Recent studies indicated that adult male disorders were associated with EEDs exposure (34,35), and the role of prenatal exposure was also suggested (36). However, further animal study is indispensable to reveal the associations between prenatal exposure and DSD, adult male disorders, and reproductive aging. This study used a mice model with prenatal DEHP exposure to confirm this theory. DEHP, a widely applied plasticizer, is easily contacted by pregnant women and causes prenatal exposure to male offspring. Epidemiologic and animal studies indicated that prenatal DEHP exposure was associated with male genital malformation (4,37,38). We confirmed that prenatal DEHP exposure induced immediate testicular injury at PND1 and DSD at PND56, revealing the association between prenatal DEHP exposure and DSD. Interestingly, although a low dose of (100 mg/kg) prenatal DEHP exposure induced immediate testicular injury in the neonatal stage, no DSD appeared when the mice grew up. However, a high dose (>500 mg/kg) of prenatal DEHP exposure induced DSDs and male reproductive disorders in the post-puberal stage, manifesting as poor genital development, reduced testosterone synthesis, and hypogenetic seminiferous tubules. These results also indicated the association between prenatal DEHP exposure and adult male disorders (infertility, low testosterone) in humans. Furthermore, we found prenatal DEHP exposure induced reproductive aging in middle-aged mice, which manifested as reduced AGD, serum testosterone, and semen quality. Thus, by observing the testicular toxicity in the neonatal, post-puberal, and middle-aged stages, we confirmed that prenatal DEHP exposure induced lifelong testicular toxicity in mice, including immediate testicular toxicity, DSD, and reproductive aging. We also found the dose dependence of testicular toxicity following prenatal DEHP exposure. Considering the unperfect therapeutic effect of congenital genital malformations and adult infertility (2,14) and ubiquitous EEDs, we call for avoiding prenatal exposure as much as possible.
In addition, we explored the role of interfering with steroidogenic gene expression in testicular toxicity following prenatal DEHP exposure through qPCR and WB analysis in vivo and in vitro. Fetal testosterone promotes the development of the male genital system. The deficiency of testosterone is the leading cause of male genital malformations (39,40). Steroidogenic genes, such as Lhcgr, Star, Cyp11a1, Cyp17a1, Hsd17b3, and Hsd3b2, play essential roles in testosterone synthesis (22). Interfering with steroidogenic gene expression will be bound to impact testosterone synthesis. Therefore, determining the impact of prenatal DEHP exposure on steroidogenic gene expression benefits revealing the toxic mechanism. Unlike previous studies, we collected the treated testis at PND1 and technically placed every three testes as a sample. Due to the small size of the neonatal testis, our ingenious method would improve the accuracy of results. As a result, our mice study at PND1 found that prenatal DEHP exposure reduced fetal testosterone synthesis by interfering with steroidogenic gene expression (Lhcgr, Star, Cyp11a1, Cyp17a1, Hsd17b3, and Hsd3b2). Moreover, our TM3 cells experiment confirmed that MEHP reduced the testosterone synthesis of LCs by interfering with steroidogenic gene expression. As the mice with prenatal DEHP exposure grew up, the ability of testosterone synthesis did not restore completely, and their steroidogenic gene expression was also impacted. The continuous interference of steroidogenic gene expression explained the lifelong testicular toxicity of prenatal DEHP exposure, including immediate testicular injury, DSD, and reproductive aging (poor semen quality, low testosterone, and even obesity) (41). Furthermore, our study found that the interference of steroidogenic gene expression following prenatal DEHP exposure was dose-dependent, and different steroidogenic genes presented different expression states (down- or up-regulated). The mRNA and protein expression of Hsd3b2 and Hsd17b3 was down-regulated and may be the critical targets of DEHP exposure. Not a few steroidogenic genes (Star, Cyp11a1, and Cyp17a1) were up-regulated as a response to exposure. Previous studies came to similar conclusions (42,43). All in all, we determined that prenatal DEHP exposure induced lifelong testicular toxicity by continuously interfering with steroidogenic gene expression.
An interesting change was found in the epididymis and seminal vesicle after prenatal DEHP exposure. In the post-puberal stage (8 weeks), the epididymis and seminal vesicle were relatively poorly developed after prenatal DEHP exposure, which was identical to previous studies (44,45). Due to the testosterone promotes the development of epididymis and seminal vesicle (46), the results in the post-puberal stage indicated the continuous deficiency of testosterone after prenatal DEHP exposure. However, the epididymis and seminal vesicle were enlarged in the middle-aged stage, which meant that prenatal DEHP exposure induced reproductive aging. Fan et al. found that a low dose of prenatal DEHP exposure caused metabolic adaptation and obesity (47), which was similar to our results. Our study also showed that prenatal DEHP exposure induces obesity and increases epididymal fat, indirectly indicating reproductive aging and testosterone deficiency. On the one hand, the lifelong impact of the epididymis and seminal vesicle further verified the testicular toxicity of prenatal DEHP exposure. On the other hand, the results observed in the middle-aged stage indicated that prenatal DEHP exposure induced reproductive aging, which deserves further study.
The present study had some limitations. Notably, the mechanism was not explored deeply; it merely stayed on the interference of steroidogenic gene expression, and the underlying mechanism deserves further study. Moreover, the phenotypes of testicular toxicity after prenatal exposure had not been fully explored, such as phenotypes in the old-aged stage, LCs morphology, and remaining genes of steroidogenesis, which may restrict the comprehensive understanding of testicular toxicity following prenatal DEHP exposure. In addition, the mice model may be more insensitive to DEHP, this study only induced poor genital development with no hypospadias. Lastly, prenatal DEHP exposure mainly impact fetal LCs, the TM3 cells cannot simulate fetal LCs perfectly, the primary LCs extracting from fetal testis may be more representative for in vivo experiment. Even so, our study had special significance in understanding the lifelong testicular toxicity of prenatal DEHP exposure and the critical role of interfering with steroidogenic gene expression. Our study suggested the association between prenatal exposure and DSD and adult male disorders in humans, thus having some clinical significance.
Conclusions
Prenatal DEHP exposure induces lifelong testicular toxicity by continuously interfering with steroidogenic gene expression, thus indicating the association between prenatal exposure and DSD, adult male disorders, and even reproductive aging. Preventing prenatal exposure is worthy of attention.
Acknowledgments
We sincerely thank the colleagues for their technical assistance and donation of experiment reagents. Those who contributed to the study implementation and manuscript writing has been reflected in the article.
Funding: This study was supported by grants from
Footnote
Reporting Checklist: The authors have completed the ARRIVE reporting checklist. Available at https://tau.amegroups.com/article/view/10.21037/tau-23-503/rc
Data Sharing Statement: Available at https://tau.amegroups.com/article/view/10.21037/tau-23-503/dss
Peer Review File: Available at https://tau.amegroups.com/article/view/10.21037/tau-23-503/prf
Conflicts of Interest: All authors have completed the ICMJE uniform disclosure form (available at https://tau.amegroups.com/article/view/10.21037/tau-23-503/coif). The authors have no conflicts of interest to declare.
Ethical Statement: The authors are accountable for all aspects of the work in ensuring that questions related to the accuracy or integrity of any part of the work are appropriately investigated and resolved. Experiments were performed under a project license (No. SYSU-IACUC-2022-000208) granted by the Institutional Ethics Board of Sun Yat-sen University, in compliance with the regulations of Sun Yat-sen University for the care and use of animals.
Open Access Statement: This is an Open Access article distributed in accordance with the Creative Commons Attribution-NonCommercial-NoDerivs 4.0 International License (CC BY-NC-ND 4.0), which permits the non-commercial replication and distribution of the article with the strict proviso that no changes or edits are made and the original work is properly cited (including links to both the formal publication through the relevant DOI and the license). See: https://creativecommons.org/licenses/by-nc-nd/4.0/.
References
- Wisniewski AB, Batista RL, Costa EMF, et al. Management of 46,XY Differences/Disorders of Sex Development (DSD) Throughout Life. Endocr Rev 2019;40:1547-72. [Crossref] [PubMed]
- Carson SA, Kallen AN. Diagnosis and Management of Infertility: A Review. JAMA 2021;326:65-76. [Crossref] [PubMed]
- Kishi R, Araki A, Miyashita C, et al. Importance of Two Birth Cohorts (n=20,926 and n=514): 15 Years' Experience of the Hokkaido Study on Environment and Children's Health: Malformation, Development and Allergy. Nihon Eiseigaku Zasshi 2018;73:164-77. [Crossref] [PubMed]
- Sathyanarayana S, Butts S, Wang C, et al. Early Prenatal Phthalate Exposure, Sex Steroid Hormones, and Birth Outcomes. J Clin Endocrinol Metab 2017;102:1870-8. [Crossref] [PubMed]
- Schiesaro MG, Amato AML, Maneschi C, et al. The Male Reproductive System and Endocrine Disruptors. Endocr Metab Immune Disord Drug Targets 2022;22:686-703. [Crossref] [PubMed]
- Erkekoglu P, Rachidi W, Yuzugullu OG, et al. Evaluation of cytotoxicity and oxidative DNA damaging effects of di(2-ethylhexyl)-phthalate (DEHP) and mono(2-ethylhexyl)-phthalate (MEHP) on MA-10 Leydig cells and protection by selenium. Toxicol Appl Pharmacol 2010;248:52-62. [Crossref] [PubMed]
- Stenz L, Escoffier J, Rahban R, et al. Testicular Dysgenesis Syndrome and Long-Lasting Epigenetic Silencing of Mouse Sperm Genes Involved in the Reproductive System after Prenatal Exposure to DEHP. PLoS One 2017;12:e0170441. [Crossref] [PubMed]
- Freire C, Castiello F, Lopez-Espinosa MJ, et al. Association of prenatal phthalate exposure with pubertal development in Spanish boys and girls. Environ Res 2022;213:113606. [Crossref] [PubMed]
- Ge X, Weis K, Flaws J, et al. Prenatal exposure to the phthalate DEHP impacts reproduction-related gene expression in the pituitary. Reprod Toxicol 2022;108:18-27. [Crossref] [PubMed]
- Barakat R, Lin PP, Rattan S, et al. Prenatal Exposure to DEHP Induces Premature Reproductive Senescence in Male Mice. Toxicol Sci 2017;156:96-108. [Crossref] [PubMed]
- Fiandanese N, Borromeo V, Berrini A, et al. Maternal exposure to a mixture of di(2-ethylhexyl) phthalate (DEHP) and polychlorinated biphenyls (PCBs) causes reproductive dysfunction in adult male mouse offspring. Reprod Toxicol 2016;65:123-32. [Crossref] [PubMed]
- Jones HB, Garside DA, Liu R, et al. The influence of phthalate esters on Leydig cell structure and function in vitro and in vivo. Exp Mol Pathol 1993;58:179-93. [Crossref] [PubMed]
- Oishi S, Hiraga K. Effects of phthalic acid monoesters on mouse testes. Toxicol Lett 1980;6:239-42. [Crossref] [PubMed]
- Cools M, Nordenström A, Robeva R, et al. Caring for individuals with a difference of sex development (DSD): a Consensus Statement. Nat Rev Endocrinol 2018;14:415-29. [Crossref] [PubMed]
- Payne AH, Hales DB. Overview of steroidogenic enzymes in the pathway from cholesterol to active steroid hormones. Endocr Rev 2004;25:947-70. [Crossref] [PubMed]
- Vo TT, Jung EM, Dang VH, et al. Di-(2 ethylhexyl) phthalate and flutamide alter gene expression in the testis of immature male rats. Reprod Biol Endocrinol 2009;7:104. [Crossref] [PubMed]
- Qin X, Ma Q, Yuan J, et al. The effects of di-2-ethylhexyl phthalate on testicular ultrastructure and hormone-regulated gene expression in male rats. Toxicol Res (Camb) 2018;7:408-14. [Crossref] [PubMed]
- Duan C, Fang Y, Sun J, et al. Effects of fast food packaging plasticizers and their metabolites on steroid hormone synthesis in H295R cells. Sci Total Environ 2020;726:138500. [Crossref] [PubMed]
- Menon KM, Menon B. Structure, function and regulation of gonadotropin receptors - a perspective. Mol Cell Endocrinol 2012;356:88-97. [Crossref] [PubMed]
- Miller WL. Steroidogenesis: Unanswered Questions. Trends Endocrinol Metab 2017;28:771-93. [Crossref] [PubMed]
- Galano M, Li Y, Li L, et al. Role of Constitutive STAR in Leydig Cells. Int J Mol Sci 2021;22:2021. [Crossref] [PubMed]
- Jin H, Yan M, Pan C, et al. Chronic exposure to polystyrene microplastics induced male reproductive toxicity and decreased testosterone levels via the LH-mediated LHR/cAMP/PKA/StAR pathway. Part Fibre Toxicol 2022;19:13. [Crossref] [PubMed]
- Li J, Fang B, Ren F, et al. TCP structure intensified the chlorpyrifos-induced decrease in testosterone synthesis via LH-LHR-PKA-CREB-Star pathway. Sci Total Environ 2020;726:138496. [Crossref] [PubMed]
- Do RP, Stahlhut RW, Ponzi D, et al. Non-monotonic dose effects of in utero exposure to di(2-ethylhexyl) phthalate (DEHP) on testicular and serum testosterone and anogenital distance in male mouse fetuses. Reprod Toxicol 2012;34:614-21. [Crossref] [PubMed]
- Dorman DC, Chiu W, Hales BF, et al. Systematic reviews and meta-analyses of human and animal evidence of prenatal diethylhexyl phthalate exposure and changes in male anogenital distance. J Toxicol Environ Health B Crit Rev 2018;21:207-26. [Crossref] [PubMed]
- Sharpe RM. Androgens and the masculinization programming window: human-rodent differences. Biochem Soc Trans 2020;48:1725-35. [Crossref] [PubMed]
- Hopf NB, Berthet A, Vernez D, et al. Skin permeation and metabolism of di(2-ethylhexyl) phthalate (DEHP). Toxicol Lett 2014;224:47-53. [Crossref] [PubMed]
- Hildenbrand S, Gabrio T, Volland G. Inter-Day Variability of Metabolites of DEHP and DnBP in Human Urine-Comparability of the Results of Longitudinal Studies with a Cross-Sectional Study. Int J Environ Res Public Health 2019;16:1029. [Crossref] [PubMed]
- Qigen X, Haiming C, Kai X, et al. Prenatal DEHP Exposure Induces Premature Testicular Aging by Promoting Leydig Cell Senescence through the MAPK Signaling Pathways. Adv Biol (Weinh) 2023;7:e2300130. [Crossref] [PubMed]
- Traore K, More P, Adla A, et al. MEHP induces alteration of mitochondrial function and inhibition of steroid biosynthesis in MA-10 mouse tumor Leydig cells. Toxicology 2021;463:152985. [Crossref] [PubMed]
- Zhao T, Wang J, Wu Y, et al. Increased m6A modification of RNA methylation related to the inhibition of demethylase FTO contributes to MEHP-induced Leydig cell injury Environ Pollut 2021;268:115627. [Crossref] [PubMed]
- Stukenborg JB, Mitchell RT, Söder O. Endocrine disruptors and the male reproductive system. Best Pract Res Clin Endocrinol Metab 2021;35:101567. [Crossref] [PubMed]
- Rodprasert W, Toppari J, Virtanen HE. Endocrine Disrupting Chemicals and Reproductive Health in Boys and Men. Front Endocrinol (Lausanne) 2021;12:706532. [Crossref] [PubMed]
- Hauser R, Skakkebaek NE, Hass U, et al. Male reproductive disorders, diseases, and costs of exposure to endocrine-disrupting chemicals in the European Union. J Clin Endocrinol Metab 2015;100:1267-77. [Crossref] [PubMed]
- Arya S, Dwivedi AK, Alvarado L, et al. Exposure of U.S. population to endocrine disruptive chemicals (Parabens, Benzophenone-3, Bisphenol-A and Triclosan) and their associations with female infertility. Environ Pollut 2020;265:114763. [Crossref] [PubMed]
- Green MP, Harvey AJ, Finger BJ, et al. Endocrine disrupting chemicals: Impacts on human fertility and fecundity during the peri-conception period. Environ Res 2021;194:110694. [Crossref] [PubMed]
- Sathyanarayana S, Grady R, Barrett ES, et al. First trimester phthalate exposure and male newborn genital anomalies. Environ Res 2016;151:777-82. [Crossref] [PubMed]
- Saillenfait AM, Sabaté JP, Gallissot F. Effects of in utero exposure to di-n-hexyl phthalate on the reproductive development of the male rat. Reprod Toxicol 2009;28:468-76. [Crossref] [PubMed]
- Mäkelä JA, Koskenniemi JJ, Virtanen HE, et al. Testis Development. Endocr Rev 2019;40:857-905. [Crossref] [PubMed]
- Grinspon RP, Bergadá I, Rey RA. Male Hypogonadism and Disorders of Sex Development. Front Endocrinol (Lausanne) 2020;11:211. [Crossref] [PubMed]
- Abdel-Maksoud FM, Ali FAZ, Akingbemi BT. Prenatal exposures to bisphenol A and di (2-ethylhexyl) phthalate disrupted seminiferous tubular development in growing male rats. Reprod Toxicol 2019;88:85-90. [Crossref] [PubMed]
- Saillenfait AM, Sabaté JP, Robert A, et al. Dose-dependent alterations in gene expression and testosterone production in fetal rat testis after exposure to di-n-hexyl phthalate. J Appl Toxicol 2013;33:1027-35. [Crossref] [PubMed]
- Vo TT, Jung EM, Dang VH, et al. Differential effects of flutamide and di-(2-ethylhexyl) phthalate on male reproductive organs in a rat model. J Reprod Dev 2009;55:400-11. [Crossref] [PubMed]
- Toxicology and carcinogenesis studies of di(2-ethylhexyl) phthalate administered in feed to Sprague Dawley (Hsd:Sprague Dawley SD) rats. Natl Toxicol Program Tech Rep Ser 2021;NTP-TR-601.
- Andrade AJ, Grande SW, Talsness CE, et al. A dose response study following in utero and lactational exposure to di-(2-ethylhexyl) phthalate (DEHP): reproductive effects on adult male offspring rats. Toxicology 2006;228:85-97. [Crossref] [PubMed]
- Hannema SE, Hughes IA. Regulation of Wolffian duct development. Horm Res 2007;67:142-51. [PubMed]
- Fan Y, Qin Y, Chen M, et al. Prenatal low-dose DEHP exposure induces metabolic adaptation and obesity: Role of hepatic thiamine metabolism. J Hazard Mater 2020;385:121534. [Crossref] [PubMed]