Extracellular vesicles and prostate cancer management: a narrative review
Introduction
Prostate cancer (PCa) is the second leading cause of cancer-related death in males in the United States, and approximately one in eight men are at risk of PCa (1). The age at diagnosis and the clinical severity vary, and the disease is heterogeneous. Localized PCa can often be well-managed via surgical intervention or radiotherapy, but patients with metastatic PCa face a bleak future, with a 5-year survival rate of 55% (2). Androgen deprivation therapy (ADT) is the cornerstone systemic strategy for locally advanced and metastatic PCa. However, prolonged ADT is often associated with development of castration-resistant PCa (CRPC). Notably, chemotherapy enhances overall survival in patients with metastatic hormone-sensitive PCa (HSPC) and CRPC (3,4). However, a substantial subset of patients who initially respond to chemotherapy eventually become treatment-resistant. The recent regulatory approval of androgen receptor (AR) signaling inhibitors (ARSIs), such as abiraterone acetate and enzalutamide, and olaparib (a poly ADP-ribose polymerase inhibitor) has triggered a paradigm shift in PCa management (5,6). However, clinical challenges remain, including accurate screening of drug-resistant PCa. Thus, the elucidation of PCa pathology and the development of efficacious therapeutic strategies require urgent study.
Extracellular vesicles (EVs) are small lipid-bilayer membrane-bound vesicles released by all living cells. EVs were identified in 1996 (7) but were initially thought to simply remove unwanted components from cells. In 2007, a milestone study from Valadi et al. showed that both mRNAs and microRNAs (miRNAs) within EVs are transferred between cells (8). This principle of transferring nucleic acid to other cell was a novel concept, many researchers started to focus on the EV as research topics. EV contents reflect the characteristics of the cells from which the EVs originate. EVs contain bioactive molecules, including miRNAs, mRNAs, proteins, DNA fragments, and lipids, which are transferred to recipient cells. These EV contents then mediate intercellular signaling in the recipient cells and affect both normal physiological processes and the pathogenesis of various diseases (9). As EVs are encapsulated, their contents are stable in bodily fluids, and thus EVs will serve as useful biomarkers (10). In this review, we summarize recent reports on EVs in PCa and discuss the potential clinical applications. We present this article in accordance with the Narrative Review reporting checklist (available at https://tau.amegroups.com/article/view/10.21037/tau-23-533/rc).
Methods
Using PubMed, a comprehensive literature search was performed. In Table 1, the search strategy for articles included is given. Peer-reviewed original articles and reviews published between 2006 and 2023 were analyzed to identify articles of relevance. The keywords searched included: “extracellular vesicles”, “exosomes”, “prostasomes”, “cancer progression”, “prostate cancer”, and “prostatic disease”. Publications that contained information that added to the existing body of literature within the field were included.
Table 1
Items | Specification |
---|---|
Date of search | September 21, 2023 |
Databases and other sources search | PubMed |
Search terms used | “extracellular vesicles”, “exosomes”, “prostasomes”, “cancer progression”, “prostate cancer”, “prostatic disease” |
Timeframe | Between the years 2006 and 2023 |
Inclusion criteria | Only English language studies were included. While peer-reviewed published manuscripts were prioritized, abstracts and textbook chapters that fit our search criteria (found on google.com) were also included |
Selection process | Each author was independently involved in literature search. The primary author (F.U.) reviewed all included articles |
Discussion
EVs
EVs are often subclassified into exosomes, microvesicles, and apoptotic bodies based on their secretory origin (11). Exosomes (30–120 nm in diameter) are intraluminal vesicles of multivesicular bodies (MVBs) and are released when MVBs fuse with cellular membranes (12). Microvesicles (100–1,000 nm in diameter) are directly released from cells via outward pinching of the plasma membrane (13). Apoptotic bodies (500–2,000 nm in diameter) are released during the final steps of apoptosis via plasma membrane blebbing (14). Additionally, prostasomes (40–5,000 nm in diameter) feature a cholesterol-rich, multilayered lipid membrane (15). Prostasomes are derived from prostate epithelial cells and are abundant in seminal fluid (16). Notably, prostasomes are ones of the first vesicles to be reported their function between cells. Prostasomes enhance spermatozoal mobility and protect spermatozoa from infection and phagocytosis by immune cells, thus contributing to human reproductive success (17,18). Furthermore, recently, novel small extracellular non-EV particles, termed exomeres, have been reported, complicating our understanding of the compositions and functions of EVs (19,20). Each EV subtype may exert distinct functions, and thus new methods are required to distinguish among the subpopulations. The International Society for Extracellular Vesicles (ISEV) developed standardized terms to be used when analyzing exosomes and other EVs, and it recommends taking great care in the use of terms for the different EVs (21,22). It is essential that scientists involved in EV research should understand the diverse and heterogenous nature of EVs (Figure 1). Against this background, here we use “EV” as an umbrella term for all small vesicles secreted into the extracellular space, i.e., exosomes, microvesicles, apoptotic bodies, prostasomes, and exomeres, which will enable the creation of a review that is in accordance with the recommendations of the ISEV (21).
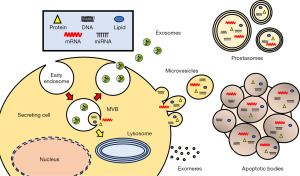
The effects of EVs on PCa progression
Between-cell communication mediated by EVs is pivotal for cancer progression. In the context of tumor initiation, Kosaka et al. firstly reported EV-mediated competition between cancer cells and adjacent normal epithelial cells (23). From then, an increasing body of evidence indicates that EVs promote cancer progression including the development of premetastatic niches (24,25), angiogenesis (26), destruction of the blood-brain barrier and the peritoneum (27,28), activation of cancer fibroblasts (29), and induction of drug resistance (30). Furthermore, Ono et al. found that EVs from bone marrow mesenchymal stem cells induce breast cancer cell dormancy and contribute to future recurrence (31).
In PCa, EVs from PCa cells enhance cellular proliferation (32), affect the immune milieu (33), and confer resistance to pharmacological interventions (34). Such actions synergistically accelerate the tumorigenic trajectory. Patients with advanced PCa often exhibit skeletal metastases that are not only impervious to treatment but also precipitate skeletal-related incidents, seriously compromising quality of life. Recently, several articles have suggested that intercellular communication facilitated by EVs contributes to bone metastasis progression (35). Such metastasis in PCa patients is predominantly osteoblastic in nature, but the lesions are the consequence of intricate interactions among disseminated cancer cells, osteoblasts, and osteoclasts (35). This intricate feedback loop is the “vicious cycle” that is postulated to underpin bone metastasis outgrowth (36).
Ito et al. were the first to describe EV-facilitated communication between PCa cells and osteoblasts. EVs from the human CRPC cell lines DU-145 and PC-3 (which express the Ets-1 protein) triggered differentiation of murine MC3T3-E1 pre-osteoblastic cells (37). Ye et al. showed that EVs from a PCa cell line, MDA-PCa-2b, not only enhanced osteoblastic activity but also regulated the microenvironments of bone metastases. miR-141-3p contained in the EVs from MDA-PCa-2b cells reduced osteoblast DLC1 levels and activated the p38MAPK pathway, in turn enhancing osteoblast proliferation and OPG/RANKL expression. In addition, they also elucidated that the EVs from the PCa cells specifically targeted bone, promoting osteoblastic metastasis in vivo (38). Hashimoto et al. reported that miR-940 encapsulated in EVs from PCa cells triggered osteogenic differentiation of human mesenchymal stem cells via precise targeting of ARHGP1 and FAM134A, thereby contributing significantly to establishment of an osteoblastic metastatic milieu (39). The cited studies focused on the effects of PCa-derived EVs on osteoblast differentiation (37-39); however, our group recently found that not only PCa-derived EVs but also crosstalk between PCa cells and osteoblasts mediated by EV transfer promoted osteoblastic bone metastases (40). Additionally, we also found that CUB domain-containing protein 1 was present on the EVs of an advanced PCa cell line, PC3M, and promoted osteoclast differentiation (41). Clinically, osteoblastic metastasis is the major form of PCa bone metastasis. However, we sometimes encounter PCa patients, especially advanced-stage patients, with partially osteolytic bone metastasis. Such metastasis is associated with a poorer prognosis compared with the osteoblastic phenotype (42). PC3M is an AR-independent, very aggressive PCa cell line that mirrors advanced-stage PCa. The mechanism by which bone metastasis is induced is complex, and further studies are required. However, reports suggest that the balance of EV-mediated intercellular communication might decide the phenotype of bone metastasis in PCa (Figure 2).
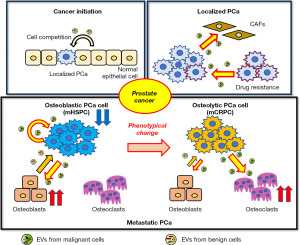
The roles played by EVs in PCa drug resistance
Antiandrogens
Antiandrogenic therapy, which comprises ADT with or without an ARSI, has traditionally been the principal systemic intervention for PCa. However, notwithstanding recent developments in antiandrogen drug development, all patients eventually become resistant to the therapy. It is thus imperative to understand the intricate molecular actions that trigger resistance to antiandrogen therapy.
Lei et al. found that let-7a was upregulated in EVs from androgen-independent PCa cells, and that it activated the AR and PI3K/Akt signaling pathways, thus contributing to androgen-independent transformation of androgen-sensitive PCa (43). Lee et al. evaluated ARSI resistance, and found that YAP1 and COUP-TFII were upregulated in enzalutamide-resistant PCa cells and EVs from these cells. Notably, after EV-mediated transfer of these components, YAP 1 and COUP-TFII upregulated many genes involved in cancer stemness and lipid metabolism in the recipient PCa cells, which then developed resistance to enzalutamide (44). Martens-Uzunova et al. recently reported that androgen manipulation drastically altered the EV RNA profiles (45), supporting the idea that EV communication contributes to the development of antiandrogen drug resistance (Figure 3A).
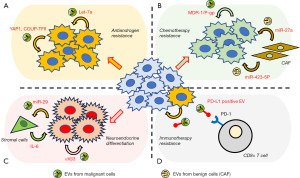
Chemotherapy
Docetaxel is the primary therapeutic modality for patients with high-volume metastatic HSPC and metastatic CRPC (4,46). However, drug resistance always develops with docetaxel treatment. The mechanism of such resistance and a method to overcome it require urgent attention.
Shan et al. found that EVs from PCa cancer-associated fibroblasts (CAFs) transfer miR-423-5p to PCa cells, thereby inducing docetaxel resistance by modulating GREM2 expression via the TGF-β signaling pathway (47). Cao et al. showed that chemotherapy significantly upregulated miR-27a expression in PCa CAF. EVs from the PCa CAF were rich in miR-27a, which increased chemoresistance by targeting the gene encoding P53 (48). Additionally, Jiang et al. reported that lincROR, an oncogenic long noncoding RNA, was packaged into the EVs of docetaxel-resistant PCa cells and then delivered to non-resistant cells, in turn stimulating the β-catenin/hypoxia-inducible factor 1-alpha pathway and contributing to dissemination of the docetaxel-resistant phenotype (49). Furthermore, EVs also enhance chemoresistance in a different approach. Corcoran et al. found that docetaxel-resistant PCa cells overexpressing MDR-1/P-gp, a transporter protein, discharged docetaxel from the cytoplasm; MDR-1/P-gp was then transferred by EVs from docetaxel-resistant PCa cells to docetaxel-sensitive cells, which contribute to acquired chemoresistance (34). Many EVs play pivotal roles in chemotherapy resistance. Intercellular communication mediated by EVs may serve as a useful therapeutic target in individuals with chemoresistant PCa (Figure 3B).
Neuroendocrine differentiation (NED)
Neuroendocrine PCa is an aggressive histological subtype of PCa that usually develops via adenocarcinoma transdifferentiation after treatment resistance is established. The term “lineage plasticity” is often used to describe the phenotypic switch from an epithelial to a neuroendocrine cell.
Quaglia et al. reported that EVs derived from PCa cells expressing Vβ3 specifically promote tumor growth and induce NED, as evidenced by increased levels of neuroendocrine markers (50). Enriquez et al. reported that crosstalk between PCa and stromal cells triggers NED development. Castration elevated GRP78 expression in PCa cells, eliciting the release of EVs that contained miR-29b, which was then transported to stromal cells, where it downregulated SPARC. This culminated in IL-6 release from stromal cells; IL-6 is a well-established stimulator of NED and acts on PCa cells (51). Extracellular communication via EVs may thus play an important role in NED development (Figure 3C).
Immunotherapy
In contrast to the marked effects of immunotherapy on profoundly immunogenic neoplasms such as melanomas, PCa is a poor responder (52,53). The four pivotal KEYNOTE trials evaluated pembrolizumab combined with other agents; no therapeutic effect was noted (54-58). PCa is a “cold” tumor; the cancer does not activate the immune system to an extent that allows tumor eradication. Frequently, the tumor microenvironment exhibits immunosuppressive characteristics, thus abundant regulatory T cells, myeloid-derived suppressor cells, and M2-polarized macrophages, all of which contribute to immune system evasion (59). Some EV studies have explored the mechanisms by which PCa enables immune system resistance.
In 2019, Poggio et al. reported that programmed death-ligand 1 (PD-L1) expressed on the surface of EVs from metastatic PCa cells escalated tumor growth in an immune system-dependent manner. In a syngeneic PCa model, resistance to anti-PD-L1 therapy appeared to be triggered by PD-L1, and therefore targeting PD-L1 might be a valuable therapeutic strategy (60). Li et al. found that PD-L1 was transferred via EVs from PCa cells expressing high levels of PD-L1 to PCa cells expressing lower levels, thus aiding the ability of PCa to evade immune cells (61) (Figure 3D).
Bone-targeting radium-223 therapy prolongs survival in a fraction of bone metastatic PCa patients (62). Vardaki et al. recently found that the levels of immune checkpoint modulators in plasma derived-EVs were increased in patients who received radium-223 treatment (63). Using a mouse model, they also revealed that radium-223 treatment increased the levels of immune checkpoint modulators both in vitro and in vivo. Consequently, they tested the significance of the findings by combining Ra-223 with immune checkpoint blockade therapy and found that the combination had greater efficacy than Ra-223 alone (63), suggesting that the combination therapy may warrant a clinical trial. Although the PCa immune microenvironment is very complex, EVs may not only contribute to treatment resistance but also serve as potential markers of treatment efficacy.
The clinical significance of EVs in PCa liquid biopsies
EV contents reflect the cellular origin of the EVs and may thus serve as valuable PCa biomarkers. Unlike conventional tissue biopsy, liquid biopsy is minimally invasive and allows real-time detection of actionable anomalies. In light of each facet of PCa management, liquid biopsy presents itself as a more compelling option. Thus, we next summarize recent clinical studies that investigated whether EVs are diagnostic or prognostic in the PCa context.
Diagnostic EVs
Given the location of the prostate, urinary EVs may elucidate aspects of prostate carcinogenesis. Many authors have explored whether urinary EVs serve as useful diagnostic indicators (64-68). McKiernan et al. pioneered an FDA-endorsed non-invasive assay: the ExoDx Prostate IntelliScore urine exosome assay. The PCA3, ERG, and SPDEF levels in urine specimens are used to calculate the ExoDx Prostate IntelliScore. The method distinguishes high-grade PCa (Gleason score ≥7) from low-grade PCa, reducing the number of unnecessary prostate biopsies (69). EVs from plasma contain PCa-specific proteins including PTEN and survivin (70,71). Aggressive PCa is characterized by little or no PTEN expression; PTEN molecules have been detected in the EVs of PCa patients but not healthy controls (70). Furthermore, elevated EV survivin levels were more common in PCa patients than in those with benign prostatic hyperplasia or healthy controls (71).
Prognostic EVs
Several studies have shown that certain EVs predict the outcomes of PCa. In recent years, many new pharmaceutical agents have been developed to address the therapeutic needs of patients with CRPC, including enzalutamide and abiraterone acetate. However, 20–40% of CRPC patients do not respond to such agents (72). The discovery that AR-V7 is key in conferring resistance to enzalutamide and abiraterone among metastatic CRPC patients has garnered substantial attention (72,73). Del Re et al. examined the role played by AR-V7-encoding RNA in plasma-derived EVs in terms of predicting resistance to hormonal therapy in patients with metastatic CRPC (74). miRNAs encapsulated in serum or plasma EVs may be used to diagnose PCa and/or predict its prognosis. Notably, the miR-141 and miR-375 levels within serum EVs have been linked to the risk of metastatic PCa (75,76). Huang et al. found that the miR-1290 and miR-375 levels in plasma EVs serve as prognostic biomarkers of metastatic PCa (77). A comprehensive RNA sequencing strategy confirmed substantial associations between the levels of these miRNAs and the overall survival of CRPC patients.
Despite that EV data has aided PCa management, only the ExoDx system is used in clinical practice, because the supposedly useful EV components differ across the various investigations, partly due to variations in the assays employed and EV storage conditions. Although cell-free DNA status is applied in guiding PCa treatment selection, in the present era of precision medicine, further advances will be anticipated. As EV-centered liquid biopsies become more informative, a large prospective validation study followed by the development of pragmatic hospital-friendly protocols is key for clinical implementation.
Future clinical applications
Cells in tumor microenvironments engage in intercellular communication principally via secretion of cytokines, chemokines, and growth factors (78). The communication within such microenvironments is mediated by EVs released from all cell types. Many studies have revealed pivotal roles played by EVs in tumor progression (79). As the tumor microenvironment matures, EV production increases exponentially, contributing to tumor progression. It is possible that therapeutic reduction in the level of tumor-derived EVs might impede cancer development. To date, three distinct approaches targeting EVs have been proposed: elimination of circulating EVs, inhibition of EV secretion, and disruption of EV uptake (10) (Figure 4A). Although we previously reported the potential of the inhibition of EV secretion in PCa (80,81), to date elimination of circulating EVs might be the optimal clinical choice. Marleau et al. (82) pioneered a therapeutic paradigm to eradicate circulating EVs, in which a sophisticated hemofiltration system selectively removes circulating EVs derived from breast cancer cells via precise targeting of the human epidermal growth factor receptor 2 (HER2) on the surfaces of such EVs (82). Notably, HER2 located on EV reduces the efficacy of cancer therapies (including trastuzumab) and contributes to cancer progression (83). Hence, selective removal of cancer-derived EVs via specific HER2 targeting will be an exceptionally promising approach for cancer treatment. Additionally, in PCa, as we referred above, PD-L1 on EVs may contribute to resistance to immunotherapy (60,61), thus, targeting PD-L1 positive EVs might be a good approach for PCa treatment.
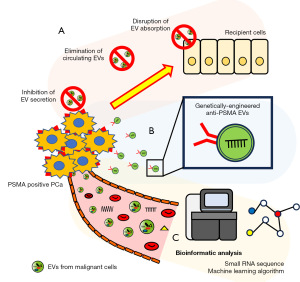
Prostate-specific membrane antigen (PSMA) is a type 2 transmembrane protein, and its expression is transcriptionally repressed by AR. Therefore, antiandrogen therapy reduces AR signaling, which increases the expression of PSMA in advanced PCa (84). According to the increasing the expression level of PSMA in PCa tissue, CD9 and PSMA double-positive plasma derived EVs are increased in patients with metastatic PCa (45). The biological function of PSMA on EVs remains unclear; one recent study proposed that EVs targeting PSMA can be used to deliver drugs. Severic et al. developed genetically engineered EVs expressing anti-PSMA antibodies that were internalized by PSMA-positive PCa cell lines both in vitro and in vivo (85). siRNA loading of the EVs expressing anti-PSMA antibody reduced the target gene expression in the recipient cells (PSMA-positive PCa cells) (86). Thus, EV-based drug carriers may greatly aid clinical drug delivery (Figure 4B).
Efforts have been made to enhance the cancer detection and prediction of liquid biopsy. Machine learning has been employed to analyze EV proteomic profiles. Hoshino et al. meticulously amassed 426 human tissue explants, and plasma and other bodily fluid samples, from patients with seven distinct cancers and from healthy individuals (87). Exhaustive proteomic profiles obtained via low- and high-resolution/high-mass-accuracy nano-liquid chromatography-tandem mass spectrometry data were subjected to rigorous evaluation by machine learning algorithms (87). A set of tumor-type-specific EV proteins in both tissue explants and plasma were identified and were able to categorize malignancies of enigmatic primary provenance (87). EV proteins may thus serve as useful biomarkers of cancer and specific cancer subtypes. Of course, further work will be required for clinical application (Figure 4C).
Conclusions
We have summarized recent research on how EVs may aid PCa management. To date, we have discovered only the tip of the iceberg. We anticipate that further research will yield innovative therapeutic modalities, thereby aiding all PCa patients.
Acknowledgments
The authors thank the members of Laboratory of Integrative Oncology, National Cancer Center Research Institute for critical discussion regarding this manuscript. The authors also thank Textcheck Inc. for English language editing.
Funding: None.
Footnote
Reporting Checklist: The authors have completed the Narrative Review reporting checklist. Available at https://tau.amegroups.com/article/view/10.21037/tau-23-533/rc
Peer Review File: Available at https://tau.amegroups.com/article/view/10.21037/tau-23-533/prf
Conflicts of Interest: All authors have completed the ICMJE uniform disclosure form (available at https://tau.amegroups.com/article/view/10.21037/tau-23-533/coif). The authors have no conflicts of interest to declare.
Ethical Statement: The authors are accountable for all aspects of the work in ensuring that questions related to the accuracy or integrity of any part of the work are appropriately investigated and resolved.
Open Access Statement: This is an Open Access article distributed in accordance with the Creative Commons Attribution-NonCommercial-NoDerivs 4.0 International License (CC BY-NC-ND 4.0), which permits the non-commercial replication and distribution of the article with the strict proviso that no changes or edits are made and the original work is properly cited (including links to both the formal publication through the relevant DOI and the license). See: https://creativecommons.org/licenses/by-nc-nd/4.0/.
References
- Siegel RL, Miller KD, Wagle NS, et al. Cancer statistics, 2023. CA Cancer J Clin 2023;73:17-48. [Crossref] [PubMed]
- Helgstrand JT, Røder MA, Klemann N, et al. Trends in incidence and 5-year mortality in men with newly diagnosed, metastatic prostate cancer-A population-based analysis of 2 national cohorts. Cancer 2018;124:2931-8. [Crossref] [PubMed]
- Sweeney CJ, Chen YH, Carducci M, et al. Chemohormonal Therapy in Metastatic Hormone-Sensitive Prostate Cancer. N Engl J Med 2015;373:737-46. [Crossref] [PubMed]
- Tannock IF, de Wit R, Berry WR, et al. Docetaxel plus prednisone or mitoxantrone plus prednisone for advanced prostate cancer. N Engl J Med 2004;351:1502-12. [Crossref] [PubMed]
- Menges D, Yebyo HG, Sivec-Muniz S, et al. Treatments for Metastatic Hormone-sensitive Prostate Cancer: Systematic Review, Network Meta-analysis, and Benefit-harm assessment. Eur Urol Oncol 2022;5:605-16. [Crossref] [PubMed]
- de Bono J, Mateo J, Fizazi K, et al. Olaparib for Metastatic Castration-Resistant Prostate Cancer. N Engl J Med 2020;382:2091-102. [Crossref] [PubMed]
- Raposo G, Nijman HW, Stoorvogel W, et al. B lymphocytes secrete antigen-presenting vesicles. J Exp Med 1996;183:1161-72. [Crossref] [PubMed]
- Valadi H, Ekström K, Bossios A, et al. Exosome-mediated transfer of mRNAs and microRNAs is a novel mechanism of genetic exchange between cells. Nat Cell Biol 2007;9:654-9. [Crossref] [PubMed]
- Urabe F, Patil K, Ramm GA, et al. Extracellular vesicles in the development of organ-specific metastasis. J Extracell Vesicles 2021;10:e12125. [Crossref] [PubMed]
- Urabe F, Kosaka N, Ito K, et al. Extracellular vesicles as biomarkers and therapeutic targets for cancer. Am J Physiol Cell Physiol 2020;318:C29-39. [Crossref] [PubMed]
- Raposo G, Stoorvogel W. Extracellular vesicles: exosomes, microvesicles, and friends. J Cell Biol 2013;200:373-83. [Crossref] [PubMed]
- Cocucci E, Meldolesi J. Ectosomes and exosomes: shedding the confusion between extracellular vesicles. Trends Cell Biol 2015;25:364-72. [Crossref] [PubMed]
- Yáñez-Mó M, Siljander PR, Andreu Z, et al. Biological properties of extracellular vesicles and their physiological functions. J Extracell Vesicles 2015;4:27066. [Crossref] [PubMed]
- Muralidharan-Chari V, Clancy JW, Sedgwick A, et al. Microvesicles: mediators of extracellular communication during cancer progression. J Cell Sci 2010;123:1603-11. [Crossref] [PubMed]
- Stridsberg M, Fabiani R, Lukinius A, et al. Prostasomes are neuroendocrine-like vesicles in human semen. Prostate 1996;29:287-95. [Crossref] [PubMed]
- Utleg AG, Yi EC, Xie T, et al. Proteomic analysis of human prostasomes. Prostate 2003;56:150-61. [Crossref] [PubMed]
- Carlsson L, Påhlson C, Bergquist M, et al. Antibacterial activity of human prostasomes. Prostate 2000;44:279-86. [Crossref] [PubMed]
- Poliakov A, Spilman M, Dokland T, et al. Structural heterogeneity and protein composition of exosome-like vesicles (prostasomes) in human semen. Prostate 2009;69:159-67. [Crossref] [PubMed]
- Zhang H, Freitas D, Kim HS, et al. Identification of distinct nanoparticles and subsets of extracellular vesicles by asymmetric flow field-flow fractionation. Nat Cell Biol 2018;20:332-43. [Crossref] [PubMed]
- Zhang H, Lyden D. Asymmetric-flow field-flow fractionation technology for exomere and small extracellular vesicle separation and characterization. Nat Protoc 2019;14:1027-53. [Crossref] [PubMed]
- Théry C, Witwer KW, Aikawa E, et al. Minimal information for studies of extracellular vesicles 2018 (MISEV2018): a position statement of the International Society for Extracellular Vesicles and update of the MISEV2014 guidelines. J Extracell Vesicles 2018;7:1535750. [Crossref] [PubMed]
- Witwer KW, Soekmadji C, Hill AF, et al. Updating the MISEV minimal requirements for extracellular vesicle studies: building bridges to reproducibility. J Extracell Vesicles 2017;6:1396823. [Crossref] [PubMed]
- Kosaka N, Iguchi H, Yoshioka Y, et al. Competitive interactions of cancer cells and normal cells via secretory microRNAs. J Biol Chem 2012;287:1397-405. [Crossref] [PubMed]
- Peinado H, Alečković M, Lavotshkin S, et al. Melanoma exosomes educate bone marrow progenitor cells toward a pro-metastatic phenotype through MET. Nat Med 2012;18:883-91. [Crossref] [PubMed]
- Grange C, Tapparo M, Collino F, et al. Microvesicles released from human renal cancer stem cells stimulate angiogenesis and formation of lung premetastatic niche. Cancer Res 2011;71:5346-56. [Crossref] [PubMed]
- Kosaka N, Iguchi H, Hagiwara K, et al. Neutral sphingomyelinase 2 (nSMase2)-dependent exosomal transfer of angiogenic microRNAs regulate cancer cell metastasis. J Biol Chem 2013;288:10849-59. [Crossref] [PubMed]
- Tominaga N, Kosaka N, Ono M, et al. Brain metastatic cancer cells release microRNA-181c-containing extracellular vesicles capable of destructing blood-brain barrier. Nat Commun 2015;6:6716. [Crossref] [PubMed]
- Yokoi A, Yoshioka Y, Yamamoto Y, et al. Malignant extracellular vesicles carrying MMP1 mRNA facilitate peritoneal dissemination in ovarian cancer. Nat Commun 2017;8:14470. [Crossref] [PubMed]
- Pang W, Su J, Wang Y, et al. Pancreatic cancer-secreted miR-155 implicates in the conversion from normal fibroblasts to cancer-associated fibroblasts. Cancer Sci 2015;106:1362-9. [Crossref] [PubMed]
- Wei F, Ma C, Zhou T, et al. Exosomes derived from gemcitabine-resistant cells transfer malignant phenotypic traits via delivery of miRNA-222-3p. Mol Cancer 2017;16:132. [Crossref] [PubMed]
- Ono M, Kosaka N, Tominaga N, et al. Exosomes from bone marrow mesenchymal stem cells contain a microRNA that promotes dormancy in metastatic breast cancer cells. Sci Signal 2014;7:ra63. [Crossref] [PubMed]
- Hosseini-Beheshti E, Choi W, Weiswald LB, et al. Exosomes confer pro-survival signals to alter the phenotype of prostate cells in their surrounding environment. Oncotarget 2016;7:14639-58. [Crossref] [PubMed]
- Lundholm M, Schröder M, Nagaeva O, et al. Prostate tumor-derived exosomes down-regulate NKG2D expression on natural killer cells and CD8+ T cells: mechanism of immune evasion. PLoS One 2014;9:e108925. [Crossref] [PubMed]
- Corcoran C, Rani S, O'Brien K, et al. Docetaxel-resistance in prostate cancer: evaluating associated phenotypic changes and potential for resistance transfer via exosomes. PLoS One 2012;7:e50999. [Crossref] [PubMed]
- Park SH, Keller ET, Shiozawa Y. Bone Marrow Microenvironment as a Regulator and Therapeutic Target for Prostate Cancer Bone Metastasis. Calcif Tissue Int 2018;102:152-62. [Crossref] [PubMed]
- Cook LM, Shay G, Araujo A, et al. Integrating new discoveries into the "vicious cycle" paradigm of prostate to bone metastases. Cancer Metastasis Rev 2014;33:511-25. [Crossref] [PubMed]
- Itoh T, Ito Y, Ohtsuki Y, et al. Microvesicles released from hormone-refractory prostate cancer cells facilitate mouse pre-osteoblast differentiation. J Mol Histol 2012;43:509-15. [Crossref] [PubMed]
- Ye Y, Li SL, Ma YY, et al. Exosomal miR-141-3p regulates osteoblast activity to promote the osteoblastic metastasis of prostate cancer. Oncotarget 2017;8:94834-49. [Crossref] [PubMed]
- Hashimoto K, Ochi H, Sunamura S, et al. Cancer-secreted hsa-miR-940 induces an osteoblastic phenotype in the bone metastatic microenvironment via targeting ARHGAP1 and FAM134A. Proc Natl Acad Sci U S A 2018;115:2204-9. [Crossref] [PubMed]
- Ito K, Yamamoto T, Hayashi Y, et al. Osteoblast-derived extracellular vesicles exert osteoblastic and tumor-suppressive functions via SERPINA3 and LCN2 in prostate cancer. Mol Oncol 2023;17:2147-67. [Crossref] [PubMed]
- Urabe F, Kosaka N, Yamamoto Y, et al. Metastatic prostate cancer-derived extracellular vesicles facilitate osteoclastogenesis by transferring the CDCP1 protein. J Extracell Vesicles 2023;12:e12312. [Crossref] [PubMed]
- Shimazaki J, Higa T, Akimoto S, et al. Clinical course of bone metastasis from prostatic cancer following endocrine therapy: examination with bone x-ray. Adv Exp Med Biol 1992;324:269-75. [Crossref] [PubMed]
- Lei L, Yu L, Fan W, et al. Prostate cancer small extracellular vesicles participate in androgen-independent transformation of prostate cancer by transferring let-7a-5p. Heliyon 2022;8:e12114. [Crossref] [PubMed]
- Lee HC, Ou CH, Huang YC, et al. YAP1 overexpression contributes to the development of enzalutamide resistance by induction of cancer stemness and lipid metabolism in prostate cancer. Oncogene 2021;40:2407-21. [Crossref] [PubMed]
- Martens-Uzunova ES, Kusuma GD, Crucitta S, et al. Androgens alter the heterogeneity of small extracellular vesicles and the small RNA cargo in prostate cancer. J Extracell Vesicles 2021;10:e12136. [Crossref] [PubMed]
- Kyriakopoulos CE, Chen YH, Carducci MA, et al. Chemohormonal Therapy in Metastatic Hormone-Sensitive Prostate Cancer: Long-Term Survival Analysis of the Randomized Phase III E3805 CHAARTED Trial. J Clin Oncol 2018;36:1080-7. [Crossref] [PubMed]
- Shan G, Gu J, Zhou D, et al. Cancer-associated fibroblast-secreted exosomal miR-423-5p promotes chemotherapy resistance in prostate cancer by targeting GREM2 through the TGF-β signaling pathway. Exp Mol Med 2020;52:1809-22. [Crossref] [PubMed]
- Cao Z, Xu L, Zhao S. Exosome-derived miR-27a produced by PSC-27 cells contributes to prostate cancer chemoresistance through p53. Biochem Biophys Res Commun 2019;515:345-51. [Crossref] [PubMed]
- Jiang X, Xu Y, Liu R, et al. Exosomal lincROR Promotes Docetaxel Resistance in Prostate Cancer through a β-catenin/HIF1α Positive Feedback Loop. Mol Cancer Res 2023;21:472-82. [Crossref] [PubMed]
- Quaglia F, Krishn SR, Daaboul GG, et al. Small extracellular vesicles modulated by αVβ3 integrin induce neuroendocrine differentiation in recipient cancer cells. J Extracell Vesicles 2020;9:1761072. [Crossref] [PubMed]
- Enriquez C, Cancila V, Ferri R, et al. Castration-Induced Downregulation of SPARC in Stromal Cells Drives Neuroendocrine Differentiation of Prostate Cancer. Cancer Res 2021;81:4257-74. [Crossref] [PubMed]
- Topalian SL, Hodi FS, Brahmer JR, et al. Five-Year Survival and Correlates Among Patients With Advanced Melanoma, Renal Cell Carcinoma, or Non-Small Cell Lung Cancer Treated With Nivolumab. JAMA Oncol 2019;5:1411-20. [Crossref] [PubMed]
- Topalian SL, Hodi FS, Brahmer JR, et al. Safety, activity, and immune correlates of anti-PD-1 antibody in cancer. N Engl J Med 2012;366:2443-54. [Crossref] [PubMed]
- Yu EY, Kolinsky MP, Berry WR, et al. Pembrolizumab Plus Docetaxel and Prednisone in Patients with Metastatic Castration-resistant Prostate Cancer: Long-term Results from the Phase 1b/2 KEYNOTE-365 Cohort B Study. Eur Urol 2022;82:22-30. [Crossref] [PubMed]
- Yu EY, Piulats JM, Gravis G, et al. Pembrolizumab plus Olaparib in Patients with Metastatic Castration-resistant Prostate Cancer: Long-term Results from the Phase 1b/2 KEYNOTE-365 Cohort A Study. Eur Urol 2023;83:15-26. [Crossref] [PubMed]
- Antonarakis ES, Park SH, Goh JC, et al. Pembrolizumab Plus Olaparib for Patients With Previously Treated and Biomarker-Unselected Metastatic Castration-Resistant Prostate Cancer: The Randomized, Open-Label, Phase III KEYLYNK-010 Trial. J Clin Oncol 2023;41:3839-50. [Crossref] [PubMed]
- Gratzke C, Kwiatkowski M, De Giorgi U, et al. KEYNOTE-991: pembrolizumab plus enzalutamide and androgen deprivation for metastatic hormone-sensitive prostate cancer. Future Oncol 2023; Epub ahead of print. [Crossref] [PubMed]
- Petrylak DP, Ratta R, Gafanov R, et al. KEYNOTE-921: Phase III study of pembrolizumab plus docetaxel for metastatic castration-resistant prostate cancer. Future Oncol 2021;17:3291-9. [Crossref] [PubMed]
- Kwon JTW, Bryant RJ, Parkes EE. The tumor microenvironment and immune responses in prostate cancer patients. Endocr Relat Cancer 2021;28:T95-T107. [Crossref] [PubMed]
- Poggio M, Hu T, Pai CC, et al. Suppression of Exosomal PD-L1 Induces Systemic Anti-tumor Immunity and Memory. Cell 2019;177:414-427.e13. [Crossref] [PubMed]
- Li D, Zhou X, Xu W, et al. Prostate cancer cells synergistically defend against CD8(+) T cells by secreting exosomal PD-L1. Cancer Med 2023;12:16405-15. [Crossref] [PubMed]
- Hoskin P, Sartor O, O'Sullivan JM, et al. Efficacy and safety of radium-223 dichloride in patients with castration-resistant prostate cancer and symptomatic bone metastases, with or without previous docetaxel use: a prespecified subgroup analysis from the randomised, double-blind, phase 3 ALSYMPCA trial. Lancet Oncol 2014;15:1397-406. [Crossref] [PubMed]
- Vardaki I, Corn P, Gentile E, et al. Radium-223 Treatment Increases Immune Checkpoint Expression in Extracellular Vesicles from the Metastatic Prostate Cancer Bone Microenvironment. Clin Cancer Res 2021;27:3253-64. [Crossref] [PubMed]
- Nilsson J, Skog J, Nordstrand A, et al. Prostate cancer-derived urine exosomes: a novel approach to biomarkers for prostate cancer. Br J Cancer 2009;100:1603-7. [Crossref] [PubMed]
- Işın M, Uysaler E, Özgür E, et al. Exosomal lncRNA-p21 levels may help to distinguish prostate cancer from benign disease. Front Genet 2015;6:168. [PubMed]
- Donovan MJ, Noerholm M, Bentink S, et al. A molecular signature of PCA3 and ERG exosomal RNA from non-DRE urine is predictive of initial prostate biopsy result. Prostate Cancer Prostatic Dis 2015;18:370-5. [Crossref] [PubMed]
- Foj L, Ferrer F, Serra M, et al. Exosomal and Non-Exosomal Urinary miRNAs in Prostate Cancer Detection and Prognosis. Prostate 2017;77:573-83. [Crossref] [PubMed]
- Rodríguez M, Bajo-Santos C, Hessvik NP, et al. Identification of non-invasive miRNAs biomarkers for prostate cancer by deep sequencing analysis of urinary exosomes. Mol Cancer 2017;16:156. [Crossref] [PubMed]
- McKiernan J, Donovan MJ, O'Neill V, et al. A Novel Urine Exosome Gene Expression Assay to Predict High-grade Prostate Cancer at Initial Biopsy. JAMA Oncol 2016;2:882-9. [Crossref] [PubMed]
- Gabriel K, Ingram A, Austin R, et al. Regulation of the tumor suppressor PTEN through exosomes: a diagnostic potential for prostate cancer. PLoS One 2013;8:e70047. [Crossref] [PubMed]
- Khan S, Jutzy JM, Valenzuela MM, et al. Plasma-derived exosomal survivin, a plausible biomarker for early detection of prostate cancer. PLoS One 2012;7:e46737. [Crossref] [PubMed]
- Antonarakis ES, Lu C, Wang H, et al. AR-V7 and resistance to enzalutamide and abiraterone in prostate cancer. N Engl J Med 2014;371:1028-38. [Crossref] [PubMed]
- Seitz AK, Thoene S, Bietenbeck A, et al. AR-V7 in Peripheral Whole Blood of Patients with Castration-resistant Prostate Cancer: Association with Treatment-specific Outcome Under Abiraterone and Enzalutamide. Eur Urol 2017;72:828-34. [Crossref] [PubMed]
- Del Re M, Biasco E, Crucitta S, et al. The Detection of Androgen Receptor Splice Variant 7 in Plasma-derived Exosomal RNA Strongly Predicts Resistance to Hormonal Therapy in Metastatic Prostate Cancer Patients. Eur Urol 2017;71:680-7. [Crossref] [PubMed]
- Bryant RJ, Pawlowski T, Catto JW, et al. Changes in circulating microRNA levels associated with prostate cancer. Br J Cancer 2012;106:768-74. [Crossref] [PubMed]
- Li Z, Ma YY, Wang J, et al. Exosomal microRNA-141 is upregulated in the serum of prostate cancer patients. Onco Targets Ther 2015;9:139-48. [PubMed]
- Huang X, Yuan T, Liang M, et al. Exosomal miR-1290 and miR-375 as prognostic markers in castration-resistant prostate cancer. Eur Urol 2015;67:33-41. [Crossref] [PubMed]
- Xu R, Rai A, Chen M, et al. Extracellular vesicles in cancer - implications for future improvements in cancer care. Nat Rev Clin Oncol 2018;15:617-38. [Crossref] [PubMed]
- Naito Y, Yoshioka Y, Yamamoto Y, et al. How cancer cells dictate their microenvironment: present roles of extracellular vesicles. Cell Mol Life Sci 2017;74:697-713. [Crossref] [PubMed]
- Urabe F, Kosaka N, Sawa Y, et al. The miR-1908/SRM regulatory axis contributes to extracellular vesicle secretion in prostate cancer. Cancer Sci 2020;111:3258-67. [Crossref] [PubMed]
- Urabe F, Kosaka N, Sawa Y, et al. miR-26a regulates extracellular vesicle secretion from prostate cancer cells via targeting SHC4, PFDN4, and CHORDC1. Sci Adv 2020;6:eaay3051. [Crossref] [PubMed]
- Marleau AM, Chen CS, Joyce JA, et al. Exosome removal as a therapeutic adjuvant in cancer. J Transl Med 2012;10:134. [Crossref] [PubMed]
- Ciravolo V, Huber V, Ghedini GC, et al. Potential role of HER2-overexpressing exosomes in countering trastuzumab-based therapy. J Cell Physiol 2012;227:658-67. [Crossref] [PubMed]
- Bakht MK, Oh SW, Youn H, et al. Influence of Androgen Deprivation Therapy on the Uptake of PSMA-Targeted Agents: Emerging Opportunities and Challenges. Nucl Med Mol Imaging 2017;51:202-11. [Crossref] [PubMed]
- Severic M, Ma G, Pereira SGT, et al. Genetically-engineered anti-PSMA exosome mimetics targeting advanced prostate cancer in vitro and in vivo. J Control Release 2021;330:101-10. [Crossref] [PubMed]
- Greco KA, Franzen CA, Foreman KE, et al. PLK-1 Silencing in Bladder Cancer by siRNA Delivered With Exosomes. Urology 2016;91:241.e1-7. [Crossref] [PubMed]
- Hoshino A, Kim HS, Bojmar L, et al. Extracellular Vesicle and Particle Biomarkers Define Multiple Human Cancers. Cell 2020;182:1044-1061.e18. [Crossref] [PubMed]