About metformin and its action on the mitochondrial respiratory chain in prostate cancer
The dramatically increasing costs related to the research and development of new anticancer drugs has attracted special attention on drug repurposing. Metformin, the most widely prescribed drug worldwide for patients suffering from type 2 diabetes, is one of the potential candidates. At the time of writing this editorial, according to the website clinicaltrial.gov, metformin has been included in 427 clinical trials in cancer patients, including 37 studies for prostate cancer. However, so far, combining metformin with conventional anticancer treatments has been inconclusive: while several reports [reviewed in (1)] suggested a potential benefit, including for treating prostate cancer (2), clinical trials failed to prove any benefit on the outcome of prostate cancer patients (3,4). The discrepancy in conclusions from these trials suggests that the anticancer activity of metformin in prostate cancer therapy (if any) could be dependent on the presence or absence of specific factors in individual tumors. In this context, a recent paper of Papachristodoulou et al. suggests that prostate cancer patients with low expression of the prostate-specific homeobox gene NKX3.1, but not those with high NKX3.1 expression, are likely to benefit from metformin to impede cancer progression (5). In this editorial, we provide a timely perspective on the potential utility of metformin to treat prostate cancer, given its multiple molecular mechanisms of action, with a special focus on the mitochondrial electron transport chain (ETC).
In diabetic patients, metformin benefits are related to glucose metabolism and diabetes-related complications. Mechanistically, metformin inhibits the ETC in the liver, consequently activating AMP-activated protein kinase (AMPK), enhancing insulin sensitivity and lowering cAMP, which collectively decrease the expression of gluconeogenic enzymes (6). Comparatively, the anti-neoplastic effects of metformin are far from being fully elucidated. As most cancer cells exhibit high glucose consumption and a dysregulated glucose metabolism, a simplistic explanation would be that metformin could decrease energy production by proliferative cancer cells. However, metformin may act by several other mechanisms, as reviewed by Zhao et al. (7). The most important mechanisms are summarized in Figure 1. In the tumor microenvironment, metformin can indeed stimulate anticancer immunity through the NF-κB signaling pathway (8). It also exerts antiangiogenic effects (9) and can also improve the sensitivity to chemotherapeutics (10). Direct effects on cancer cells further encompass the capability of metformin to arrest the cell cycle through activating the AMPK and phosphatidylinositol 3-kinase (PI3K) pathways (7), and to alter DNA methylation and histone acetylation through modulation of one- and two-carbon metabolism. Several studies further highlighted that metformin affects cancer cell mitochondria (Figure 2), as it inhibits ETC complex I and ATP production, including in prostate cancer cells (11-13). The drop in ATP production supports AMPK activation, which consequently inhibits the mTOR pathway, biosynthesis and cancer cell proliferation (7). In prostate cancer cells, lipogenesis is strongly affected (14). Metformin may also induce cancer cell death by sequentially triggering the translocation of Bax into mitochondria, cytochrome c release, apoptosome formation, caspase activation and apoptosis (7,15). ETC complex I inhibition by metformin also modulates the mitochondrial production of reactive oxygen species (ROS) by cancer cells: while some reports described enhanced antioxidants defenses (mitohormesis) (16), others rather highlighted enhanced mitochondrial ROS production, including in prostate cancer cells (17-19). Of note, ROS plays a key role in prostate cancer onset (20) and castration resistance occurrence is favored in prostate cancer cells expressing high antioxidant capacity (21,22).
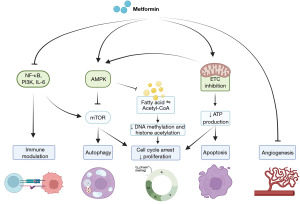
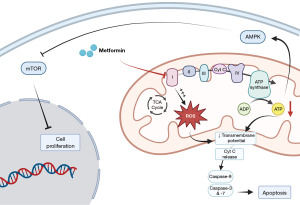
Integrating the broad spectrum of actions of metformin is important to contextualize the new report of Papachristodoulou et al. These authors suggest that determining NKX3.1 expression levels may help to identify prostate cancer patients who are likely to benefit from metformin administration (5). NKX3.1 is a homeobox gene, i.e., a DNA sequence that normally regulates anatomical features during embryogenesis, known to support the normal differentiation of the prostatic epithelium. Conversely, NKX3.1 loss of function is considered as an initiating event in prostate carcinogenesis (23). In a previous publication, Papachristodoulou et al. described a nonnuclear function of NKX3.1 that suppresses prostate cancer (24). In human prostate cancer cells exposed to oxidative stress, they found that NKX3.1 is imported into mitochondria where it stimulates the transcription of mitochondrial-encoded ETC genes, thereby restoring oxidative phosphorylation and preventing cancer initiation (24). They also demonstrated an association between NKX3.1 expression and cancer outcome: by analyzing human biopsies, they found that low levels of NKX3.1 combined with low levels of mitochondrial ETC genes were associated with a poor clinical outcome, whereas high expression of NKX3.1 was associated with a more favorable outcome (24). The new study published in European Urology (5) suggests that metformin could potentially overcome the adverse consequences of loss NKX3.1 by protecting prostate cancer cells against oxidative stress and promoting normal mitochondrial functions.
As the benefit of using metformin in prostate cancer has been so far inconclusive, seeking predictive markers to stratify patients is unquestionably important. The results from the retrospective analyses presented in this study are interesting. The authors performed tissue microarray analyses in specimens coming from radical prostatectomy in two independent cohorts of patients who were taking or not taking metformin. Patients with low or high NKX3.1 expression were distributed among those taking or not taking metformin across Gleason grades and risk groups according to the European Association of Urology (EAU) guidelines. Patients from both cohorts who displayed low levels of NKX3.1 and were taking metformin had significantly improved biochemical recurrence-free survival compared to patients who were not taking metformin. Papachristodoulou et al. also found that patients with high levels of NKX3.1 displayed no difference in overall survival regardless of whether they were taking or not taking metformin (5). In their conclusions, the authors suggested that prospective randomized controlled clinical trials should now evaluate the association of NKX3.1 expression and metformin usage in prostate cancer (5).
While we fully support the need to complement clinical data to better define the role played by NKX3.1 in prostate cancer progression, the mechanisms proposed by the authors to link NKX3.1 expression and the benefits of metformin deserve a more balanced commentary. For Papachristodoulou et al., metformin could overcome the adverse consequences of NKX3.1 loss in prostate cancer cells by protecting them against oxidative stress and restoring normal mitochondrial functions (5). Their starting hypothesis is that excessive ROS production due to oxidative stress may impair mitochondrial functions and that NKX3.1 could protect the organelles against the consequences of the oxidative damage. To demonstrate this, they experimentally induced oxidative stress with paraquat, an herbicide known for its devastating toxicity after accidental or voluntary poisoning. NKX3.1 wild-type (NKX3.1+/+) or homozygous deficient (NKX3.1–/–) mice received paraquat for 9 months and metformin during the last 3 months of paraquat administration. The authors justified the use of paraquat because it “has been observed to have negligible effects other than in prostate”, which is contradictory to what has been observed by others in numerous toxicological studies. It is well established that paraquat undergoes a NADPH-dependent one-electron reduction to produce its free radical, which then instantly reacts with oxygen to reform the cation and produce superoxide anion (25). In addition, paraquat may initiate a global inflammatory response by affecting multiple redox-sensitive signaling pathways, including activation of mitogen-activated protein kinases (MAPKs), protein kinase B (Akt)/β-catenin, toll-like receptors, and suppression of PPAR-γ receptor activity (25). Paraquat is known for its dramatic toxicity, mainly in the lungs, liver and brain. Due to the pleiotropic effects that can be induced by paraquat, the selection of this drug to induce chronic oxidative stress is questionable to analyze the effect of metformin, especially because metformin may act by many different mechanisms, as previously stated. Paraquat is known to induce a massive production of free radicals. It is therefore quite intriguing that the authors report that paraquat increased ROS production in NKX3.1–/–, but not in NKX3.1+/+ mice (5). One could wonder whether the unspecific assays used for quantifying ROS (5) could have led to artifactual estimates of ROS levels (26). Another unexpected result of the study is the apparent absence of any effect of metformin used alone on the oxygen consumption rate of prostate cancer cells (5), while metformin has been reported to interfere with the mitochondrial respiration of many cancer cells models (11-13,17-19), often triggering mitochondrial superoxide production (17-19). The authors reported that paraquat decreased mitochondrial respiration in NKX3.1-depleted cells but not in control cells, and that mitochondrial respiration was rescued by metformin (5). If confirmed, these interesting results could shed a new light on the role of NKX3.1 and refine the use of metformin for treating prostate cancer. For future studies, the use of models where oxidative stress would be generated selectively in cancer cells instead of the massive ROS overload induced by paraquat, is warranted. For example, highly invasive and metastatic models have been developed in which selected cells simultaneously gained aggressiveness and a high production of mitochondrial superoxide compared to parental cells (27-30). So far, these models have been developed for melanoma, breast, lung and pancreatic cancer, and it could be interesting to use a same strategy to study prostate cancer. Such models would not only help to confirm the results obtained by Papachristodoulou et al. in a more relevant pathophysiological context, but they could also provide key information to firmly establish that metformin can be used to inhibit metastatic progression, the main actual clinical reason of prostate cancer-associated death.
Acknowledgments
Funding: This work was supported by
Footnote
Provenance and Peer Review: This article was commissioned by the editorial office, Translational Andrology and Urology. The article has undergone external peer review.
Peer Review File: Available at https://tau.amegroups.com/article/view/10.21037/tau-23-602/prf
Conflicts of Interest: All authors have completed the ICMJE uniform disclosure form (available at https://tau.amegroups.com/article/view/10.21037/tau-23-602/coif). B.G. and P.S. report the collaborative works of their lab are supported by the Actions de Recherche Concertées program of the Communauté Française de Belgique (ARC 21/26-118). B.G. reports research grants from the Fonds National de la Recherche Scientifique (FNRS) (CDR J.0084.22). B.M. is a Research Fellow of the Belgian Fonds National de la Recherche Scientifique (F.R.S.-FNRS). P.S. reports that he is the inventor of patent application EP21175397.5 “Molecular signature for assessing the responsiveness of cancer to mitochondria-targeted antioxidants” and is involved in a clinical collaboration with Antipodean Pharmaceuticals Inc. for the prevention of breast cancer metastasis; and he is a Research Director of the Belgian Fonds National de la Recherche Scientifique (F.R.S.-FNRS). The authors have no other conflicts of interest to declare.
Ethical Statement: The authors are accountable for all aspects of the work in ensuring that questions related to the accuracy or integrity of any part of the work are appropriately investigated and resolved.
Open Access Statement: This is an Open Access article distributed in accordance with the Creative Commons Attribution-NonCommercial-NoDerivs 4.0 International License (CC BY-NC-ND 4.0), which permits the non-commercial replication and distribution of the article with the strict proviso that no changes or edits are made and the original work is properly cited (including links to both the formal publication through the relevant DOI and the license). See: https://creativecommons.org/licenses/by-nc-nd/4.0/.
References
- Saraei P, Asadi I, Kakar MA, et al. The beneficial effects of metformin on cancer prevention and therapy: a comprehensive review of recent advances. Cancer Manag Res 2019;11:3295-313. [Crossref] [PubMed]
- Margel D, Urbach DR, Lipscombe LL, et al. Metformin use and all-cause and prostate cancer-specific mortality among men with diabetes. J Clin Oncol 2013;31:3069-75. [Crossref] [PubMed]
- Patel T, Hruby G, Badani K, et al. Clinical outcomes after radical prostatectomy in diabetic patients treated with metformin. Urology 2010;76:1240-4. [Crossref] [PubMed]
- Morgans AK, Chen YH, Jarrard DF, et al. Association between baseline body mass index and survival in men with metastatic hormone-sensitive prostate cancer: ECOG-ACRIN CHAARTED E3805. Prostate 2022;82:1176-85. [Crossref] [PubMed]
- Papachristodoulou A, Heidegger I, Virk RK, et al. Metformin Overcomes the Consequences of NKX3.1 Loss to Suppress Prostate Cancer Progression. Eur Urol 2024;85:361-72. [Crossref] [PubMed]
- Rena G, Hardie DG, Pearson ER. The mechanisms of action of metformin. Diabetologia 2017;60:1577-85. [Crossref] [PubMed]
- Zhao B, Luo J, Yu T, et al. Anticancer mechanisms of metformin: A review of the current evidence. Life Sci 2020;254:117717. [Crossref] [PubMed]
- Chiang CF, Chao TT, Su YF, et al. Metformin-treated cancer cells modulate macrophage polarization through AMPK-NF-κB signaling. Oncotarget 2017;8:20706-18. [Crossref] [PubMed]
- Dallaglio K, Bruno A, Cantelmo AR, et al. Paradoxic effects of metformin on endothelial cells and angiogenesis. Carcinogenesis 2014;35:1055-66. [Crossref] [PubMed]
- Tossetta G. Metformin Improves Ovarian Cancer Sensitivity to Paclitaxel and Platinum-Based Drugs: A Review of In Vitro Findings. Int J Mol Sci 2022;23:12893. [Crossref] [PubMed]
- Wheaton WW, Weinberg SE, Hamanaka RB, et al. Metformin inhibits mitochondrial complex I of cancer cells to reduce tumorigenesis. Elife 2014;3:e02242. [Crossref] [PubMed]
- Andrzejewski S, Gravel SP, Pollak M, et al. Metformin directly acts on mitochondria to alter cellular bioenergetics. Cancer Metab 2014;2:12. [Crossref] [PubMed]
- Mayer MJ, Klotz LH, Venkateswaran V. Metformin and prostate cancer stem cells: a novel therapeutic target. Prostate Cancer Prostatic Dis 2015;18:303-9. [Crossref] [PubMed]
- Loubière C, Goiran T, Laurent K, et al. Metformin-induced energy deficiency leads to the inhibition of lipogenesis in prostate cancer cells. Oncotarget 2015;6:15652-61. [Crossref] [PubMed]
- Scotland S, Saland E, Skuli N, et al. Mitochondrial energetic and AKT status mediate metabolic effects and apoptosis of metformin in human leukemic cells. Leukemia 2013;27:2129-38. [Crossref] [PubMed]
- Finisguerra V, Dvorakova T, Formenti M, et al. Metformin improves cancer immunotherapy by directly rescuing tumor-infiltrating CD8 T lymphocytes from hypoxia-induced immunosuppression. J Immunother Cancer 2023;11:e005719. [Crossref] [PubMed]
- Chowdhury AR, Zielonka J, Kalyanaraman B, et al. Mitochondria-targeted paraquat and metformin mediate ROS production to induce multiple pathways of retrograde signaling: A dose-dependent phenomenon. Redox Biol 2020;36:101606. [Crossref] [PubMed]
- d'Hose D, Danhier P, Northshield H, et al. A versatile EPR toolbox for the simultaneous measurement of oxygen consumption and superoxide production. Redox Biol 2021;40:101852. [Crossref] [PubMed]
- d'Hose D, Mathieu B, Mignion L, et al. EPR Investigations to Study the Impact of Mito-Metformin on the Mitochondrial Function of Prostate Cancer Cells. Molecules 2022;27:5872. [Crossref] [PubMed]
- Marzioni D, Mazzucchelli R, Fantone S, et al. NRF2 modulation in TRAMP mice: an in vivo model of prostate cancer. Mol Biol Rep 2023;50:873-81. [Crossref] [PubMed]
- Tossetta G, Fantone S, Marzioni D, et al. Cellular Modulators of the NRF2/KEAP1 Signaling Pathway in Prostate Cancer. Front Biosci (Landmark Ed) 2023;28:143. [Crossref] [PubMed]
- Tossetta G, Fantone S, Marzioni D, et al. Role of Natural and Synthetic Compounds in Modulating NRF2/KEAP1 Signaling Pathway in Prostate Cancer. Cancers (Basel) 2023;15:3037. [Crossref] [PubMed]
- Shen MM, Abate-Shen C. Roles of the Nkx3.1 homeobox gene in prostate organogenesis and carcinogenesis. Dev Dyn 2003;228:767-78. [Crossref] [PubMed]
- Papachristodoulou A, Rodriguez-Calero A, Panja S, et al. NKX3.1 Localization to Mitochondria Suppresses Prostate Cancer Initiation. Cancer Discov 2021;11:2316-33. [Crossref] [PubMed]
- Subbiah R, Tiwari RR. The herbicide paraquat-induced molecular mechanisms in the development of acute lung injury and lung fibrosis. Crit Rev Toxicol 2021;51:36-64. [Crossref] [PubMed]
- Kalyanaraman B, Cheng G, Hardy M, et al. Teaching the basics of reactive oxygen species and their relevance to cancer biology: Mitochondrial reactive oxygen species detection, redox signaling, and targeted therapies. Redox Biol 2018;15:347-62. [Crossref] [PubMed]
- Porporato PE, Payen VL, Pérez-Escuredo J, et al. A mitochondrial switch promotes tumor metastasis. Cell Rep 2014;8:754-66. [Crossref] [PubMed]
- Scheinok S, Capeloa T, Porporato PE, et al. An EPR Study Using Cyclic Hydroxylamines To Assess The Level of Mitochondrial ROS in Superinvasive Cancer Cells. Cell Biochem Biophys 2020;78:249-54. [Crossref] [PubMed]
- Capeloa T, Krzystyniak J, d'Hose D, et al. MitoQ Inhibits Human Breast Cancer Cell Migration, Invasion and Clonogenicity. Cancers (Basel) 2022;14:1516. [Crossref] [PubMed]
- Capeloa T, Van de Velde JA, d'Hose D, et al. Inhibition of Mitochondrial Redox Signaling with MitoQ Prevents Metastasis of Human Pancreatic Cancer in Mice. Cancers (Basel) 2022;14:4918. [Crossref] [PubMed]