BRD4 plays an antiaging role in the senescence of renal tubular epithelial cells
Highlight box
Key findings
• BRD4 suppressed age-related kidney failure via ameliorating D-galactose (D-gal)-induced renal injury in vivo and in vitro. BRD4 inhibitors could promote renal aging and impair renal function.
What is known and what is new?
• Aging leads to a significant decrease in BRD4 expression in renal tubular cells.
• BRD4 has the potential to reverse some aging-related physiological phenomena induced by D-gal in renal tubular cells.
What is the implication, and what should change now?
• Activation of BRD4 can prevent the aging of renal tubular cells. So, BRD4 is a promising drug target for treating aging-related kidney injury.
Introduction
Aging, a natural process leading to organ function deterioration, is driven by multiple molecular mechanisms, such as cellular senescence (1). Senescence was initially described by Leonard Hayflick as a condition in which the proliferative capacity of non-immortalized human cells in culture was arrested in response to various stimuli. Cellular senescence is a state of irreversible cycle arrest in which the cell becomes resistant to growth-promoting stimuli (2). Similar to autophagy and apoptosis, senescence restricts the propagation of defective cells and maintains tissue integrity and function (3). Common stimuli of senescence include oncogenic activation, cytokine release, reactive oxygen species (ROS) overproduction, DNA damage, and nucleotide depletion (4). D-galactose (D-gal) is an aldohexose. At high levels, it can be converted into aldose and hydroperoxide under the catalysis of galactose oxidase, producing ROS. D-gal is most preferred due to its convenience, less side effects, and the higher survival rate throughout the experimental period, and it has been proven to artificially induce cellular senescence in vitro and in vivo for anti-aging therapeutic interventions (5).
Aging renders the kidneys more susceptible to internal toxins (6). End-stage renal disease results from progressive structural and functional deterioration of renal tubular epithelial cells (7). During the senescence of renal tubular epithelial cells, mitochondrial dysfunction and DNA repair deficiency lead to cell cycle arrest, apoptosis and renal fibrosis (8). The senescence of renal tubular epithelial cells can be regulated to treat aging-related kidney disease. Therefore, targeting the senescence of renal tubular epithelial cells has become a therapeutic approach for treating kidney injury (9).
In recent years, the role of epigenetic regulation in cellular senescence has garnered much attention (10). Upon stimulation, epigenetic regulation initiates cell cycle arrest, DNA repair deficiency, senescence, and apoptosis.
The bromodomain and extraterminal (BET) family of bromodomains (BRDs) are well-known drug targets for many human diseases. BRD4, a member of the BET protein family, has been shown to be a transcriptional and epigenetic regulator in the organization and expression of genes (11). Inhibition of BRD4 can block the communication between the super-enhancer (SE) and the promoter region of the target genes, with subsequent cell-specific repression to induce cell death (12). Cells with BRD4 knockdown grow slowly and are arrested at the G0/G1 phase; however, reintroduction of BRD4 rescues G1 gene expression and promotes the cells to S phase (13).
Currently, the role of BRD4 in senescence is largely unexplored. Emerging evidence reveals that BRD4 maintains genomic integrity by reducing DNA damage (14). Lee et al. reported that BRD4 is required for adipogenesis and myogenesis due to its maintenance of cell-specific gene expression (15). BRD4 can also activate the DNA damage checkpoint and maintain telomeres in a non-transcriptional manner, thus trapping cells into senescence or apoptosis. Herein, we investigated the biological function of BRD4 in the aging of kidneys in cells and animals and figured out related molecular mechanisms. We present this article in accordance with the ARRIVE and MDAR reporting checklists (Available at https://tau.amegroups.com/article/view/10.21037/tau-24-214/rc).
Methods
Culture of renal tubular epithelial cells
Human proximal tubular epithelial cells (HRPTEpiCs) were purchased from ScienCell (Carlsbad, CA, USA) and cultured in medium at 37 ℃ in 95% air with 5% CO2.
Cell transfection
The cells were incubated with lentiviral vectors (Genechem LLC, Shanghai, China) according to the manufacturer’s protocol. First, cells were seeded in 70–90% confluent in 24-well. Second, the supernatant was removed, medium was replaced without fetal bovine serum (FBS) and the lentiviral vectors were added. At last, after incubation for 12 hours, the culture supernatant was removed and replaced with complete growth medium. The cells were collected 48 hours after transfection for other analyses.
3-(4,5-dimethylthiazol-2-yl)-2,5-diphenyltetrazolium bromide (MTT) assay
Cell viability was determined by MTT assay. First, the cells were seeded in 96-well plates (5×103/well) for 24 hours and then stimulated with D-gal (Selleck Chemicals LLC, TX, USA) in different concentrations (50, 100, 150, 200 mM) for 24 hours. MTT solution (5 mg/mL) was added and incubated for 4 hours, and dimethyl sulfoxide (DMSO) was added to dissolve the crystals. Absorbance was measured by the plate reader at a wavelength of 492 nm.
Cell migration assay
Cell migration was assayed in 24-well, 6.5-mm-internal-diameter transwell plates (8.0 µm pore size; Costar Corp.). Cells were placed on the upper chambers, and the lower chamber was filled with medium containing FBS. Cells were allowed to migrate. After 24 hours, cells on the upper surfaces of the chambers were removed by cotton swabs and the migrated cells on the undersides of the chambers were fixed with methanol, stained with crystal violet, and photographed by microscope.
Annexin V-propidium iodide (PI) staining assay for apoptosis analysis
Apoptosis of the cells was evaluated using Annexin V-PI staining. The cells were treated with various concentrations of D-gal for 24 hours, collected, washed with phosphate-buffered saline (PBS), stained with Annexin V-PI for 5 minutes in the dark, and finally analyzed by fluorescence-activated cell sorting (FACS).
PI staining assay for cell cycle analysis
Apoptosis of the cells was evaluated using PI staining (Thermo Fisher Scientific, NA, USA). The cells were treated with various concentrations of D-gal for 24 hours, collected, washed with PBS, stained with PI for 5 minutes in the dark, and finally analyzed by FACS.
Senescence-associated β-galactosidase (SA-β-gal) staining
Senescence was evaluated according to SA-β-gal activity in cultured cells, a typical indicator of senescence. The cells were treated with various concentrations of D-gal for 24 hours on 35 mm dish in a 5% carbon dioxide (CO2) incubator. After removing the culture medium, the cells were washed with PBS. Two mL of 4% paraformaldehyde (PFA)/PBS solution was added to the dish and the cells were incubated at room temperature. The supernatant was removed, and the cells were washed with PBS for three times. The cells were stained with SA-β-gal staining (Beyotime Biotechnology, Shanghai, China) working solution and incubated at 37 ℃ incubator without CO2 for 30 minutes. After removing the supernatant, the cells were washed with PBS and observed by microscope.
Western blotting (WB)
Protein content was measured using the Bradford method. After sodium dodecyl sulfonate polyacrylamide gel electrophoresis (SDS-PAGE), the proteins were transferred to polyvinylidene fluoride (PVDF) membranes. The membrane was incubated with anti-p16 (Abcam, ab189034, 1:1,000; Abcam, Cambridge, MA, USA), BRD4 (Cell Signaling Technology, 13440, 1:1,000, Danvers, MA, USA) and anti-GAPDH (Cell Signaling Technology, 5174, 1:1,000) antibodies at 4 ℃ overnight. After washing, the membrane was incubated with the secondary antibody (Cell Signaling Technology, 7074/7076, 1:5,000) at 1:10,000 for 2 hours and then washed again. Substrate ChemStation scanning (Agilent, Santa Clara, CA, USA) for color analysis was performed with Tanon Gis software (Tanon, Shanghai, China).
Mouse model
All male mouse experiments were conducted under protocols approved by the Animal Care and Use Committee of Jiangsu Province Academy of Nanjing Medical University (No. 2201040), in compliance with Nanjing Medical University guidelines for the care and use of animals. A protocol was prepared before the study without registration. We obtained 8-week-old C57BL/6 mice from the Aniphe BioLab Company (Jiangsu, China). The animals were kept at 22±1 ℃ in a 12-hour light-dark cycle (6–18 hours) with free access to water and food. The physical and mental parameters of the animals were recorded on a daily basis.
Experimental design
The mice were randomly assigned to three experimental groups: a control group (CTL) that received intraperitoneal (IP) saline solution (NaCl 0.9%), a D-gal group (DGAL) that received D-gal at 150 mg/kg via IP (n=8 animals/per group). The mice in these first two groups were subjected to daily treatment for 8 weeks. The third group was DbBETi (Abbv-075) that received Abbv-075 1.5 mg/kg by oral gavage (16) for 10 days (n=8 animals/per group). The IP was standardized as a volume less than or equal to 2 mL/kg. D-gal was dosed at 150 mg/kg (as reported in the literature) to induce aging in the mice.
Biochemical analysis
At the end of week 1 and 2, blood samples were collected immediately after sacrificing the mice. The blood was centrifuged at 1,500 g and 4 ℃ for 15 minutes, and then the contents of blood urea nitrogen (BUN), serum creatinine (sCr), uric acid (UA), and cystatin C (Cys C) were measured using a Beckman II Analyzer (Beckman Coulter Instruments, Fullerton, CA, USA).
Histopathological analysis
The mouse kidney tissues were fixed in paraformaldehyde and then embedded in paraffin. Immunohistochemistry (IHC) assays were performed as previously described (17). The renal tissue sections were incubated with antibodies against BRD4 and P21 (Santa Cruz Biotechnology, SC-6246, 1:100, TX, USA) at 4 ℃ overnight, followed by incubation with a secondary antibody. Finally, the sections were counterstained with Mayer’s hematoxylin.
Statistical analysis
SPSS 20.0 software (IBM Corp., Armonk, NY, USA) was used for statistical analysis. Single-factor analysis of variance (ANOVA) was used to compare data among the experimental groups. The results were expressed as the mean ± standard deviation. P<0.05 was considered statistically significant.
Results
D-gal induces HRPTEpiC senescence and renal injury
Cell viability measured by MTT assay showed that D-gal suppressed the proliferation of HRPTEpiC in a time- and concentration-dependent manner (Figure 1A). Next, we measured the levels of senescence-related markers. The level of SA-β-gal-positive cells among HRPTEpiCs after D-gal treatment was significantly higher than that in the control group (Figure 1B). Cell cycle arrest, a hallmark of cellular senescence (18), was induced by D-gal, implying that D-gal inhibited the proliferation of HRPTEpiCs (Figure 1C). Additionally, D-gal remarkably upregulated the expression levels of senescence-related proteins p16 in HRPTEpiCs (Figure 1D). In vivo, D-gal significantly increased the levels of renal function-related markers, such as Cys C, BUN, and sCr (Figure 1E).
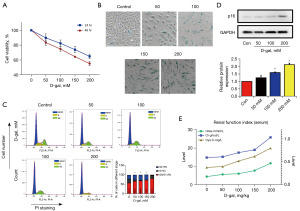
A BRD4 inhibitor induces HRPTEpiC senescence and renal injury
BRD4 is a pivotal transcriptional and epigenetic regulator during embryogenesis and development (19). Here, we focused on whether it plays an essential role in the senescence of tubular epithelial cells. We quantified the protein expression of BRD4 in D-gal-treated cells and a mouse senescence model. D-gal significantly decreased the protein expression of BRD4, which was significantly decreased by D-gal in vivo (Figure 2A,2B). It was further revealed that Abbv-075 (Selleck Chemicals LLC), an inhibitor of BRD4, could inhibit the protein expression of BRD4 in HRPTEpiCs (Figure 2C). The SA-β-gal activity of HRPTEpiCs increased notably after treatment with Abbv-075 (Figure 2D). In addition, Abbv-075 notably aggravated 24-hour albuminuria and decreased the weight of C57/BL6 mice (Figure 2E). IHC results also showed that Abbv-075 increased the expression levels of senescence-related proteins (Figure 2F). These results verified the involvement of BRD4 in D-gal-induced cellular senescence.
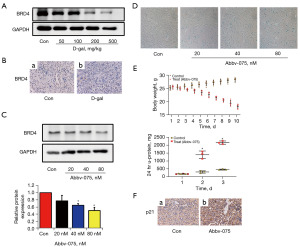
Knockdown of BRD4 induces HRPTEpiC senescence
To further prove that inhibiting BRD4 could induce cell senescence, the expression of BRD4 was knocked down by short hairpin RNA (shRNA). The SA-β-gal activity in HRPTEpiCs increased dramatically after BRD4 shRNA treatment (Figure 3A). Senescent cells are generally believed to exert an irreversible arrest in the G0/G1 stage of the cell cycle. Knockdown of BRD4 also inhibited cell migration and increased the proportion of cells in the G0/G1 phase (Figure 3B,3C). Additionally, the knockdown also promoted the expression of the senescence-related proteins p16 (Figure 3D). In summary, the knockdown of BRD4 could induce the senescence of HRPTEpiCs.
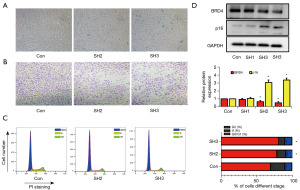
Overexpression of BRD4 rescues D-gal-induced HRPTEpiC senescence
Next, we designed an experiment to confirm whether the overexpression of BRD4 could alleviate cell senescence. As BRD4 was overexpressed, the migration and viability of HRPTEpiCs were enhanced, as shown in the 3D migration assay (Figure 4A). As expected, D-gal-induced apoptosis and cell cycle arrest were rescued (Figure 4B,4C). Taken together, BRD4 reversed the senescence of D-gal-treated tubular epithelial cells.
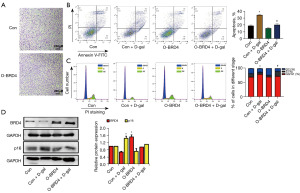
BRD4 downregulates the P16 protein expression to alleviate D-gal-induced HRPTEpiC senescence
The p16 tumor suppressor (CDKN2A) is also involved in the cell cycle and senescence (20). We focused on p16 because of its well-established role in controlling cell proliferation, survival, and senescence. We found that overexpression BRD4 did not downregulate the protein expression of p16, but it could reverse the down-expression of p16 induced by D-gal (Figure 4D). Therefore, BRD4 attenuated D-gal-induced renal tubule senescence.
Discussion
Worldwide, life expectancy has reached an unprecedented peak. By the middle of the 21st century, the global population aged over 65 years is expected to exceed 1.5 billion (21). Aging is the major risk factor causing the molecular and physiological changes that lead to impairment of the renal function and regenerative potential (22). With the growth of the aging population, one of our challenges is to understand the basic biological mechanisms underlying these changes that accompany aging, as these changes increase the risk of developing acute kidney injury (AKI) and chronic kidney disease (CKD) (23). A research has shown that specific cellular biologic events and signal pathways of lipotoxicity, oxidative stress, and inflammation are involved in the complex process of kidney aging (24). In this study, we highlight experimental findings about the molecular mechanisms of cellular senescence in renal aging that pose challenges in therapeutic aspects of senescence. This would provide the great potential of anti-senescent therapies for alleviating the harmful senescent to avoid age-related AKI and CKD.
In the present study, we found that BRD4 attenuated D-gal-induced senescence of renal tubular cells, which contributes to aging-related renal diseases. Inspired by this finding, more in-depth studies and even potential therapeutic options can be expected.
Kidney tubular epithelial cells undergo pathological changes crucial for the development of aging-related CKD, but the related molecular mechanisms and interventional strategies remain obscure (7). BRD4 acts as a genetic reader of histone acetyl-lysine residues to regulate gene transcription. BRD4 has shown therapeutic potential in a variety of human diseases, including cancer, inflammation, nervous system disorders, and cardiovascular diseases (25). Recently, Zhang et al. reported that degradation and inhibition of BRD4 exacerbate Alzheimer’s disease-related neuropathy in cell models by increasing the levels of BACE1 (26). BACE1, which is also expressed in renal tubular epithelial cells, increases renal damage in aging mice (27). In this study, we found that BRD4 functioned actively in the senescence of kidney tubular epithelial cells.
D-gal is considered an authoritative agent in inducing aging in animal models (28). D-gal, an aldohexose and a monosaccharide sugar, is a reducing sugar that is present naturally in the body and in different kinds of food such as milk, yogurt, cherries, and celery (29). After ingestion, the maximal daily dose of D-gal that can be metabolized and excreted by the body is 50 g within about 8 hours for a healthy adult (30). Amounts in excess of this limit can increase the level of ROS and cause oxidative stress, inflammation, mitochondrial dysfunction, and apoptosis (31). In China, the first researchers using D-gal for the study of senescence discovered that injections of it could reduce longevity in rodents (5). Since this discovery, a study has utilized this model and it has been well confirmed to mimic the changes of some aging markers such as advanced glycation end (AGE) products, telomere length shortening, senescence-associated genes (P16, P21, P53), and senescence-associated SA-β-gal staining (32). The increased AGEs may give rise to age-related kidney failure (33). In this study, we used D-gal to mimic the senescence to investigate the possible underlying mechanisms of aging-related kidney injury.
According to our results, D-gal inhibited the expression of BRD4 and induced aging in tubular epithelial cells in vivo and in vitro. The pan-BRD4 inhibitor (Abbv-075) could also mimic D-gal-induced aging. To further confirm this conclusion, downregulated protein expression of BRD4 may lead to tubular epithelial cell injury and subsequent renal dysfunction. To explore its role in tubular epithelial cell senescence, we overexpressed BRD4 in primary cells with a lentiviral vector. To a certain extent, upregulation of BRD4 alleviated D-gal-induced senescence of renal tubular epithelial cells. In contrast, downregulating BRD4 with the inhibitor directly induced primary tubular epithelial cell senescence and G0/G1 cell cycle arrest, an effect similar to that of D-gal. G0/G1 phase cycle arrest is a critical step in cellular senescence (34). P16, a 16 kDa protein encoded by the CDKN2A gene and a member of the INK family of CDKIs, can regulate the entry of cells to the G1-S-phase transition checkpoint (35). In addition, p16 inhibits the function of CDK4/6-cyclin D, a protein complex catalyzing the progression to G1 phase (36). It also downregulates the phosphorylation of retinoblastoma (RB), which promotes the formation of the RB-E2F complex to induce the cell-cycle arrest (37). Therefore, upregulation of p16 may initiate the process of senescence by arresting cell growth. In the current study, we found that as BRD4 overexpression down-regulated the protein expression of p16 induced by D-gal, the senescence of kidney tubular epithelial cells induced by D-gal was remarkably alleviated.
Conclusions
In recent studies, the importance of BET protein BRD4 has been investigated and characterized in the senescent processes of the kidney. In this study, we showed that BRD4 inhibitors could promote renal senescence and impair renal function. And the biological function of BRD4 declines in elderly patients with chronic kidney disease. Therefore, restoring the normal physiological function of BRD4 in these patients can help slow the progression of chronic kidney disease. So, BRD4 may be a potential therapeutic biomarker for aging-related kidney diseases.
Acknowledgments
Funding: This work was supported by the grants from
Footnote
Reporting Checklist: The authors have completed the ARRIVE and MDAR reporting checklists. Available at https://tau.amegroups.com/article/view/10.21037/tau-24-214/rc
Data Sharing Statement: Available at https://tau.amegroups.com/article/view/10.21037/tau-24-214/dss
Peer Review File: Available at https://tau.amegroups.com/article/view/10.21037/tau-24-214/prf
Conflicts of Interest: All authors have completed the ICMJE uniform disclosure form (available at https://tau.amegroups.com/article/view/10.21037/tau-24-214/coif). The authors have no conflicts of interest to declare.
Ethical Statement:
Open Access Statement: This is an Open Access article distributed in accordance with the Creative Commons Attribution-NonCommercial-NoDerivs 4.0 International License (CC BY-NC-ND 4.0), which permits the non-commercial replication and distribution of the article with the strict proviso that no changes or edits are made and the original work is properly cited (including links to both the formal publication through the relevant DOI and the license). See: https://creativecommons.org/licenses/by-nc-nd/4.0/.
References
- Childs BG, Durik M, Baker DJ, et al. Cellular senescence in aging and age-related disease: from mechanisms to therapy. Nat Med 2015;21:1424-35. [Crossref] [PubMed]
- Takahashi A, Ohtani N, Hara E. Irreversibility of cellular senescence: dual roles of p16INK4a/Rb-pathway in cell cycle control. Cell Div 2007;2:10. [Crossref] [PubMed]
- He S, Sharpless NE. Senescence in Health and Disease. Cell 2017;169:1000-11. [Crossref] [PubMed]
- Mijit M, Caracciolo V, Melillo A, et al. Role of p53 in the Regulation of Cellular Senescence. Biomolecules 2020;10:420. [Crossref] [PubMed]
- Azman KF, Zakaria R. D-Galactose-induced accelerated aging model: an overview. Biogerontology 2019;20:763-82. [Crossref] [PubMed]
- Hirakawa Y, Jao TM, Inagi R. Pathophysiology and therapeutics of premature ageing in chronic kidney disease, with a focus on glycative stress. Clin Exp Pharmacol Physiol 2017;44:70-7. [Crossref] [PubMed]
- Denic A, Glassock RJ, Rule AD. Structural and Functional Changes With the Aging Kidney. Adv Chronic Kidney Dis 2016;23:19-28. [Crossref] [PubMed]
- Franzin R, Stasi A, Ranieri E, et al. Targeting Premature Renal Aging: from Molecular Mechanisms of Cellular Senescence to Senolytic Trials. Front Pharmacol 2021;12:630419. [Crossref] [PubMed]
- Zhang Q, Qi J, Luo Q, et al. Yishen Xiezhuo formula ameliorates the development of cisplatin-induced acute kidney injury by attenuating renal tubular epithelial cell senescence. Ann Transl Med 2022;10:1392. [Crossref] [PubMed]
- Sidler C, Kovalchuk O, Kovalchuk I. Epigenetic Regulation of Cellular Senescence and Aging. Front Genet 2017;8:138. [Crossref] [PubMed]
- Donati B, Lorenzini E, Ciarrocchi A. BRD4 and Cancer: going beyond transcriptional regulation. Mol Cancer 2018;17:164. [Crossref] [PubMed]
- He Y, Long W, Liu Q. Targeting Super-Enhancers as a Therapeutic Strategy for Cancer Treatment. Front Pharmacol 2019;10:361. [Crossref] [PubMed]
- Mochizuki K, Nishiyama A, Jang MK, et al. The bromodomain protein Brd4 stimulates G1 gene transcription and promotes progression to S phase. J Biol Chem 2008;283:9040-8. [Crossref] [PubMed]
- Lam FC, Kong YW, Huang Q, et al. BRD4 prevents the accumulation of R-loops and protects against transcription-replication collision events and DNA damage. Nat Commun 2020;11:4083. [Crossref] [PubMed]
- Lee JE, Park YK, Park S, et al. Brd4 binds to active enhancers to control cell identity gene induction in adipogenesis and myogenesis. Nat Commun 2017;8:2217. [Crossref] [PubMed]
- Faivre EJ, McDaniel KF, Albert DH, et al. Selective inhibition of the BD2 bromodomain of BET proteins in prostate cancer. Nature 2020;578:306-10. [Crossref] [PubMed]
- Kim SW, Roh J, Park CS. Immunohistochemistry for Pathologists: Protocols, Pitfalls, and Tips. J Pathol Transl Med 2016;50:411-8. [Crossref] [PubMed]
- Hernandez-Segura A, Nehme J, Demaria M. Hallmarks of Cellular Senescence. Trends Cell Biol 2018;28:436-53. [Crossref] [PubMed]
- Devaiah BN, Gegonne A, Singer DS. Bromodomain 4: a cellular Swiss army knife. J Leukoc Biol 2016;100:679-86. [Crossref] [PubMed]
- Wlaschek M, Maity P, Makrantonaki E, et al. Connective Tissue and Fibroblast Senescence in Skin Aging. J Invest Dermatol 2021;141:985-92. [Crossref] [PubMed]
- Knickman JR, Snell EK. The 2030 problem: caring for aging baby boomers. Health Serv Res 2002;37:849-84. [Crossref] [PubMed]
- Weinstein JR, Anderson S. The aging kidney: physiological changes. Adv Chronic Kidney Dis 2010;17:302-7. [Crossref] [PubMed]
- Rex N, Melk A, Schmitt R. Cellular senescence and kidney aging. Clin Sci (Lond) 2023;137:1805-21. [Crossref] [PubMed]
- Yuan Q, Tang B, Zhang C. Signaling pathways of chronic kidney diseases, implications for therapeutics. Signal Transduct Target Ther 2022;7:182. [Crossref] [PubMed]
- Liu Z, Wang P, Chen H, et al. Drug Discovery Targeting Bromodomain-Containing Protein 4. J Med Chem 2017;60:4533-58. [Crossref] [PubMed]
- Zhang S, Bai P, Lei D, et al. Degradation and inhibition of epigenetic regulatory protein BRD4 exacerbate Alzheimer's disease-related neuropathology in cell models. J Biol Chem 2022;298:101794. [Crossref] [PubMed]
- Shi Y, Gao F, Yang X, et al. Increase of BACE1, Brain-Renal Risk Factor, Contributes to Kidney Damage in an Alzheimer's Disease Mouse Model. J Alzheimers Dis 2020;76:237-48. [Crossref] [PubMed]
- Zheng S. Protective effect of Polygonatum sibiricum Polysaccharide on D-galactose-induced aging rats model. Sci Rep 2020;10:2246. [Crossref] [PubMed]
- Acosta PB, Gross KC. Hidden sources of galactose in the environment. Eur J Pediatr 1995;154:S87-92. [Crossref] [PubMed]
- Gupta A, Singh AK, Kumar R, et al. Neuroprotective Potential of Ellagic Acid: A Critical Review. Adv Nutr 2021;12:1211-38. [Crossref] [PubMed]
- Ullah F, Ali T, Ullah N, et al. Caffeine prevents d-galactose-induced cognitive deficits, oxidative stress, neuroinflammation and neurodegeneration in the adult rat brain. Neurochem Int 2015;90:114-24. [Crossref] [PubMed]
- Shwe T, Pratchayasakul W, Chattipakorn N, et al. Role of D-galactose-induced brain aging and its potential used for therapeutic interventions. Exp Gerontol 2018;101:13-36. [Crossref] [PubMed]
- Haider S, Liaquat L, Shahzad S, et al. A high dose of short term exogenous D-galactose administration in young male rats produces symptoms simulating the natural aging process. Life Sci 2015;124:110-9. [Crossref] [PubMed]
- Makpol S, Durani LW, Chua KH, et al. Tocotrienol-rich fraction prevents cell cycle arrest and elongates telomere length in senescent human diploid fibroblasts. J Biomed Biotechnol 2011;2011:506171. [Crossref] [PubMed]
- Gao H, Nepovimova E, Heger Z, et al. Role of hypoxia in cellular senescence. Pharmacol Res 2023;194:106841. [Crossref] [PubMed]
- Silwal P, Nguyen-Thai AM, Mohammad HA, et al. Cellular Senescence in Intervertebral Disc Aging and Degeneration: Molecular Mechanisms and Potential Therapeutic Opportunities. Biomolecules 2023;13:686. [Crossref] [PubMed]
- Bateman G, Guo-Parke H, Rodgers AM, et al. Airway Epithelium Senescence as a Driving Mechanism in COPD Pathogenesis. Biomedicines 2023;11:2072. [Crossref] [PubMed]