A review of tanshinone compounds in prostate cancer treatment
Introduction
Prostate cancer (PCa) is the second most common cancer in men, which occurs more frequently in developed countries than in developing countries. In 2021, there were an estimated approximate 250,000 cases and 30,000 deaths in the American population; thus, causing immense pressure on medical and health work in America and globally (1-4). The current treatment methods for PCa include androgen deprivation, radical prostatectomy, local radiotherapy or brachytherapy, and chemotherapy. Androgen deprivation method has become the mainstay option of treatment for advanced PCa. However, about 90% of PCa patients eventually progress to castration-resistant prostate cancer (CRPC) in 2 years, which ultimately leads to death (5-8). Because there is no cure for CRPC, experimental and clinical trials on CRPC have been carried out around the world. Currently, the selection of chemotherapy drugs for CRPC is rather limited, encompassing docetaxel, oxaliplatin, mitoxantrone, cabazitaxel, and a few others. Furthermore, immune checkpoint inhibitors have not been found to be effective in PCa, although they alter clinical outcomes in other solid tumors. Natural botanicals play crucial roles in chemotherapy for malignant tumors. Therefore, in recent years, screening new plant-derived anticancer drugs has become a hot topic in tumor treatment.
For thousands of years, medicinal herb preparation has been used to treat various chronic diseases and infectious diseases worldwide (9,10). The World Health Organization’s prediction suggests that about 80% of people living in the developing world rely on herbal medicinal products as a primary source of healthcare (11). Furthermore, approximately 40% of the approved drugs in the market are extracted from natural plants. Salvia miltiorrhiza is the most commonly used herbal medicine in some developing countries, and it has been widely used to treat cancer, coronary heart disease, and gynecological diseases, especially amenorrhea-galactorrhea syndrome and polycystic ovary syndrome (12-14). Salviae Miltiorrhizae Radix et Rhizoma, commonly known as Danshen, is derived from the dry roots of Salvia miltiorrhiza. Within this medicinal herb, tanshinones (TANs) stand out as a unique class of abietane diterpene compounds. Since their initial isolation from Danshen by Nakao in the 1930s, more than 90 chemical constituents have been identified. These constituents can be broadly categorized into more than 40 lipophilic components and over 50 hydrophilic compounds, each offering potential medicinal benefits (15-17). In addition to the high content of polysaccharides, salvianolic acids and TANs are mainly present in Salvia miltiorrhiza (18,19). Growing evidence has shown that salvianolic acids mainly act on cardiovascular and cerebrovascular diseases (20), while TANs have been found to show potential anticancer activity (21,22). The active constituents of Salvia miltiorrhiza include liposoluble and hydrosoluble compounds. Liposoluble compounds include cryptotanshinone, TAN IIA, dihydrotanshinone I, and TAN I (Figure 1). Hydrosoluble compounds include purple oxalic acid and salvianolic acid B. In recent years, liposoluble components of TANs have been proven to have good anti-cancer activity in PCa cells. In this review, we reported the most recent research progress on the anti-tumor effects and mechanisms of TAN compounds in PCa to provide a theoretical basis for the experiments and clinical studies of these compounds.
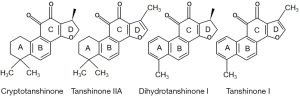
TANs inhibit cell proliferation and promote apoptosis
Signal transducer and activator of transcription 3 (STAT3) is an important intracellular signaling pathway, and it is involved in cancer cell proliferation, anti-apoptosis, immune evasion, and angiogenesis. Shin et al. verified that cryptotanshinone is an effective inhibitor of STAT3 by dual-luciferase assay. Cryptotanshinone (7 µM) can directly bind to STAT3 molecules and inhibit the growth of DU145 PCa cells by down-regulating the expression of STAT3 downstream target protein, such as survivin, Bcl-xL, and cyclin D1 (23). Other study has shown that cryptotanshinone (10 µmol/L) inhibits the proliferation and promotes the apoptosis of DU145 PCa cells in a dose- and time-dependent fashion, which may be associated with the decrease in metadherin expression and its downstream PI3K/Akt gene (24). Our research group has also previously developed a system consisting of solvent distribution and high-performance liquid chromatography (HPLC) fractionation that successfully isolated several active compounds from Salvia miltiorrhiza roots, including dihydrotanshinone I, methyltanshinonate, cryptanshinone, 1,2-dihydrotanshinquinone I, and TAN IIA. At a concentration of 7.5 or 15 µg/mL, these compounds showed an anti-proliferative effect on PCa cells; and among them, dihydrotanshinone I and methylsalvionate showed higher activities than 1,2-dihydrotanshinquinone I and TAN IIA. Their mechanisms need validation by further functional and biological experiments (25). According to another study, TAN I, cryptotanshinone, and TAN IIA inhibited the growth of human PCa cell lines (DU145 and PC-3) in a dose-dependent fashion through cell cycle arrest and induction of apoptosis in vitro. Among these compounds, TAN I showed the most potent activity with a half-maximal inhibitory concentration (IC50) value of around 3–6.5 µM, whereas the IC50 values of cryptotanshinone and TAN IIA were around 10–25 µM and 8–15 µM, respectively. Cryptotanshinone and TAN I arrested the cells in the S phase; however, TAN IIA arrested the cells in the G2/M phase. TAN I (2.5–5 µM), cryptotanshinone (10–20 µM), and TAN IIA (5–10 µM) treatments significantly down-regulated the level of CDC2. Apoptosis assay indicated that the three TANs markedly reduced the expression level of Bcl-2 in PC-3 cells; however, only TAN I increased the expression of Bax significantly. Further study demonstrated that TAN I (150 mg/kg BW, body weight, for 7 days) treatment inhibited DU145 cell growth by inducing apoptosis, inhibiting proliferation, down-regulating Aurora A protein, and inhibiting angiogenesis in vivo (26). Wang et al. found that a novel TAN analog 2-((glycine methyl ester)methyl)-naphtho (TC7) inhibited the proliferation of PC3 and LNCAP cells and initiated apoptosis by preventing cell cycle into M phase and regulating apoptosis-related proteins in vivo. More importantly, TC7 [60 mg/kg, for 18 days, intraperitoneal (IP)] treatment could reduce the tumor volume in xenograft mice (27).
TRAIL (TNF-related apoptosis-inducing ligand) is a member of the tumor necrosis factor superfamily, which can regulate apoptosis in tumor cells but does not show any toxic effects on non-cancerous cells; thus, serving as a potential therapeutic target (28,29). Shin et al. verified that co-treatment of TAN I (0, 20, 40, 80 µM) and TRAIL (0, 25, 50 ng/mL) induced apoptosis of PC-3 and DU145 cells by increasing the cleavage of PARP, activating caspases-8 and -9, and increasing accumulation of the apoptotic sub G1 portion. Also, further study showed that TAN I sensitized PCa cells to TRAIL-induced apoptosis by up-regulation of DR5 in PC-3 and DU145 cells (30). Chuang et al. confirmed that dihydrotanshinone I inhibited the proliferation and significantly induced the apoptosis of DU145 cells in a dose- and time-dependent manner. dihydrotanshinone I (1.5 µg/mL) treatment significantly increased the number of apoptotic cells compared with the control group (35.95% vs. 11.05%), and the underlying mechanism may be related to increased cleavage of PARP and cleaved form of caspases-3 and -9 in cells (31). Other study has shown that TAN IIA (50 µM) treatment reduced the viability of LNCaP cells and induced apoptosis, which were associated with activation of cleavage of pro-caspases-9 and -3, but not of pro-caspase-8, and cleavage of PARP, accompanied by reduction in the level of Bcl-2 family protein Mcl-1L, but not Bcl-2 and Bax, as well as an increase in cytochrome C and reduction in the mitochondria membrane potential. Additionally, TAN IIA (50 µM) significantly decreased the level of the PI3K p85 subunit and p-Akt and p-mTOR in LNCaP cells. This study suggested that TAN IIA-induced apoptosis was associated with the mitochondria-mediated caspase activation cascade and PI3K/Akt/mTOR signaling pathway (32). According to another study, TAN IIA (2.5–5 µg/mL) treatment significantly induced apoptosis of LNCaP cells, which was associated with an increase in the cleavage of caspases-9, -3 and PARP, indicating that TAN IIA induces apoptosis via the mitochondria-mediated apoptosis pathway (33). In addition, Li et al. demonstrated that TAN IIA (3.5 and 10 µg) induced apoptosis and autophagy in human PCa PC-3 cells through induction of reactive oxygen species (ROS) accumulation. However, ROS inhibitors could reverse the apoptosis and autophagy induced by TAN IIA (34).
Fas/APO-1 is a cell surface receptor that transduces apoptosis signals, which can mediate tumor cell apoptosis, but PCa displays resistance to Fas-induced apoptosis, highlighting that Fas/APO-1 can serve as a potential therapeutic target in PCa. Bcl-2 overexpression significantly interfered with Fas-induced apoptosis. Park et al. showed that cryptotanshinone (1 µM) significantly down-regulated the expression level of Bcl-2 by blocking JNK and p38 MAPK, remarkably enhancing the sensitivity of DU145 cells to Fas-mediated apoptosis; thus, suggesting that cryptotanshinone can be used as a Fas sensitizer to induce apoptosis (35).
TANs induce endoplasmic reticulum (ER) stress
ER stress is a protective stress response in cells, which induces endogenous apoptosis (36). Higher expression of the glucose-regulated protein (GRP78/BiP) and growth arrest- and DNA damage-inducible gene 153 (GADD153/CHOP) plays an important role in ER stress-induced apoptosis. ER stress is activated by IRE1, PERK, and ATF6 signaling pathways. Under ER stress, increased phosphorylation of eukaryotic initiation factor 2α (eIF2α) and c-Jun leads to cell apoptosis (37,38). Chuang et al. confirmed that dihydrotanshinone I (0.1–1.5 µg/mL) treatment significantly induced apoptosis of DU145 cells, which was related to ER stress. The expression levels of GRP78/BiP and CHOP/GADD153 were significantly increased and phosphorylation of PERK, its substrate, eIF2ɑ, and JNK was induced after dihydrotanshinone I treatment (31). Similarly, other study has shown that TAN IIA (2.5–5 µg/mL) treatment significantly induced apoptosis of LNCaP and PC-3 cells, which was correlated with ER stress. The expression levels of inositol-requiring protein-1α (IRE1α), GRP78/BiP, and CHOP/GADD153 were obviously up-regulated after TAN IIA treatment. In-vivo study using an LNCaP xenograft mouse model indicated that TAN IIA (60 and 90 mg/kg, for 13 days) reduced the tumor volume. Besides, Western blotting showed that GADD153/CHOP expression was up-regulated in a T2A-treated tumor. This study suggested that TAN IIA induced apoptosis of human PCa cells by induction of ER stress in vitro and in vivo (33).
TANs block the metastatic phenotype
Invasion and metastasis are one of the major malignant phenotypes of PCa cells. Epithelial-to-mesenchymal transition (EMT) enables tumor cells to acquire properties that enable easy invasion and migration. Wu et al. found that dihydrotanshinone I (5–10 µM) significantly inhibited the migration abilities of 22Rv1 cells and PC3 cells as well as of DU145 cells in PCa. Furthermore, they discovered that dihydrotanshinone I (5 µM) can inhibit the invasion ability of DU145 cells. Mechanistically, dihydrotanshinone I inhibits the migration and invasion of PCa cells via suppressing the CCL2/STAT3 axis and tumor EMT-related genes, including RhoA and SNAI1 (39). Other study has shown that dihydroisotanshinone I (5 µM) displayed radio-sensitization and anti-migration effects in PC-3 and DU145 cells via inducing DNA damage and inhibiting the expression of CCL2 (40). As we mentioned earlier in this paper, the TAN analog, TC7 (3–12 µM) inhibited the migration and invasion abilities of PC3 and LNCaP cells through down-regulating VEGF-1 expression and up-regulating matrix metalloproteinase 9 (MMP9) protein expression (27).
Modulating microRNA
MicroRNAs were single-stranded RNA molecules encoded by endogenous genes with a length of about 22 nucleotides, which play key roles in cell differentiation, proliferation, cycle regulation, and apoptosis (41). MicroRNAs are up- or down-regulated in PCa, and they were referred to as the potential therapeutic target in PCa cells (42,43). Shin et al. found that miR135a-3p expression was markedly up-regulated by co-treatment of TAN I (20 and 40 µM) and TRAIL (25 ng) in PC-3 cells. Meanwhile, overexpression of miR135a-3p increased the cytotoxicity, PARP cleavage, and the number of apoptotic cells in PC-3 cells, a similar phenomenon as that with a combination of TAN I and TRAIL (30).
TANs enhance chemosensitivity
Park et al. found that cryptotanshinone conferred sensitization of DU145 cells to apoptosis induced by etoposide, 5-fluorouracil (5-FU), cisplatin, TNF-a, and doxorubicin (DOX) (35). Sun et al. synthesized a nanodrug delivery system consisting of a prostate-specific membrane antigen (PSMA) targeted ligand with DOX and TAN, referred to as PN-DOX/TAN. The uptake rate of PN-DOX/TAN by LNCaP cells was higher than that of lipid nanoparticles loaded with DOX and TAN (N-DOX/TAN) (58.9%±1.9% vs. 36.7%±1.3%). In vivo and in vitro assays revealed that PN-DOX/TAN has a higher tumor suppressive effect on LNCaP cells (44). Qiu et al. developed a system, which is a combination of gold/polyethyleneimine (AuNPs/PEI) nanoparticles and sulphated β-cyclodextrin (CD), and then TAN IIA was integrated into the AuNPs/PEI/CD compound system. Initial experiments indicated that AuNPs/PEI/CD-T2A natural compounds were significantly more cytotoxic to PC-3 cells (IC50 =6 µM) than TAN IIA (IC50 =9.5 µM). Moreover, enzyme-linked immunosorbent assay (ELISA) test showed that AuNPs/PEI/CD-T2A increased DNA double strand breakage in PC-3 and DU145 cells when compared to TAN IIA. This study suggested that TAN IIA can obtain higher bioactivity by optimizing its chemical construction (45). Hou et al. found that TAN IIA (20 µmol/L) remarkably enhanced the anti-proliferative effects of cisplatin on PC-3 and LNCaP cells in a dose- and time-dependent manner. A combination of TAN IIA (20 µmol/L) and cisplatin (0.05 µmol/L) can induce LNCaP and PC3 cell cycle arrest in the S phase and induce apoptosis, and the underlying mechanism is activation of caspase-8, caspase-9, and caspase-3, and regulation of Bcl-2/Bax expression (46).
TANs inhibit androgen receptor (AR) signaling
Accumulating data indicate that AR signaling is closely related to the development and progression of PCa cells. Prostate-specific antigen (PSA) is one of the target genes of AR, while dihydrotestosterone (DHT) is the most effective androgen for AR activation. The present treatment strategy for metastatic PCa is to inhibit AR signaling by reducing androgen levels. The present AR antagonists include flutamide and Casodex, and PCa ultimately develops resistance to AR antagonists due to mutations in AR or an increase in the anti-apoptotic protein. Therefore, it is necessary to continue developing new drugs to improve PCa treatment and prognosis (47,48).
Zhang et al. found that TAN IIA, cryptotanshinone, and TAN I significantly inhibited the growth of androgen-dependent LNCaP cells. A colony growth assay showed that TAN IIA and cryptotanshinone had a strong tumor inhibitory effect in LNCaP cells (IC50 =0.06 µM), while TAN I showed a weak tumor inhibitory effect (IC50 =0.5 µM). Furthermore, the experimental findings revealed that the suppressive impact of TAN IIA, cryptotanshinone, and TAN I on androgen-dependent LNCaP cells was conspicuously more potent compared to their influence on androgen-independent cells, namely DU-145 and 22Rv1. In vitro, TAN IIA and cryptotanshinone inhibit LNCaP cell growth by inhibiting AR nuclear translocation, inducing AR protein degradation, and reducing the protein and mRNA expression levels of AR and PSA. In-vivo study using an LNCaP xenograft mouse model showed that TAN IIA (25 mg/kg, once a day, oral) inhibited tumor growth and decreased tumor AR expression in mice. The above data shows that TANs as potential novel anti-AR signaling agents without agonist activity (49). Ketola et al. found that TAN IIA (10 µM) inhibits the proliferation and induces apoptosis of androgen-ablated LNCaP (LNCaP-abl) cells, and its regulation mechanism is related to a decrease in the expression levels of AR and PSA (50). Liu et al. discovered that TAN IIA (2.5–5 µM) was an effective antagonist of mutated ARs and it increased maspin expression via AR. Further study showed that TAN IIA (2.5–5 µM) decreased the expression of AR and PSA and induced apoptosis in LNCaP cells. More importantly, this study found that 4,4-dimethyl on the A ring of TAN IIA derivatives plays a critical role in the anti-androgen and maspin-induced activity of TAN IIA (51). Later, Won et al. identified that TAN IIA (3 µM) induced cell cycle arrest in the G1 phase and suppressed G1 regulatory proteins, such as cyclin D1, CDK2, and CDK4, which was mediated by activation of the p53 pathway and inhibition of AR expression in LNCaP cells (52). Xu et al. suggested that cryptotanshinone (0.5 µM) inhibits the growth of AR-positive LNCaP and castration resistant 22Rv1 cells, but not of AR-negative PC-3 cells or benign prostate RWPE1 cells, through an AR-dependent pathway. Further study showed that cryptotanshinone (0.5 µM) can modulate DHT-mediated AR trans-activation and inhibit the expression of AR target proteins, such as PSA, TMPRSS2, and TMEPA1, in LNCaP cells and 22Rv1 cells. In addition, the experimental results showed that cryptotanshinone selectively inhibited AR activities, but did not repress the activities of other nuclear receptors such as estrogen receptor alpha (ERα), glucocorticoid receptor (GR), and progesterone receptor (PR). In-vivo study using a 22Rv1 xenograft PCa model indicate that cryptotanshinone (5 mg/kg or 25 mg/kg, once every other day, IP) can effectively suppress tumor growth (53). In addition, Xu et al. synthesized 25 new TAN IIA derivatives and found that seven methoxy-substituted TAN IIA derivatives showed a significant inhibitory effect on DHT-mediated AR transactivation; and among these derivatives, TAN-24 containing three oxygen methyl showed the strongest inhibitory effect. In the cytotoxicity tests on PCa cell lines, TAN-24 showed strong cytotoxicity against LNCaP and CWR22Rv1 cells, displaying IC50 values 20 times and 19 times lower than those of TAN IIA and comparable to those of enzalutamide. These results indicated that TAN-24 is a new and effective AR inhibitor and holds huge potential in treating CRPC (54).
Summary and discussion
The above-described findings strongly support the potential application of TAN IIA, TAN I, cryptotanshinone, and dihydrotanshinone I in the treatment of PCa, including CRPC, as shown in (Table 1).
Table 1
Tanshinones | Mechanisms | In-vitro | In-vivo | References |
---|---|---|---|---|
Cryptotanshinone | Inhibiting STAT3 | Inhibiting the growth of DU145 cells | ND | Shin et al. (23) |
Targeting PI3K/Akt | Inhibiting the proliferation and inducing apoptosis of DU145 cells | ND | Yao et al. (24) | |
Arresting the cell cycle and inducing apoptosis | Inhibiting growth of DU145 and PC-3 cells | ND | Gong et al. (26) | |
Sensitizing Fas/APO-1 | Inducing apoptosis of DU145 cells | ND | Park et al. (35) | |
Sensitizing etoposide, 5-FU, cisplatin, TNF-a, and doxorubicin | Inducing apoptosis of DU145 cells | ND | Park et al. (35) | |
Inhibiting AR signaling | Inhibiting the growth of LNCaP cells | ND | Zhang et al. (49) | |
Inhibiting AR signaling | Inhibiting the growth of LNCaP and CWR22Rv1 cells | Reducing tumor growth of CWR22Rv1 cells xenograft | Xu et al. (53) | |
Tanshinone IIA | Arresting the cell cycle and inducing apoptosis | Inhibiting growth of DU145 and PC-3 cells | ND | Gong et al. (26) |
Targeting PI3K/Akt/mTOR signaling and mitochondria-mediated apoptosis pathway | Inducing apoptosis of LNCaP cells | ND | Won et al. (32) | |
Inducing of ER stress and targeting mitochondria-mediated apoptosis pathway | Inducing apoptosis of LNCaP cells | Reducing tumor growth of LNCaP cells xenograft | Chiu et al. (33) | |
Inducing the accumulate of ROS | Inducing apoptosis and autophagy of PC-3 cells | ND | Li et al. (34) | |
Sensitizing doxorubicin | Inducing apoptosis of LNCaP cells | Reducing tumor growth of LNCaP cells xenograft | Sun et al. (44) | |
Sensitizing cisplatin | Arresting the cell cycle and inducing apoptosis of PC-3 and LNCaP cells | ND | Hou et al. (46) | |
inhibiting AR signaling | Inhibiting growth of LNCaP cells | Reducing tumor growth of LNCaP cells xenograft | Zhang et al. (49) | |
Inhibiting AR signaling | Inhibiting the proliferation and inducing apoptosis of LNCaP cells | ND | Ketola et al. (50); Liu et al. (51) | |
Targeting p53 and inhibiting AR expression | Inducing cell cycle arrest of LNCaP cells | ND | Won et al. (52) | |
Tanshinone I | Inducing apoptosis and inhibiting Aurora A | Inhibiting growth of DU145 and PC-3 cells | Reducing tumor growth of DU145 cells xenograft | Gong et al. (26) |
Sensitizing TRAIL and targeting apoptotic pathway | Inducing apoptosis of PC-3 and DU145 cells | ND | Shin et al. (30) | |
Regulating microRNAs | Inducing apoptosis of PC-3 cells | ND | Shin et al. (30) | |
Dihydrotanshinone I | Inducing of ER stress and targeting apoptotic pathway | Inhibiting the proliferation and inducing apoptosis of DU145 cells | ND | Chuang et al. (31) |
Inhibiting CCL2/STAT3 axis and EMT-related genes | Inhibiting migration and invasion in PC-3, DU145 and 22Rv1 cells | ND | Wu et al. (39) | |
Inducing DNA damage and inhibiting CCL2 | Sensitizing radiotherapy and Inhibiting migration of PC-3 and DU145 cells | ND | Lee et al. (40) |
STAT3, signal transducer and activator of transcription 3; PI3K, phosphoinositide 3-kinase; Akt, protein kinase B; 5-FU, 5-fluorouracil; TNF, tumor necrosis factor; AR, androgen receptor; mTOR, mammalian target of rapamycin; ER, endoplasmic reticulum; ROS, reactive oxygen species; TRAIL, TNF-related apoptosis-inducing ligand; EMT, epithelial-to-mesenchymal transition; ND, not determined.
Although these four TAN compounds belong to liposoluble components of Salvia miltiorrhiza, they appear to treat PCa through slightly different mechanisms. The chemical structures of cryptotanshinone and TAN IIA are very similar; both of them can regulate PI3K/Akt (24,32), apoptosis-related protein Bcl-2 (26), and cell cycle related protein CDC2 (26); and inhibit AR signaling, but the impact of TAN IIA is more dependent on the androgen levels. TAN IIA mainly inhibit AR nuclear translocation, decrease AR protein abundance and mRNA levels, and enhance AR proteosomal degradation. These observations suggests that TAN IIA could be a potential novel anti-AR signaling agents devoid of agonist activity. However, cryptotanshinone not only suppresses the growth of androgen-dependent cells but also acts on castration resistant 22Rv1 cells. It modulates DHT-mediated AR trans-activation and suppresses the expression of AR target proteins, including PSA, TMPRSS2, and TMEPA1, in both LNCaP and 22Rv1 cells. Experimental findings reveal that cryptotanshinone selectively inhibits AR activities without compromising the functions of other nuclear receptors like ERα, GR, and PR (49,53). While cryptotanshinone is able to regulate STAT3 (23), used as a Fas/APO-1 sensitizer (35), TAN IIA seems to target mitochondria-mediated apoptosis pathway (32,33), induce ER stress (33), initiate the accumulation of ROS (34), and regulate the p53 signaling pathway (52). TAN IIA only has been found to sensitize cisplatin and DOX (44,46); however, cryptotanshinone has been found to sensitize etoposide, 5-FU, cisplatin, TNF-a, and DOX chemotherapeutic drugs (35), as summarized in (Figure 2).
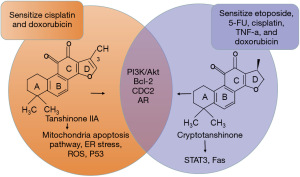
In addition, the chemical structures of TAN I and dihydrotanshinone I are very similar; both of them can regulate apoptosis-associated proteins, including PARP, caspases-8/9, and caspase-3 (30, 31). While TAN I is able to regulate Aurora A (26) and microRNAs (30), and sensitize TRAIL (30), dihydrotanshinone I seems to induce ER stress (31), regulate the CCL2/STAT3 axis (39), EMT-related genes, including RhoA and SNAI1 (39), and induce DNA damage (40), as summarized in Figure 3.
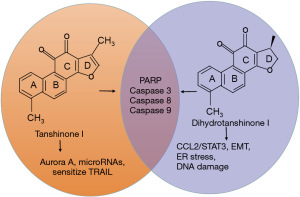
These results suggest that TANs inhibit the proliferation and growth of PCa cells by inducing apoptosis and cell cycle arrest, targeting mitochondria-mediated apoptosis pathway, regulating the PI3K/Akt, STAT3, and p53 signaling pathways, inhibiting the metastatic phenotype, inducing ER stress, regulating microRNAs, inhibiting AR signaling, and enhancing chemosensitivity. In summary, cryptotanshinone and TAN IIA appear to exert more anticancer activity against PCa; especially, TAN IIA can obtain higher bioactivity by optimizing its chemical construction and is expected to be a source of new drug development (27,44,45,54). This conclusion necessitates further verification through additional experiments and clinical practice. However, currently, there is a lack of clinical studies of TANs against PCa in humans. Thus, it is necessary to design more clinical trials of TANs in PCa patients to further verify their anti-tumor activity and toxicity.
Conclusions
TANs, including cryptotanshinone, dihydrotanshinone I, and TAN I, especially TAN IIA, exhibited great potentials in the treatment of PCa including resistant PCa.
Acknowledgments
Funding: This work was supported by
Footnote
Peer Review File: Available at https://tau.amegroups.com/article/view/10.21037/tau-24-49/prf
Conflicts of Interest: All authors have completed the ICMJE uniform disclosure form (available at https://tau.amegroups.com/article/view/10.21037/tau-24-49/coif). The authors have no conflicts of interest to declare.
Ethical Statement: The authors are accountable for all aspects of the work in ensuring that questions related to the accuracy or integrity of any part of the work are appropriately investigated and resolved.
Open Access Statement: This is an Open Access article distributed in accordance with the Creative Commons Attribution-NonCommercial-NoDerivs 4.0 International License (CC BY-NC-ND 4.0), which permits the non-commercial replication and distribution of the article with the strict proviso that no changes or edits are made and the original work is properly cited (including links to both the formal publication through the relevant DOI and the license). See: https://creativecommons.org/licenses/by-nc-nd/4.0/.
References
- do Pazo C, Webster RM. The prostate cancer drug market. Nat Rev Drug Discov 2021;20:663-4. [Crossref] [PubMed]
- Whitaker H, Tam JO, Connor MJ, et al. Prostate cancer biology & genomics. Transl Androl Urol 2020;9:1481-91. [Crossref] [PubMed]
- Komura K, Sweeney CJ, Inamoto T, et al. Current treatment strategies for advanced prostate cancer. Int J Urol 2018;25:220-31. [Crossref] [PubMed]
- Dunn MW. Prostate Cancer Screening. Semin Oncol Nurs 2017;33:156-64. [Crossref] [PubMed]
- Makino T, Izumi K, Mizokami A. Undesirable Status of Prostate Cancer Cells after Intensive Inhibition of AR Signaling: Post-AR Era of CRPC Treatment. Biomedicines 2021;9:414. [Crossref] [PubMed]
- Huang Y, Jiang X, Liang X, et al. Molecular and cellular mechanisms of castration resistant prostate cancer. Oncol Lett 2018;15:6063-76. [Crossref] [PubMed]
- Lin JZ, Wang WW, Hu TT, et al. FOXM1 contributes to docetaxel resistance in castration-resistant prostate cancer by inducing AMPK/mTOR-mediated autophagy. Cancer Lett 2020;469:481-9. [Crossref] [PubMed]
- Moussa M, Papatsoris A, Abou Chakra M, et al. Pharmacotherapeutic strategies for castrate-resistant prostate cancer. Expert Opin Pharmacother 2020;21:1431-48. [Crossref] [PubMed]
- Cui Q, Yang DH, Chen ZS. Special Issue: Natural Products: Anticancer and Beyond. Molecules 2018;23:1246. [Crossref] [PubMed]
- Pashirzad M, Johnston TP, Sahebkar A. Therapeutic Effects of Polyphenols on the Treatment of Colorectal Cancer by Regulating Wnt β-Catenin Signaling Pathway. J Oncol 2021;2021:3619510. [Crossref] [PubMed]
- Ekor M. The growing use of herbal medicines: issues relating to adverse reactions and challenges in monitoring safety. Front Pharmacol 2014;4:177. [Crossref] [PubMed]
- Shen W, Jin B, Han Y, et al. The Effects of Salvia miltiorrhiza on Reproduction and Metabolism in Women with Polycystic Ovary Syndrome: A Systematic Review and Meta-Analysis. Evid Based Complement Alternat Med 2021;2021:9971403. [Crossref] [PubMed]
- Wang L, Zhang X, Chan JY, et al. A Novel Danshensu Derivative Prevents Cardiac Dysfunction and Improves the Chemotherapeutic Efficacy of Doxorubicin in Breast Cancer Cells. J Cell Biochem 2016;117:94-105. [Crossref] [PubMed]
- Ren J, Fu L, Nile SH, et al. Salvia miltiorrhiza in Treating Cardiovascular Diseases: A Review on Its Pharmacological and Clinical Applications. Front Pharmacol 2019;10:753. [Crossref] [PubMed]
- Zhou L, Zuo Z, Chow MS. Danshen: an overview of its chemistry, pharmacology, pharmacokinetics, and clinical use. J Clin Pharmacol 2005;45:1345-59. [Crossref] [PubMed]
- Dong Y, Morris-Natschke SL, Lee KH. Biosynthesis, total syntheses, and antitumor activity of tanshinones and their analogs as potential therapeutic agents. Nat Prod Rep 2011;28:529-42. [Crossref] [PubMed]
- Wang X, Morris-Natschke SL, Lee KH. New developments in the chemistry and biology of the bioactive constituents of Tanshen. Med Res Rev 2007;27:133-48. [Crossref] [PubMed]
- Shi M, Huang F, Deng C, et al. Bioactivities, biosynthesis and biotechnological production of phenolic acids in Salvia miltiorrhiza. Crit Rev Food Sci Nutr 2019;59:953-64. [Crossref] [PubMed]
- Wang X, Yang Y, Liu X, et al. Pharmacological properties of tanshinones, the natural products from Salvia miltiorrhiza. Adv Pharmacol 2020;87:43-70. [Crossref] [PubMed]
- Chen W, Chen G. Danshen (Salvia miltiorrhiza Bunge): A Prospective Healing Sage for Cardiovascular Diseases. Curr Pharm Des 2017;23:5125-35. [PubMed]
- Fang ZY, Zhang M, Liu JN, et al. Tanshinone IIA: A Review of its Anticancer Effects. Front Pharmacol 2021;11:611087. [Crossref] [PubMed]
- Cao Y, Huang B, Gao C. Salvia miltiorrhiza extract dihydrotanshinone induces apoptosis and inhibits proliferation of glioma cells. Bosn J Basic Med Sci 2017;17:235-40. [Crossref] [PubMed]
- Shin DS, Kim HN, Shin KD, et al. Cryptotanshinone inhibits constitutive signal transducer and activator of transcription 3 function through blocking the dimerization in DU145 prostate cancer cells. Cancer Res 2009;69:193-202. [Crossref] [PubMed]
- Yao Y, Li HZ, Qian BJ, et al. Crypotanshione reduces the expression of metadherin in DU145 prostate cancer cells. Zhonghua Nan Ke Xue 2015;21:782-7. [PubMed]
- Ye QM, Ji X, Wang B, et al. Construction and Screening of Fractional Library of Salviae Miltiorrhizae Radix et Rhizoma for the Rapid Identification of Active Compounds against Prostate Cancer. J Oncol 2022;2022:9955834. [Crossref] [PubMed]
- Gong Y, Li Y, Lu Y, et al. Bioactive tanshinones in Salvia miltiorrhiza inhibit the growth of prostate cancer cells in vitro and in mice. Int J Cancer 2011;129:1042-52. [Crossref] [PubMed]
- Wang M, Zeng X, Li S, et al. A Novel Tanshinone Analog Exerts Anti-Cancer Effects in Prostate Cancer by Inducing Cell Apoptosis, Arresting Cell Cycle at G2 Phase and Blocking Metastatic Ability. Int J Mol Sci 2019;20:4459. [Crossref] [PubMed]
- Alizadeh Zeinabad H, Szegezdi E. TRAIL in the Treatment of Cancer: From Soluble Cytokine to Nanosystems. Cancers (Basel) 2022;14:5125. [Crossref] [PubMed]
- von Karstedt S, Montinaro A, Walczak H. Exploring the TRAILs less travelled: TRAIL in cancer biology and therapy. Nat Rev Cancer 2017;17:352-66. [Crossref] [PubMed]
- Shin EA, Sohn EJ, Won G, et al. Upregulation of microRNA135a-3p and death receptor 5 plays a critical role in Tanshinone I sensitized prostate cancer cells to TRAIL induced apoptosis. Oncotarget 2014;5:5624-36. [Crossref] [PubMed]
- Chuang MT, Ho FM, Wu CC, et al. 15,16-Dihydrotanshinone I, a Compound of Salvia miltiorrhiza Bunge, Induces Apoptosis through Inducing Endoplasmic Reticular Stress in Human Prostate Carcinoma Cells. Evid Based Complement Alternat Med 2011;2011:865435. [Crossref] [PubMed]
- Won SH, Lee HJ, Jeong SJ, et al. Tanshinone IIA induces mitochondria dependent apoptosis in prostate cancer cells in association with an inhibition of phosphoinositide 3-kinase/AKT pathway. Biol Pharm Bull 2010;33:1828-34. [Crossref] [PubMed]
- Chiu SC, Huang SY, Chen SP, et al. Tanshinone IIA inhibits human prostate cancer cells growth by induction of endoplasmic reticulum stress in vitro and in vivo. Prostate Cancer Prostatic Dis 2013;16:315-22. [Crossref] [PubMed]
- Li C, Han X, Zhang H, et al. The interplay between autophagy and apoptosis induced by tanshinone IIA in prostate cancer cells. Tumour Biol 2016;37:7667-74. [Crossref] [PubMed]
- Park IJ, Kim MJ, Park OJ, et al. Cryptotanshinone sensitizes DU145 prostate cancer cells to Fas(APO1/CD95)-mediated apoptosis through Bcl-2 and MAPK regulation. Cancer Lett 2010;298:88-98. [Crossref] [PubMed]
- English AR, Voeltz GK. Endoplasmic reticulum structure and interconnections with other organelles. Cold Spring Harb Perspect Biol 2013;5:a013227. [Crossref] [PubMed]
- Rao RV, Ellerby HM, Bredesen DE. Coupling endoplasmic reticulum stress to the cell death program. Cell Death Differ 2004;11:372-80. [Crossref] [PubMed]
- Ma Y, Brewer JW, Diehl JA, et al. Two distinct stress signaling pathways converge upon the CHOP promoter during the mammalian unfolded protein response. J Mol Biol 2002;318:1351-65. [Crossref] [PubMed]
- Wu CY, Yang YH, Lin YY, et al. Anti-cancer effect of danshen and dihydroisotanshinone I on prostate cancer: targeting the crosstalk between macrophages and cancer cells via inhibition of the STAT3/CCL2 signaling pathway. Oncotarget 2017;8:40246-63. [Crossref] [PubMed]
- Lee IY, Lin YY, Yang YH, et al. Dihydroisotanshinone I combined with radiation inhibits the migration ability of prostate cancer cells through DNA damage and CCL2 pathway. BMC Pharmacol Toxicol 2018;19:5. [Crossref] [PubMed]
- Lynam-Lennon N, Maher SG, Reynolds JV. The roles of microRNA in cancer and apoptosis. Biol Rev Camb Philos Soc 2009;84:55-71. [Crossref] [PubMed]
- Tang X, Tang X, Gal J, et al. Detection of microRNAs in prostate cancer cells by microRNA array. Methods Mol Biol 2011;732:69-88. [Crossref] [PubMed]
- Farooqi AA, De Rosa G. TRAIL and microRNAs in the treatment of prostate cancer: therapeutic potential and role of nanotechnology. Appl Microbiol Biotechnol 2013;97:8849-57. [Crossref] [PubMed]
- Sun G, Sun K, Sun J. Combination prostate cancer therapy: Prostate-specific membranes antigen targeted, pH-sensitive nanoparticles loaded with doxorubicin and tanshinone. Drug Deliv 2021;28:1132-40. [Crossref] [PubMed]
- Qiu S, Granet R, Mbakidi JP, et al. Delivery of tanshinone IIA and α-mangostin from gold/PEI/cyclodextrin nanoparticle platform designed for prostate cancer chemotherapy. Bioorg Med Chem Lett 2016;26:2503-6. [Crossref] [PubMed]
- Hou LL, Xu QJ, Hu GQ, et al. Synergistic antitumor effects of tanshinone II A in combination with cisplatin via apoptosis in the prostate cancer cells. Yao Xue Xue Bao 2013;48:675-9. [PubMed]
- He Y, Xu W, Xiao YT, et al. Targeting signaling pathways in prostate cancer: mechanisms and clinical trials. Signal Transduct Target Ther 2022;7:198. [Crossref] [PubMed]
- Salvi S, Conteduca V, Lolli C, et al. AR Copy Number and AR Signaling-directed Therapies in Castrationresistant Prostate Cancer. Curr Cancer Drug Targets 2018;18:869-76. [Crossref] [PubMed]
- Zhang Y, Won SH, Jiang C, et al. Tanshinones from Chinese medicinal herb Danshen (Salvia miltiorrhiza Bunge) suppress prostate cancer growth and androgen receptor signaling. Pharm Res 2012;29:1595-608. [Crossref] [PubMed]
- Ketola K, Viitala M, Kohonen P, et al. High-throughput cell-based compound screen identifies pinosylvin methyl ether and tanshinone IIA as inhibitors of castration-resistant prostate cancer. J Mol Biochem 2016;5:12-22. [PubMed]
- Liu W, Zhou J, Geng G, et al. Antiandrogenic, maspin induction, and antiprostate cancer activities of tanshinone IIA and its novel derivatives with modification in ring A. J Med Chem 2012;55:971-5. [Crossref] [PubMed]
- Won SH, Lee HJ, Jeong SJ, et al. Activation of p53 signaling and inhibition of androgen receptor mediate tanshinone IIA induced G1 arrest in LNCaP prostate cancer cells. Phytother Res 2012;26:669-74. [Crossref] [PubMed]
- Xu D, Lin TH, Li S, et al. Cryptotanshinone suppresses androgen receptor-mediated growth in androgen dependent and castration resistant prostate cancer cells. Cancer Lett 2012;316:11-22. [Crossref] [PubMed]
- Xu D, Hu H, Guan J, et al. Synthesis of novel tanshinone derivatives for treatment of castration-resistant prostate cancer. Chem Biol Drug Des 2019;94:1656-63. [Crossref] [PubMed]