Testicular compartment syndrome: an overview of pathophysiology, etiology, evaluation, and management
Introduction
Compartment syndrome disrupts the lumen of vasculature through several pathways that reinforce themselves to result in further damage. Testicular compartment syndrome (TCS) is defined as the decreased perfusion of microcirculation in testicular tissue secondary to compression, leading to hypoxia and eventually infarction of said tissue. Though compartment syndrome is traditionally not thought to occur in the testes, their sensitivity to the condition is explained by the low compliance of the tunica albuginea that increases the likelihood of capillary constriction from expansive processes. The decreased capillary perfusion within the testicle is not initially indicated by a corresponding decrease in macrocirculatory perfusion, as the larger vessels lie outside the tunica vaginalis and also require more extraluminal pressure for occlusion. In the testicle, capillary constriction secondary to TCS arises from two mechanisms: increased resistance of the pampiniform venous plexus or compressive processes from within the layers of the scrotum (1). Furthermore, ischemia/reperfusion injury (IRI) worsens the duration and severity of vessel occlusion secondary to TCS, amplifying the ramifications of TCS on fertility (2). The pathophysiology, etiology, evaluation, and management of TCS are reviewed.
Pathophysiology
In the preliminary stages of TCS, the onset of ischemia causes hypoxia within the endothelium and surrounding tissues of the testicle, resulting in endothelial dysfunction by increasing cell volume, neutrophil and platelet adhesion, oxidant release, as well as by decreasing membrane fluidity (3-6). After recruitment through proinflammatory cytokines, neutrophil and platelet adhesion is accomplished by increased expression of cell adhesion molecules in the vascular endothelium as well as integrins on neutrophils. The neutrophils infiltrate the testicular interstitium using endothelial migration proteins (i.e., selectins) and release reactive oxygen species (ROS). They also cause swelling via local inflammatory responses (6). Such cell surface proteins that direct immune traffic to tissues are appropriately named addressins.
Ironically, the initial ischemia-induced changes are exacerbated in the period following reperfusion, a phenomenon known as IRI. The increased tissue deterioration from IRI is due to the increased production of ROS, e.g., superoxide and hydrogen peroxide. The ROS not only oxidize proteins and DNA but also cause phospholipid peroxidation, leading to membrane destruction and the ensuing release of autolytic enzymes (6). The oxidized products alone are highly reactive, generating a cascade that culminates in necrosis. Testicular tissue is particularly susceptible to ROS damage due to its inherently high rates of metabolism and cellular respiration (7).
There are two sources of oxidant release following IRI: endothelium and leukocytes. In the endothelium, hypoxia transforms xanthine dehydrogenase into xanthine oxidase, leading hypoxanthine (i.e., the substrate of xanthine dehydrogenase) to accumulate. The elevated oxygen levels upon reperfusion enable xanthine oxidase to convert the stores of hypoxanthine into urate, releasing superoxides and hydrogen peroxides as byproducts (3-6). Thus, endothelial oxidant release depends on the stores of hypoxanthine that accumulate during hypoxia. Hypoxia can negatively impact Leydig cell function and spermatogenesis, leading to male subfertility or infertility (8,9).
As the second source of oxidants, leukocytes account for the bulk of oxidant release after the initial endothelial response to reperfusion using the enzyme NADPH oxidase, a plasma membrane protein that forms superoxides while oxidizing NADPH to resume respiration after reperfusion (4,6). The effects of oxidants in reperfusion injury often persist for hours, as the oxidants promote gene transcription of adhesion proteins, e.g., E-selectin and intercellular adhesion molecule (ICAM)-1, which in turn attract more oxidant-releasing neutrophils, extending the half-life of IRI.
The increased release of oxygen radicals during reperfusion also disrupts nitric oxide (NO) levels in the vasculature. Normally, a minimal quantity of NO is sufficient to clear the serum of oxidants, however the post-reperfusion oxidant surge impedes other native functions of NO, such as vasodilation and preventing leukocyte and platelet adhesion (4). Furthermore, NO reacts with superoxide to form peroxynitrite, which participates in a negative feedback loop of NO synthase, perpetuating the decrease in NO levels (6).
This reperfusion-induced cascade worsens and prolongs the initial endothelial dysfunction. The transient compartment syndrome initially displayed by the vasculature begins to worsen as oxygenated blood reperfuses temporarily hypoxic regions of the endothelium. While the arterioles vasoconstrict due to NO deficiency, the capillaries are easily constricted due to the increased extraluminal pressure from edema and decreased intraluminal pressure from platelet and leukocyte adhesion (4,6). The venules face the largest amounts of blood cell adhesion and ensuing edema due to increased transcription of P-selectin and NF-κB, which mobilize selectins and other proteins involved in the inflammatory response (6). Since these changes in gene transcription can outlast the ischemia, even mild TCS can be harmful to tissues, particularly those involved in spermatogenesis. Damage to the Leydig cell compartment can impair fertility as it is closely associated with the severity of spermatogenic impairment (10).
IRI can cause partially occlusive events to become acute and potentially plays a major role in the progression of TCS. For instance, transient compression of the microcirculation by orchitis can be exacerbated by IRI to complete capillary constriction, resulting in TCS. In addition to this, IRI may also complicate the management of TCS, as the restoration of microcirculation inevitably involves some degree of IRI. The concept of IRI is critical to understanding the pathogenesis of TCS illustrated in Figure 1.
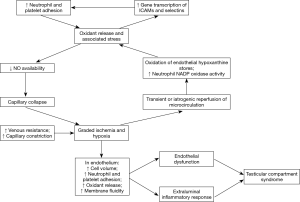
Etiology
There are two basic mechanisms that result in TCS: increased venous resistance and extraluminal compression of the microvasculature (1). Increasing venous resistance out of the scrotum increases the hydrostatic pressure in the venules and capillaries, in turn increasing the water filtration into the interstitium. TCS occurs when the increasing interstitial pressure results in capillary constriction. The second mechanism causes TCS by directly constricting the capillaries.
Table 1 lists TCS etiologies with respect to increased venous resistance. Varicoceles are associated with higher hydrostatic pressure and incompetent valves, causing stagnation of capillary flow—leading to ischemia—and increased fluid filtration into the interstitium (11-14). While subclinical varicocele may not pose a risk for TCS, clinical varicocele is documented to increase the pulsatility and resistive indices of testicular arteries, both of which decrease after varicocelectomy (15-17). The fact that varicoceles increase oxidant release in the testicle supports the theory that the infertility associated with varicoceles may be a result of secondary TCS (18-20). Arteriovenous fistulas can also theoretically lead to TCS, since arterial flow directly into the veins can cause backflow, increasing venous resistance. Testicular torsion can result in TCS by causing the spermatic cord veins to twist, which decreases vessel diameter and increases venous resistance (1,21). Even partial torsion poses a risk for TCS as it is documented to reverse diastolic flow and increase the arterial resistive index of the affected testicle (22,23). A hernia compressing the spermatic cord at the inguinal rings can obstruct the venous outflow of the testicles, leading to TCS. In severe cases, a large descending inguinoscrotal hernia can compress the tunica vaginalis enough to cause TCS via the second mechanism previously described.
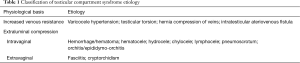
Full table
The second mechanism of TCS onset can be further classified based on whether the pressure is internal or external to the parietal tunica vaginalis. If the pressure is intravaginal, then the low compliance of the tunica albuginea maintains the pressure on the testicle, making constriction, and thus TCS more likely. On the other hand, extravaginal pressure must be severe enough to cause TCS; the fascial and dermal layers in the scrotum can expand, preventing much of the pressure from being translated to the testicle. Compression-related etiologies of TCS are grouped in Table 1. Orchitis or epididymo-orchitis may cause severe TCS as it is documented to cause testicular infarction by increasing the testicular resistive index (24-26). Hemorrhage or hematoma may be particularly likely to cause TCS if the patient is taking anticoagulant medication. Hydrocele can cause TCS by altering the shape of the testicle through compression and increasing the pulsatility and resistive indices (27,28). Mihmanli et al. noted that these parameters decrease post-hydrocelectomy (29). Though hydrocele has been found to increase interstitial and tunical fibrosis, it is the fluid pressure and tension in the tunica vaginalis that has been linked to halted spermatogenesis (30). Resultant testicular atrophy may stem from interstitial fibrosis as well as the fluid pressure-based obstruction of the vas or epididymis which prompts disorganization and sloughing (30). Edema within the tight fibrous sheath surrounding the tunica vaginalis may be responsible for the pressure on the testicular blood supply vital to spermatogenesis (30). Theoretically, severe pneumoscrotum may cause TCS by compressing the testicle (31). Compression from the inguinal canal of an undescended testicle may result in TCS, indicated by smaller testicular size and higher instance of vascular abnormalities in cryptorchidism (32). The instance of infertility is higher for many of these etiologies, such as hydrocele and cryptorchidism, with suspected underlying oxidant stres (18). The IRI from TCS secondary to these etiologies may explain the oxidant stress and associated infertility (33,34).
Evaluation
The key diagnostic tool for TCS is the index of suspicion; if the patient presents with one or more of the etiologies in Table 1, TCS should be suspected. If acute scrotum is presented, ultrasonography (US) must be performed as soon as possible to detect and prevent testicular injury. As detailed by Schurich et al., US offers two modalities to evaluate perfusion of testicular microcirculation: structural or functional analysis (35). The former consists of testicular volume measurements by grayscale US, which can indicate prolonged ischemia secondary to TCS if an unusually small testis is observed. Real-time elastography also falls in this category and measures the elasticity of tissue, which can indicate TCS, as the inflammatory response stiffens tissue (35). Functional analysis aims to directly measure the macro and microcirculations of the testicle. Unenhanced Doppler US—including the color, power, and spectral variations—is only sensitive enough to view macrocirculatory discrepancies, indicating TCS only if the capillary block is severe enough to decrease arterial flow. Upon initial duplex US scan, we recommend injection of prostaglandin (20 mg) into the spermatic cord for a secondary scan to adequately review the status of the compressed testicle and circulation for TCS. Power Doppler US is the most sensitive to low flow states, so it may hold the most potential for TCS diagnosis (35,36). Pulse wave US assesses perfusion by measuring the resistive index of testicular vessels—typically the testicular and intratesticular branches—where a higher resistance corresponds to impaired flow. However, the preceding methods can detect only the severe presentations of TCS, in which case testicle recovery may be unfeasible. Contrast-enhanced US depicts small-vessel perfusion by releasing contrast into the testicular venous system, thus allowing for earlier detection of TCS. However, most medical centers do not routinely use contrast for the direct visualization of TCS due to the high costs of testing and possible complications. Developing technologies in this field, e.g., microvascular imaging and Cadence™ contrast pulse sequencing, promise to improve diagnostic integrity of microcirculatory perfusion (35).
A proven chemical test for TCS would be a diagnostic breakthrough; current candidates for chemical markers consist of the byproducts of oxidative stress, such as malondialdehyde, 13-hydroperoxy-9,11-octadeconoic acid, volatile hydrocarbons, and isoprostanes (6). Measuring tissue oxygen saturation using near-infrared spectroscopy has been shown to reliably detect chronic compartment syndrome in the leg, but has yet not been used to evaluate TCS (37). If applicable, near-infrared spectroscopy of the testicle could measure the severity of TCS and determine when surgical exploration is necessary (38).
When acute TCS is highly suspected, due to disproportionate pain, diagnosis must be confirmed by exploration of the scrotum. Kutikov et al. demonstrated that a pressure needle through the tunica albuginea could provide an accurate indication of TCS, with 30 mmHg being the threshold for “imminent” compartment syndrome (1,39). Kutikov et al. also noted that significant TCS gives the testicle a dull purple appearance; its bright red color is restored upon relieving the pressure (1). If TCS is suspected in subacute cases, we suggest that the testicle should be periodically monitored with power Doppler US. The other unenhanced US techniques can only determine if testicular blood flow is absent, in which case the testicle is unlikely to be viable (40). However, power Doppler measurements are “symmetrical” with respect to the two testes, allowing a testicle suspected of TCS to be compared to the unaffected testicle to determine if blood flow is in fact diminished (36). Though further testing is necessary, periodic power Doppler US monitoring has the potential to give an earlier indication of TCS while avoiding unnecessary puncture of the testicle to obtain pressure readings.
Management
Since TCS can lead to defective testicular endocrine function and spermatogenesis, the condition must be taken seriously and managed with prompt care. Treatment options should be factored on the etiology and severity of clinical presentation. When compartment pressure is low, significant infarction is unlikely and the underlying etiology should be treated, followed by pulsed wave Doppler US perfusion evaluations to confirm diminishing TCS (28). We suggest periodic power Doppler US in case TCS recurs. In presentations of recurrent or acute TCS where IRI is responsible for increased intratesticular pressure, Kutikov et al. demonstrated that incising (i.e., testicular capsulotomy) and then enlarging the tunica albuginea with a tunica vaginalis graft returns compartment pressure to normal ranges (1). Figueroa et al. was one of the first to test this technique via retrospective clinical study and indeed found that it enhances testicular salvageability after prolonged ischemia, offsetting the need for orchiectomy (41). Alternately, albeit in a rat model, Józsa et al. found that decompressed testes did not have any significant effect in testicular microcirculation, reduction in testicular volume, changes in hemorheological parameters, and germinal epithelial necrosis (42). Despite holding potential in immediately addressing TCS, more studies such as those testing spermatogenesis are warranted to establish it as a treatment standard.
IRI also has to be addressed since it can potentially counteract the treatment. Antioxidant administration can, in principle, prevent IRI in three ways: inhibit oxidant release, scavenge released oxidants, and rectify oxidant damage. Antioxidant therapy should be given before surgical intervention to prevent IRI. The common enzymatic and non-enzymatic antioxidants used for infertility include superoxide dismutase, thioredoxin reductase, gluthione reductase, vitamin E, vitamin C, allopurinol, and melatonin (6,43,44). Although the clinical use of these antioxidants has seen mixed success in minimizing IRI and restoring fertility, their use is not known to be detrimental. Hence, prior to reperfusing the testicle, administering antioxidants in standard doses may be prudent (45-47). Recently in animal models, ghrelin has demonstrated not only increased antioxidant enzyme activity and decreased lipid peroxidation of testicular tissue exposed to IRI (48), but also protection against degeneration by increasing seminiferous tubule diameter and thickness of the germinal epithelium (7). Also in animal models, Al-Maghrebi et al. discovered that exogenous angiotensin-(1-7) administration before IRI rescued testicular angiotensin-converting enzyme and Mas expression, protecting against germ cell apoptosis and oxidative stress; increased NO and activation of the PI3K/Akt signaling pathway may be potentially responsible (49). The same investigator recently found that the antioxidant genistein alleviates testicular IRI-induced spermatogenic damage and oxidative stress by suppressing the aberrant testicular matrix metalloproteinase system via the Notch 2/Jagged 1/Hes-1 and caspase-8 pathways (50). Al-Maghrebi et al. also observed that lutein treatment may prevent testicular IRI spermatogenic damage by interfering with its modulation of the survivor activating factor enhancement pathway (51). Pterostillbene (52), dexmedetomidine (53), curcumin (54), apocynin (55), 2-APB (56), interleukin-10 (57), tyrphostin AG 556 (58), desferrioxamine (59), and hyperbaric oxygen therapy (60) have all shown protective effect against testicular IRI at some capacity. Another possible novel therapeutic target for minimizing IRI that warrants further study is chemical histone deacetylase (HDAC) inhibitors, as Hou et al. found ischemia-induced HDAC activity in the testis with reduced histone acetyl transferase activity (61).
Conclusions
TCS is the impairment of microcirculation in the testicle and has ramifications on tissue integrity and spermatogenesis through the oxidant-producing mechanisms of IRI. In theory, several etiologies are and should be able to induce TCS, potentially explaining the association of these etiologies with infertility and overall testicular dysfunction. Current imaging technologies allow TCS to be detected only when it has progressed to impair the larger vessels of the testicle, but future imaging promises to advance microcirculatory visualization and tissue oxygenation evaluation. Periodically monitoring suspected TCS with power Doppler US may allow for earlier detection by comparing to the contralateral testis. Upon diagnosis, TCS should be evaluated for severity and then treated accordingly. Antioxidants may be used to minimize IRI and can only help, not hurt, in treatment. Because testicular oxidant stress occurs in up to 50% of infertility cases and since TCS has many mechanisms to cause such an increased oxidant load, it seems logical that TCS may be a larger contributor to infertility than currently established (45). Additional studies are needed to substantiate the role of TCS in mechanisms for testicular dysfunction.
Acknowledgements
The authors are thankful to Drs. Todd Miller, Kelly Warren, and Peter Brink for departmental support, as well as Mrs. Wendy Isser and Ms. Grace Garey for literature retrieval.
Footnote
Conflicts of Interest: The authors have no conflicts of interest to declare.
References
- Kutikov A, Casale P, White MA, et al. Testicular compartment syndrome: a new approach to conceptualizing and managing testicular torsion. Urology 2008;72:786-9. [Crossref] [PubMed]
- Karaguzel E, Kadihasanoglu M, Kutlu O. Mechanisms of testicular torsion and potential protective agents. Nat Rev Urol 2014;11:391-9. [Crossref] [PubMed]
- Sirotković-Skerlev M, Plestina S, Bilić I, et al. Pathophysiology of ischaemia-reperfusion injury. Lijec Vjesn 2006;128:87-95. [PubMed]
- Carden DL, Granger DN. Pathophysiology of ischaemia-reperfusion injury. J Pathol 2000;190:255-66. [Crossref] [PubMed]
- Welbourn CR, Goldman G, Paterson IS, et al. Pathophysiology of ischaemia reperfusion injury: central role of the neutrophil. Br J Surg 1991;78:651-5. [Crossref] [PubMed]
- Girn HR, Ahilathirunayagam S, Mavor AI, et al. Reperfusion syndrome: cellular mechanisms of microvascular dysfunction and potential therapeutic strategies. Vasc Endovascular Surg 2007;41:277-93. [Crossref] [PubMed]
- Taati M, Moghadasi M, Dezfoulian O, et al. Effects of Ghrelin on Testicular Ischemia/Reperfusion-Induced Injury. Acta Med Iran 2016;54:32-8. [PubMed]
- Reyes JG, Farias JG, Henríquez-Olavarrieta S, et al. The hypoxic testicle: physiology and pathophysiology. Oxid Med Cell Longev 2012;2012:929285.
- Madrid E, Reyes JG, Hernández B, et al. Effect of normobaric hypoxia on the testis in a murine model. Andrologia 2013;45:332-8. [Crossref] [PubMed]
- Lardone MC, Piottante A, Valdevenito R, et al. Histological and hormonal testicular function in oligo/azoospermic infertile men. Andrologia 2013;45:379-85. [Crossref] [PubMed]
- Salama N, Bergh A, Damber JE. The changes in testicular vascular permeability during progression of the experimental varicocele. Eur Urol 2003;43:84-91. [Crossref] [PubMed]
- Gat Y, Gornish M, Navon U, et al. Right varicocele and hypoxia, crucial factors in male infertility: fluid mechanics analysis of the impaired testicular drainage system. Reprod Biomed Online 2006;13:510-5. [Crossref] [PubMed]
- Gat Y, Zukerman Z, Chakraborty J, et al. Varicocele, hypoxia and male infertility. Fluid Mechanics analysis of the impaired testicular venous drainage system. Hum Reprod 2005;20:2614-9. [Crossref] [PubMed]
- Braedel HU, Steffens J, Ziegler M, et al. A possible ontogenic etiology for idiopathic left varicocele. J Urol 1994;151:62-6. [PubMed]
- Akcar N, Turgut M, Adapinar B, et al. Intratesticular arterial resistance and testicular volume in infertile men with subclinical varicocele. J Clin Ultrasound 2004;32:389-93. [Crossref] [PubMed]
- Unsal A, Turgut AT, Taşkin F, et al. Resistance and pulsatility index increase in capsular branches of testicular artery: indicator of impaired testicular microcirculation in varicocele? J Clin Ultrasound 2007;35:191-5. [Crossref] [PubMed]
- Balci A, Karazincir S, Gorur S, et al. Long-term effect of varicocele repair on intratesticular arterial resistance index. J Clin Ultrasound 2008;36:148-52. [Crossref] [PubMed]
- Turner TT, Lysiak JJ. Oxidative stress: a common factor in testicular dysfunction. J Androl 2008;29:488-98. [Crossref] [PubMed]
- Mohammed A, Chinegwundoh F. Testicular varicocele: an overview. Urol Int 2009;82:373-9. [Crossref] [PubMed]
- Agarwal A, Hamada A, Esteves SC. Insight into oxidative stress in varicocele-associated male infertility: part 1. Nat Rev Urol 2012;9:678-90. [Crossref] [PubMed]
- Kolbe A, Sun CC, Hill JL. Unpredictability of capsulotomy in testicular torsion. J Pediatr Surg 1987;22:1105-9. [Crossref] [PubMed]
- Cassar S, Bhatt S, Paltiel HJ, et al. Role of spectral Doppler sonography in the evaluation of partial testicular torsion. J Ultrasound Med 2008;27:1629-38. [PubMed]
- Prando D. Torsion of the spermatic cord: the main gray-scale and doppler sonographic signs. Abdom Imaging 2009;34:648-61. [Crossref] [PubMed]
- Lefort C, Thoumas D, Badachi Y, et al. Ischemic orchiditis: review of 5 cases diagnosed by color Doppler ultrasonography. J Radiol 2001;82:839-42. [PubMed]
- Moore JB, Hasenboehler EA. Orchiectomy as a result of ischemic orchitis after laparoscopic inguinal hernia repair: case report of a rare complication. Patient Saf Surg 2007;1:3. [Crossref] [PubMed]
- López Milena G, Medina Benítez A, Ortega Herrera R, et al. Usefulness of Doppler-color ultrasonography and identification of resistance indexes as early indicators of testicular infarction secondary to orchiepididymitis. Actas Urol Esp 2000;24:43-7. [PubMed]
- Turgut AT, Unsal A, Ozden E, et al. Unilateral idiopathic hydrocele has a substantial effect on the ipsilateral testicular geometry and resistivity indices. J Ultrasound Med 2006;25:837-43. [PubMed]
- Douglas JW, Hicks JA, Manners J, et al. A pressing diagnosis - a compromised testicle secondary to compartment syndrome. Ann R Coll Surg Engl 2008;90:W6-8. [Crossref] [PubMed]
- Mihmanli I, Kantarci F, Kulaksizoglu H, et al. Testicular size and vascular resistance before and after hydrocelectomy. AJR Am J Roentgenol 2004;183:1379-85. [Crossref] [PubMed]
- Dandapat MC, Padhi NC, Patra AP. Effect of hydrocele on testis and spermatogenesis. Br J Surg 1990;77:1293-4. [Crossref] [PubMed]
- Watson HS, Klugo RC, Coffield KS. Pneumoscrotum: report of two cases and review of mechanisms of its development. Urology 1992;40:517-21. [Crossref] [PubMed]
- Weiss AP, Van Heukelom J. Torsion of an undescended testis located in the inguinal canal. J Emerg Med 2012;42:538-9. [Crossref] [PubMed]
- Makker K, Agarwal A, Sharma R. Oxidative stress & male infertility. Indian J Med Res 2009;129:357-67. [PubMed]
- Agarwal A, Makker K, Sharma R. Clinical relevance of oxidative stress in male factor infertility: an update. Am J Reprod Immunol 2008;59:2-11. [Crossref] [PubMed]
- Schurich M, Aigner F, Frauscher F, et al. The role of ultrasound in assessment of male fertility. Eur J Obstet Gynecol Reprod Biol 2009;144 Suppl 1:S192-8. [Crossref] [PubMed]
- Barth RA, Shortliffe LD. Normal pediatric testis: comparison of power Doppler and color Doppler US in the detection of blood flow. Radiology 1997;204:389-93. [Crossref] [PubMed]
- van den Brand JG, Nelson T, Verleisdonk EJ, et al. The diagnostic value of intracompartmental pressure measurement, magnetic resonance imaging, and near-infrared spectroscopy in chronic exertional compartment syndrome: a prospective study in 50 patients. Am J Sports Med 2005;33:699-704. [Crossref] [PubMed]
- Seekamp A, Blankenburg H, van Griensven M, et al. Intramuscular pO2 monitoring in compartment syndrome--an experimental study. Zentralbl Chir 1998;123:285-91; discussion 291-2. [PubMed]
- Scola E. Pathophysiology and pressure measurement in compartment syndrome. Unfallchirurg 1991;94:220-4. [PubMed]
- Kaye JD, Shapiro EY, Levitt SB, et al. Parenchymal echo texture predicts testicular salvage after torsion: potential impact on the need for emergent exploration. J Urol 2008;180:1733-6. [Crossref] [PubMed]
- Figueroa V, Pippi Salle JL, Braga LH, et al. Comparative analysis of detorsion alone versus detorsion and tunica albuginea decompression (fasciotomy) with tunica vaginalis flap coverage in the surgical management of prolonged testicular ischemia. J Urol 2012;188:1417-22. [Crossref] [PubMed]
- Józsa T, Klárik Z, Kiss F, et al. Morphological and microcirculatory evaluation of the rat testis after detorsion with or without a capsular release with a tunica vaginalis flap. Asian J Androl 2016;18:462-6. [Crossref] [PubMed]
- Sukhotnik I, Meyer G, Nativ O, et al. Effect of allopurinol on germ cell apoptosis following testicular ischemia-reperfusion injury in a rat. Pediatr Surg Int 2008;24:61-6. [Crossref] [PubMed]
- Reiter RJ, Tan DX, Jou MJ, et al. Biogenic amines in the reduction of oxidative stress: melatonin and its metabolites. Neuro Endocrinol Lett 2008;29:391-8. [PubMed]
- Tunc O, Thompson J, Tremellen K. Improvement in sperm DNA quality using an oral antioxidant therapy. Reprod Biomed Online 2009;18:761-8. [PubMed]
- Patel SR, Sigman M. Antioxidant therapy in male infertility. Urol Clin North Am 2008;35:319-30. x. [Crossref] [PubMed]
- Shen L, Ji HF, Zhang HY. How to understand the dichotomy of antioxidants. Biochem Biophys Res Commun 2007;362:543-5. [Crossref] [PubMed]
- Taati M, Moghadasi M, Dezfoulian O, et al. The effect of ghrelin pretreatment on epididymal sperm quality and tissue antioxidant enzyme activities after testicular ischemia/reperfusion in rats. J Physiol Biochem 2012;68:91-7. [Crossref] [PubMed]
- Al-Maghrebi M, Renno WM. The tACE/Angiotensin (1-7)/Mas Axis Protects Against Testicular Ischemia Reperfusion Injury. Urology 2016;94:312.e1-8. [Crossref] [PubMed]
- Al-Maghrebi M, Renno WM. Genistein alleviates testicular ischemia and reperfusion injury-induced spermatogenic damage and oxidative stress by suppressing abnormal testicular matrix metalloproteinase system via the Notch 2/Jagged 1/Hes-1 and caspase-8 pathways. J Physiol Pharmacol 2016;67:129-37. [PubMed]
- Al-Maghrebi M, Renno WM, Al-Somali HF, et al. Lutein modulates transcription dysregulation of adhesion molecules and spermatogenesis transcription factors induced by testicular ischemia reperfusion injury: it could be SAFE. Naunyn Schmiedebergs Arch Pharmacol 2016;389:539-51. [Crossref] [PubMed]
- Kim HJ, Lee JW, Hwang BR, et al. Protective effect of pterostilbene on testicular ischemia/reperfusion injury in rats. J Pediatr Surg 2016;51:1192-6. [Crossref] [PubMed]
- Tuglu D, Yuvanc E, Yilmaz E, et al. The antioxidant effect of dexmedetomidine on testicular ischemia-reperfusion injury. Acta Cir Bras 2015;30:414-21. [PubMed]
- Takhtfooladi MA, Asghari A, Takhtfooladi HA, et al. The protective role of curcumin on testicular tissue after hindlimb ischemia reperfusion in rats. Int Urol Nephrol 2015;47:1605-10. [Crossref] [PubMed]
- Şener TE, Yüksel M, Özyılmaz-Yay N, et al. Apocynin attenuates testicular ischemia-reperfusion injury in rats. J Pediatr Surg 2015;50:1382-7. [Crossref] [PubMed]
- Sari E, Aksit H, Erken HA, et al. Protective effect of 2-APB on testicular ischemia-reperfusion injury in rats. J Urol 2015;193:1036-41. [Crossref] [PubMed]
- Ozturk H, Ozturk H, Terzi EH, et al. Interleukin 10 reduces testicular damage in experimental testicular ischemia/reperfusion injury. Urology 2014;83:508.e1-6. [Crossref] [PubMed]
- Karaguzel E, Sivrikaya A, Mentese A, et al. Investigation of tyrphostin AG 556 for testicular torsion-induced ischemia reperfusion injury in rat. J Pediatr Urol 2014;10:223-9. [Crossref] [PubMed]
- Ayvaz S, Inan M, Aksu B, et al. Desferrioxamine effectively attenuates testicular tissue at the end of 3 h of ischemia but not in an equal period of reperfusion. J Pediatr Urol 2014;10:550-8. [Crossref] [PubMed]
- Zhang Y, Lv Y, Liu YJ, et al. Hyperbaric oxygen therapy in rats attenuates ischemia-reperfusion testicular injury through blockade of oxidative stress, suppression of inflammation, and reduction of nitric oxide formation. Urology 2013;82:489.e9-489.e15. [Crossref] [PubMed]
- Hou W, Dong Y, Zhang J, et al. Hypoxia-induced deacetylation is required for tetraploid differentiation in response to testicular ischemia-reperfusion (IR) injury. J Androl 2012;33:1379-86. [Crossref] [PubMed]