External pressure induces the dysfunction of spermatogonia via triggering the intrinsic pathway of apoptosis
Highlight box
Key findings
• In this experiment, we established a clear link between heightened external pressure and apoptosis of spermatogenic cells.
What is known and what is new?
• Cryptorchidism is a known factor contributing to male infertility. In clinical practice, undescended testes that are surgically removed typically exhibit characteristics such as reduced volume, soft texture, or underdevelopment. In cases where the testicles remain undescended, the external pressure exceeds that within the scrotum.
• The escalation of external pressure leads to intrinsic apoptosis of spermatogenic cells.
What are the implications, and what needs to change?
• The surge in exogenous pressure triggering spermatogenic cell apoptosis offers a fresh perspective for investigating the mechanisms behind male infertility associated with cryptorchidism.
Introduction
During the process of reproductive development, cryptorchidism refers to the failure of the testes to descend into the scrotum, including incomplete descent and ectopic testes (1). The incidence of this condition in full-term male infants is approximately 1% to 4.6% (2-4). There are various factors associated with cryptorchidism and male infertility, including genetic variations, oxidative stress, production of anti-sperm antibodies, increased apoptosis, the influence of the genitofemoral nerve, and hormonal imbalances (5,6). The production of anti-sperm antibodies may trigger an autoimmune reaction, leading to infertility (7). Furthermore, damage to the genitofemoral nerve leads to an increase in calcitonin gene-related peptide released by the genitofemoral nerve and its terminals (8), as well as a decrease in testosterone, which cause changes in testicular blood flow and local temperature, resulting in testicular damage and infertility (9). In addition, increased cell apoptosis is considered one of the key factors leading to a decrease in sperm count (6). Genetic variations affect spermatogonia apoptosis through multiple pathways and oxidative stress and high levels of nitric oxide generate excessive reactive oxygen species, potentially leading to an increase in testicular germ cell apoptosis, while high temperature has been shown to induce spermatogonia apoptosis (10-12).
In clinical practice, undescended testes that are surgically removed generally have a smaller volume, soft texture, or are underdeveloped. Conventional wisdom holds that many factors, including hormonal balance, age, health status, stress levels, lifestyle choices, environmental influences, genetic predispositions, and conditions like varicocele, can impact sperm production. Cryptorchidism can elevate testicular temperature, disrupt hormone equilibrium, and induce genetic alterations. Additionally, research indicates that excessive external pressure can alter sperm morphology, reduce motility, increase abnormal sperm production, cause sperm DNA damage, and impair male fertility in mice (13).
Research indicated that the pressure within the inguinal canal exceeds normal atmospheric pressure by approximately 28.15 cmH2O (14), while the pressure within the scrotum typically remains at atmospheric levels. Moreover, abdominal pressure plays a crucial role in facilitating testicular descent (15). Therefore, it is hypothesized that when the testes fail to descend smoothly into the scrotum and remain in the abdominal cavity and inguinal canal, the elevated pressure behind the peritoneum and in the inguinal canal may also contribute to germ cell apoptosis. This study aimed to evaluate the impact of external pressure on spermatogonia. We present this article in accordance with the MDAR reporting checklist (available at https://tau.amegroups.com/article/view/10.21037/tau-24-158/rc).
Methods
Materials
The spermatogonia (cell line GC-1) were purchased from Wanwu Biotechnology, Hefei, China. The cell pressure culture instrument was from the Naturethink Company, Shanghai, China. The CCK8 assay kit (BS350B, Bioharp Biotechnology, Hefei, China), phalloidin fluorescent dye (CA1610, Solaibao, Beijing, China), terminal deoxynucleotidyl transferase-mediated dUTP-biotin nick end labeling (TUNEL) assay kit (E-CK-A321, Elabscience Company, Wuhan, China), flow cytometry assay kit (AP101-100, Union-Biotech, Hangzhou, China), RNA extraction kit (220010, Feijie Biotechnology, Shanghai, China), Evo M-MLV reverse transcription kit (AG11707, Accurate Biology, Changsha, China), Evo M-MLV one-step reverse transcriptase quantitative polymerase chain reaction (RT-qPCR) kit (AG11732, Accurate Biology), Z-IETD-FMK (ab141382, Abcam, Cambridge, UK), SuperSignal™ West Atto Kit (A38555, Thermo Scientific, Waltham, MA, USA), Pierce™ enhanced chemiluminescence (ECL) western blot substrate (32209, Thermo Scientific), bicinchoninic acid protein assay kit (23227, Thermo Scientific), β-actin monoclonal antibody (66009, Proteintech, Wuhan, China), rabbit anti-bax (ab32503, Abcam), bcl-2 rabbit polyclonal antibody (A0208, Abclonal, Wuhan, China), caspase-3 rabbit polyclonal antibody (A16793, Abclonal), caspase-9 rabbit polyclonal antibody (A2636, Abclonal), and rabbit anti-survivin (sc-10811, Santa Cruz Biotechnology, Dallas, TX, USA) were purchased.
Cell culture and cell viability assay
The cells were cultured in a six-well plate in a constant temperature incubator at 37 ℃ with a 5% carbon dioxide concentration. The complete culture medium was prepared using Dulbecco’s modified Eagle medium, fetal bovine serum, and a mixture of penicillin-streptomycin, and the medium was changed every day. Cell passages were performed every 2 days. Once the cells adhered to the plate, they were incubated for 48 h. Cells exhibiting approximately 80% confluence and in robust condition were subjected to pressure treatment. In Karimi’s study, cells were exposed to various pressure gradients (0, 25, 50, and 100 mmHg) using a pressure incubator (13). Each pressure level was applied for 2 and 4 h to assess its impact on cellular responses. Our study draws upon this research, employing identical pressure gradients and exposure times to simulate cellular pressures. According to the instructions of the Cell Counting Kit-8 (CCK8) kit, the spermatogonia were prepared as a cell suspension, and approximately 2×103 cells were counted. After continued cultivation in a 96-well plate for 24 h, the cells were subjected to pressure stimulation by the gauge from Naturethink Company. Pressure-dependent viability assay was examined at various pressure gradients (0, 25, 50, and 100 mmHg) for 4 h. Time-dependent viability assay was assessed for different time durations (0, 2, and 4 h) with 50 mmHg. Finally, the absorbance of each well was measured at 450 nm.
Cell morphology
The cells were pretreated with 4% paraformaldehyde and 0.5% triton. Subsequently, the cells were incubated with phalloidin dye in the dark for 30 minutes. The nuclei were stained with 4',6-diamidino-2-phenylindole (DAPI) for 5 minutes and mounted with an anti-fade mounting reagent. The cells’ morphological changes were examined using the fluorescence microscope (ZEIZZ Axioscope 5, Oberkochen, Badenweg, Germany) at magnifications of 200× and 400×. Three high-power fields were assessed for each section, and each measurement was conducted in triplicate.
TUNEL assay
According to the instructions of the TUNEL assay kit, cells were treated with 4% paraformaldehyde and 0.5% triton in advance. After thorough washing, cells were incubated with TdT equilibrium buffer in the incubator at 37 ℃ for 30 minutes. Then, cells were incubated with labeled working solution containing TdT enzyme for 60 minutes at 37 ℃. DAPI staining was performed for 5 minutes. Finally, the results were observed under the fluorescence microscope (ZEIZZ Axioscope 5, GER) at magnifications of 100×. Three high-power fields were assessed for each section, and each measurement was conducted in triplicate. TUNEL positive cells were counted by Image J.
Flow cytometry
According to the flow cytometry instructions, approximately 3×105 cells were counted by the flow cytometer, and 5 µL of Annexin V-fluorescein isothiocyanate (FITC) and 10 µL of propidium iodide (PI) were added. The cells were incubated in the dark for 5 minutes and the analysis was performed by Agilent NovoCyte flow cytometer (Agilent Technologies, Palo Alto, CA, USA). The raw data were further analyzed with the use of FlowJo software (Tree Star Inc., Ashland, OR, USA). The proportion of apoptotic cells was the proportion of cells in the first and fourth quadrant of flow cytometry.
Transmission electron microscopy (TEM)
The cells under 50 mmHg for 0, 2, and 4 h were fixed with 3% glutaraldehyde and after thorough fixation, frozen cell blocks were cut into 60 nm ultrathin sections. The sections were stained with 2% uranyl acetate and lead citrate and then examined using a TEM system (TECNAI 10, Phillips, Eindhoven, The Netherlands) at an accelerating voltage of 80 kV.
Immunohistochemistry
The cells were pretreated with 4% paraformaldehyde and 0.5% triton and then blocked with normal goat serum at room temperature for 30 minutes. After blocking, the cells were incubated with primary antibodies for caspase-3 (dilution ratio 1:200) and caspase-9 (dilution ratio 1:200) at 4 ℃ for 16 h. Subsequently, the cells were exposed to mouse horseradish peroxidase at room temperature for 50 minutes and stained with 3,3'-diaminobenzidine (DAB) for 5 minutes. After restaining, bluing, and differentiation, the cells were dehydrated and sealed. The results were observed with the optical microscope (ZEIZZ Axioscope 5, GER) at magnifications of 100×. Three high-power fields were assessed for each section, and each measurement was conducted in triplicate.
Real-time qPCR
The total cellular RNA was extracted using an RNA extraction kit. According to the instructions, complementary DNA (cDNA) was obtained through reverse transcription using a cDNA reverse transcription kit.
The following primers were used for qPCR: bax forward, 5'-TCCACAAGCTGAGCGAG-3' and reverse, 5'-GTCAGCCATGATGGTTCT-3'; bcl-2 forward, 5'-GCGGGATATGGCCTTTTTG-3' and reverse, 5'-GCGGGATATGTTGACTTCA CTTG-3'; caspase-9 forward, 5'-GCGTTACGGCACAGATGCAT-3' and reverse, 5'-TGCTCGCGTTCTTGCGAGG-3'; caspase-3 forward, 5'-ATGACAACGAACTCCG TG-3' and reverse, 5'-CCACTCTCTCATTCCTTAGTG-3'; caspase-8 forward, 5'-TG CTTGGACTACATCCCACAC-3' and reverse, 5'-GTTGCAGTCTAGGAAGTTGA CC-3'; β-actin forward, 5'-GGCTGTATTCCCCTCCATCG-3' and reverse, 5'-CCAG TTGGTAACAATGCCATGT-3'. Real-time qPCR detection was performed using the QuantStudio™ 1 System (Thermo Scientific).
Western blot
Cellular proteins were extracted with radioimmunoprecipitation assay lysis buffer. The protein concentration was determined by the bicinchoninic acid kit. A total of 20 µg of proteins were isolated using polyacrylamide gel electrophoresis and transferred to a polyvinylidene fluoride (PVDF) membrane. The PVDF membrane was blocked with a blocking solution prepared from skim milk powder at room temperature for 2 h. Then the PVDF membrane was incubated with the antibody working solution at 4 ℃ overnight. After incubation, the sample was labeled with mouse horseradish peroxidase for 2 h at room temperature. Finally, the ECL kit was used for exposure of caspase-3, caspase-8, caspase-9, bax, bcl-2, and β-actin antibodies. The obtained images were analyzed using Image J software to determine the grayscale value of each protein band.
For the blocking experiment, Z-IETD-FMK (caspase-8 inhibitor, 20 µM) and methazolamide (cytochrome C inhibitor, 2.5 µM) were added to the complete cell culture medium and spermatogonia were subjected to pressure (50 mmHg) for 2 and 4 h. Then the cells were collected and the above western blot assay was repeated to detect the expressions of caspase-3 and caspase-9.
Statistical analysis
Each experiment was conducted in triplicate to ensure statistical significance. All data were analyzed using GraphPad software and represented as the mean ± standard deviation (SD) of continuous variables. Statistical differences between the two groups were analyzed using analysis one-way of variance. The Tukey Kramer test was used for post hoc comparison. The statistical significance was set to P<0.05. The apoptotic pathway diagram was drawn by Figraw software.
Results
Changes in the morphology of spermatogonia under external pressure
Immunofluorescence was used to observe the morphological changes of the cells. Under increasing pressure, the cell cytoskeleton became loose and the cell nuclei fragmented (Figure 1).
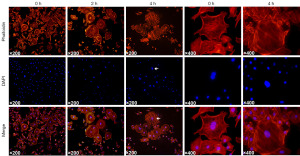
External pressure inhibited the activity of spermatogonia
The CCK8 experiment results (Figure 2A,2B) reflected the impact of external pressure on the activity of spermatogonia, showing a decrease in cell activity with increasing pressure gradients and time durations (P<0.05).
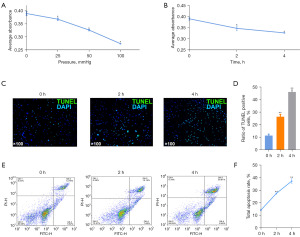
External pressure enhanced the apoptosis of spermatogonia
TUNEL assay and flow cytometry were used to identify the proportion of apoptotic cells. The results indicated that with the prolonged duration of external pressure, the rate of apoptotic cells significantly increased (P<0.01) (Figure 2C-2F).
Changes in the ultrastructure of spermatogonia under external pressure
TEM was used to observe the ultrastructure of the cells (Figure 3). Cells subjected to pressure showed changes in ultrastructure, with early apoptotic cells (2 h) exhibiting cell shrinkage, and chromatin condensation, while late apoptotic cells (4 h) showed nuclear condensation and fragmentation, cytoplasmic vacuolization, and the formation of apoptotic bodies, along with mitochondrial swelling and fusion of mitochondrial cristae.
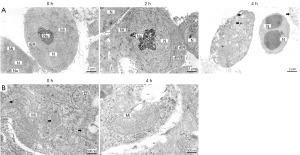
Modulation of apoptosis-related protein expressions under external pressure
Immunohistochemistry was used to observe the expressions of caspase-3 and caspase-9 (Figure 4A,4B). The proportion of cells expressing positive caspase-3 and caspase-9 increased with prolonged exposure to pressure (P<0.05). (Figure 4C) The qPCR showed significantly increased messenger RNA (mRNA) expressions of bax, caspase-3, and caspase-9 (P<0.05). The mRNA expression of bcl-2 was inhibited (P<0.05), with no remarkable effect on caspase-8 (P>0.05). Western blot analysis showed higher bax/bcl-2 ratio, as well as a higher expression levels of caspase-3 and caspase-9 with increasing time under pressure (50 mmHg) (P<0.05) (Figure 4D,4E). Cytochrome C inhibitor, methazolamide, blocked the activation of caspase-9 and caspase-3 (P>0.05) (Figure 4F). The caspase-8 inhibitor, Z-IETD-FMK, did not block the expression of caspase-3 (P<0.05) (Figure 4G).
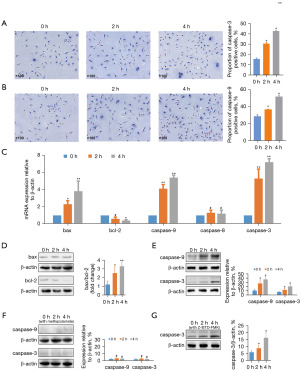
Discussion
Cryptorchidism is a congenital developmental abnormality in which one or both testes fail to descend into the scrotum during fetal development (16). It may be caused by factors such as gubernaculum issues, hormonal imbalances, and genetic predisposition (17). The incidence in full-term newborns is 1–4.6% and even higher in preterm infants (4). Cryptorchidism impacts testicular germ cell development, increasing the risk of infertility and malignant tumors (18).
Cryptorchidism is implicated in male infertility through a multitude of factors, including oxidative-reduction mechanisms, anti-sperm antibody production, increased cell apoptosis, genitofemoral nerve influence, and endocrine disturbances (2,19). Elevated levels of nitric oxide have been associated with an augmented rate of testicular germ cell apoptosis, ultimately leading to reduced male fertility (11,20). Furthermore, the presence of anti-sperm antibodies may contribute to infertility (21). Additionally, the elevated temperature environment associated with cryptorchidism not only directly triggers apoptosis of spermatogonia but also disrupts the blood-testis barrier, potentially allowing sperm leakage and immune cell infiltration, leading to an immune response and the production of anti-sperm antibodies, thus impacting fertility (20,22-24). The genitofemoral nerve and the release of calcitonin gene-related peptides from its terminals also impact male fertility by influencing testicular blood flow and temperature, ultimately causing testicular damage (7). Moreover, reduced serum testosterone levels may lead to germ cell DNA degradation and an increase in apoptotic cells, thereby affecting sperm production and quantity (9).
In clinical practice, surgically removed undescended testes typically exhibit reduced volume, a softer texture, or are underdeveloped. We hypothesize that the pressure in the retroperitoneum and inguinal canal affects testicular development and thus impacts sperm quality. A team from Razi University studied the effect of hydrostatic pressure on mouse sperm and the results indicated that excessive hydrostatic pressure reduced sperm motility, increased the rate of abnormal sperm, damaged sperm DNA, and thus reduced sperm fertilization ability (13). Spinal surgery research indicated that increased hydrostatic pressure of 30 atm induces apoptosis and decreases the viability of human nucleus pulposus cells by activating the Wnt/β-catenin pathway (25). In addition, higher external pressure can cause apoptosis of retinal ganglion cells (26,27). Therefore, elevated external pressure may be one of the causes of apoptosis in spermatogonia and this was further explored in this study.
In this study, we observed the inhibition of spermatogonia activity and changes in cell morphology under elevated pressure, as well as an increase in the rate of apoptosis of spermatogonia with increasing external pressure. There are mainly two classic pathways of apoptosis (Figure 5). The first is intrinsic apoptosis. Apoptotic stimuli trigger the opening of the non-selective channel mitochondrial permeability transition pore, causing mitochondrial swelling and increased membrane permeability, leading to cytochrome C release into the cytosol. Cytochrome C combines with Apaf-1 and pro-caspase-9 to form the apoptosome. Activated caspase-9 initiates apoptotic pathways and activates caspase-3, which cleaves substrate proteins, resulting in cellular apoptosis (28). The second is extrinsic apoptosis, in which death receptors bind to their ligands, such as Fas/FasL, leading to activation of caspase-8 and then caspase-3 to induce apoptosis (29). To further explore the pathways through which pressure-induced apoptosis in spermatogonia, TEM was used to observe mitochondrial swelling and vacuolization in apoptotic cells, suggesting the involvement of the mitochondrial pathway.
The activation and aggregation of bax protein lead to changes in mitochondrial membrane permeability and the release of apoptotic molecules from the mitochondria (30). The role of the bcl-2 protein is to inhibit the activity of the bax protein, preventing changes in mitochondrial membrane permeability and the occurrence of apoptosis (30,31). Therefore, changes in the ratio of bax/bcl-2 proteins in cells affect the sensitivity of apoptosis. This study found that pressure increased the expression of bax and decreased the expression of bcl-2, leading to an increased bax/bcl-2 ratio and an increased tendency for cell apoptosis (30,31). Caspase-3 is the execution of cell apoptosis and can be activated by intrinsic and extrinsic apoptotic pathways (30). The results of the study showed that pressure increased the expressions of caspase-9 and caspase-3, without remarkable effect on caspase-8. The cytochrome C inhibitor, methazolamide, effectively inhibited the activation of caspase-9 and inhibited the activation of caspase-3. Conversely, the caspase-8 inhibitor, Z-IETD-FMK, failed to demonstrate the same inhibitory effect on caspase-3. These findings suggested that external pressure may induce apoptosis of spermatogonia through the intrinsic apoptotic pathway.
This study only explored the effect of pressure on the intrinsic apoptotic pathway of spermatogonia in vitro and did not conduct in vivo experiments. We are developing animal models of cryptorchidism and examining the effects of external pressure on testicular tissue and spermatogenesis. This is an aspect of our research that we plan to improve in future studies.
Conclusions
External pressure induces apoptosis of spermatogonia through intrinsic apoptotic pathways, which may be one of the reasons for impaired sperm quality caused by cryptorchidism.
Acknowledgments
We thank the Naturethink Company, Shanghai for providing support for this study.
Funding: This research was funded by
Footnote
Reporting Checklist: The authors have completed the MDAR reporting checklist. Available at https://tau.amegroups.com/article/view/10.21037/tau-24-158/rc
Data Sharing Statement: Available at https://tau.amegroups.com/article/view/10.21037/tau-24-158/dss
Peer Review File: Available at https://tau.amegroups.com/article/view/10.21037/tau-24-158/prf
Conflicts of Interest: All authors have completed the ICMJE uniform disclosure form (available at https://tau.amegroups.com/article/view/10.21037/tau-24-158/coif). The authors have no conflicts of interest to declare.
Ethical Statement: The authors are accountable for all aspects of the work in ensuring that questions related to the accuracy or integrity of any part of the work are appropriately investigated and resolved.
Open Access Statement: This is an Open Access article distributed in accordance with the Creative Commons Attribution-NonCommercial-NoDerivs 4.0 International License (CC BY-NC-ND 4.0), which permits the non-commercial replication and distribution of the article with the strict proviso that no changes or edits are made and the original work is properly cited (including links to both the formal publication through the relevant DOI and the license). See: https://creativecommons.org/licenses/by-nc-nd/4.0/.
References
- Elamo HP, Virtanen HE, Toppari J. Genetics of cryptorchidism and testicular regression. Best Pract Res Clin Endocrinol Metab 2022;36:101619. [Crossref] [PubMed]
- Gurney JK, McGlynn KA, Stanley J, et al. Risk factors for cryptorchidism. Nat Rev Urol 2017;14:534-48. [Crossref] [PubMed]
- Kolon TF, Herndon CD, Baker LA, et al. Evaluation and treatment of cryptorchidism: AUA guideline. J Urol 2014;192:337-45. [Crossref] [PubMed]
- Batra NV, DeMarco RT, Bayne CE. A narrative review of the history and evidence-base for the timing of orchidopexy for cryptorchidism. J Pediatr Urol 2021;17:239-45. [Crossref] [PubMed]
- Sarila G, Hutson JM, Vikraman J. Testicular descent: A review of a complex, multistaged process to identify potential hidden causes of UDT. J Pediatr Surg 2022;57:479-87. [Crossref] [PubMed]
- Yuan JL, Zhang YT, Wang Y. Increased apoptosis of spermatogenic cells in cryptorchidism rat model and its correlation with transforming growth factor beta type II receptor. Urology 2010;75:992-8. [Crossref] [PubMed]
- Patel RP, Kolon TF, Huff DS, et al. Testicular microlithiasis and antisperm antibodies following testicular biopsy in boys with cryptorchidism. J Urol 2005;174:2008-10; discussion 2010. [Crossref] [PubMed]
- Zhu B, Liu Q, Lin LI, et al. Reductions in calcitonin gene-related peptide may be associated with the impairment of the contralateral testis in unilateral cryptorchidism. Exp Ther Med 2015;9:1797-800. [Crossref] [PubMed]
- Oreshkina EM, Bolotova NV, Pylaev TE, et al. Hormonal and genetic causes of cryptorchidism. Probl Endokrinol (Mosk) 2023;69:99-106. [Crossref] [PubMed]
- Sun W, Zhang X, Wang L, et al. RNA sequencing profiles reveals progressively reduced spermatogenesis with progression in adult cryptorchidism. Front Endocrinol (Lausanne) 2023;14:1271724. [Crossref] [PubMed]
- Kaltsas A. Oxidative Stress and Male Infertility: The Protective Role of Antioxidants. Medicina (Kaunas) 2023;59:1769. [Crossref] [PubMed]
- Wanta A, Noguchi K, Sugawara T, et al. Expression of Protein Markers in Spermatogenic and Supporting Sertoli Cells Affected by High Abdominal Temperature in Cryptorchidism Model Mice. J Histochem Cytochem 2023;71:387-408. [Crossref] [PubMed]
- Karimi N, Kamangar PB, Azadbakht M, et al. Effects of hydrostatic pressure on mouse sperm. Bratisl Lek Listy 2014;115:70-5. [Crossref] [PubMed]
- Zhou TC, Yang B, Zhang YC, et al. Study on intra-abdominal pressure in indirect inguinal hernia patients. Zhonghua Wai Ke Za Zhi 2007;45:1455-7. [PubMed]
- Logsdon NT, Sampaio FJB, Favorito LA. The role of intra-abdominal pressure in human testicular migration. Int Braz J Urol 2021;47:36-44. [Crossref] [PubMed]
- Lee PA, Houk CP. Cryptorchidism. Curr Opin Endocrinol Diabetes Obes 2013;20:210-6. [Crossref] [PubMed]
- Hadziselimovic F. On the descent of the epididymo-testicular unit, cryptorchidism, and prevention of infertility. Basic Clin Androl 2017;27:21. [Crossref] [PubMed]
- Nguyen V, Ngo L, Jaqua EE. Cryptorchidism (Undescended Testicle). Am Fam Physician 2023;108:378-85. [PubMed]
- Barthold JS, Reinhardt S, Thorup J. Genetic, Maternal, and Environmental Risk Factors for Cryptorchidism: An Update. Eur J Pediatr Surg 2016;26:399-408. [Crossref] [PubMed]
- Gao Y, Wang C, Wang K, et al. The effects and molecular mechanism of heat stress on spermatogenesis and the mitigation measures. Syst Biol Reprod Med 2022;68:331-47. [Crossref] [PubMed]
- Kurpisz M, Nakonechnyy A, Niepieklo-Miniewska W, et al. Weak association of anti-sperm antibodies and strong association of familial cryptorchidism/infertility with HLA-DRB1 polymorphisms in prepubertal Ukrainian boys. Reprod Biol Endocrinol 2011;9:129. [Crossref] [PubMed]
- Widlak W, Vydra N. The Role of Heat Shock Factors in Mammalian Spermatogenesis. Adv Anat Embryol Cell Biol 2017;222:45-65. [Crossref] [PubMed]
- Rocca MS, Di Nisio A, Sabovic I, et al. E2F1 copy number variations contribute to spermatogenic impairment and cryptorchidism by increasing susceptibility to heat stress. Andrology 2019;7:251-6. [Crossref] [PubMed]
- Zheng Y, Zhang P, Zhang C, et al. Surgery-induced cryptorchidism induces apoptosis and autophagy of spermatogenic cells in mice. Zygote 2019;27:101-10. [Crossref] [PubMed]
- Shi Z, He J, He J, et al. High hydrostatic pressure (30 atm) enhances the apoptosis and inhibits the proteoglycan synthesis and extracellular matrix level of human nucleus pulposus cells via promoting the Wnt/β-catenin pathway. Bioengineered 2022;13:3070-81. [Crossref] [PubMed]
- Vishwaraj CR, Kavitha S, Venkatesh R, et al. Neuroprotection in glaucoma. Indian J Ophthalmol 2022;70:380-5. [Crossref] [PubMed]
- Li Q, Cheng Y, Zhang S, et al. TRPV4-induced Müller cell gliosis and TNF-α elevation-mediated retinal ganglion cell apoptosis in glaucomatous rats via JAK2/STAT3/NF-κB pathway. J Neuroinflammation 2021;18:271. [Crossref] [PubMed]
- Abate M, Festa A, Falco M, et al. Mitochondria as playmakers of apoptosis, autophagy and senescence. Semin Cell Dev Biol 2020;98:139-53. [Crossref] [PubMed]
- D'Arcy MS. Cell death: a review of the major forms of apoptosis, necrosis and autophagy. Cell Biol Int 2019;43:582-92. [Crossref] [PubMed]
- Zhang Y, Yang X, Ge X, et al. Puerarin attenuates neurological deficits via Bcl-2/Bax/cleaved caspase-3 and Sirt3/SOD2 apoptotic pathways in subarachnoid hemorrhage mice. Biomed Pharmacother 2019;109:726-33. [Crossref] [PubMed]
- Mohan S, Abdelwahab SI, Kamalidehghan B, et al. Involvement of NF-κB and Bcl2/Bax signaling pathways in the apoptosis of MCF7 cells induced by a xanthone compound Pyranocycloartobiloxanthone A. Phytomedicine 2012;19:1007-15. [Crossref] [PubMed]