Association between cardiometabolic index and testosterone among adult males: a cross-sectional analysis from National Health and Nutrition Examination Survey 2013–2016
Highlight box
Key findings
• Cardiometabolic index (CMI) is negatively correlated with testosterone levels in adult males, as shown by the data from National Health and Nutrition Examination Survey 2013–2016.
What is known and what is new?
• It is known that testosterone decline and deficiency can affect men's health and may be linked to obesity and excessive visceral adipose tissue deposition.
• Higher CMI scores are associated with lower testosterone levels in adult males in the United States.
What is the implication, and what should change now?
• Clinicians should be aware of the potential use of CMI as a predictor for testosterone deficiency in adult males. Further research could be conducted to confirm the predictive value of CMI for testosterone levels and to explore the potential for CMI to be included in routine health assessments.
• Health policies might consider incorporating CMI measurements in standard physical exams for adult males to facilitate early detection and intervention for testosterone decline-related health issues.
Introduction
Testosterone exerts a multitude of beneficial physiological effects which is crucial for men’s well-being, assuming a pivotal role in the metabolism of carbohydrates, fats, and proteins (1). Moreover, testosterone considerably influences the preservation of skeletal and muscular mass, while also impacting body fat composition (2). Insufficiency of testosterone has been shown to be associated with increased adipose tissue, particularly central adiposity, reduced insulin sensitivity, impaired glucose tolerance, elevated levels of triglycerides (TGs) and total cholesterol (TC), and diminished high-density lipoprotein cholesterol (HDL-C) (3). Recent evidence suggests that testosterone levels may have declined in the USA and other industrialized countries, partly due to a rise in body mass index (BMI) and metabolic conditions. This decline in testosterone levels underscores the importance of investigating the relationship between testosterone, metabolism, and associated health outcomes. Understanding the factors contributing to changes in testosterone levels can provide valuable insights into the prevention and management of metabolic disorders in men (4). Clinical trials have demonstrated that testosterone replacement therapy ameliorates insulin resistance and improves glycemic control in hypogonadal men with type 2 diabetes, leading to reductions in body fat mass, primarily in truncal adiposity, cholesterol, and TGs (5,6). The mechanisms underlying testosterone’s impact on metabolic pathways remain unclear. However, an expanding body of evidence from studies involving animals, cells, and patients indicates that testosterone regulates the expression of crucial regulatory proteins involved in glycolysis, glycogen synthesis, and lipid and cholesterol metabolism at the molecular level (7,8).
The cardiometabolic index (CMI), calculated by multiplying TG (mmol/L)/High-density lipid-cholesterol (HDL-C) (mmol/L) × waist-to-height ratio (WHtR) (9), represents a novel metric for assessing visceral fat distribution and other adverse metabolic conditions (10). Early research has revealed its close association with patients afflicted by cardiovascular disease (11), kidney disease (12), erectile dysfunction (13), diabetes (14), and adverse metabolic conditions. Existing evidence suggests that CMI holds promise as a predictive tool for metabolic-related diseases (15), Testosterone levels, as a crucial male hormone influencing bodily metabolism, are speculated to be correlated with CMI. However, no previous study has examined the connection between CMI and testosterone. Hence, the aim of this study was to investigate the association between CMI and testosterone in adult males in the United States and evaluate the predictive value of CMI for testosterone levels. We present this article in accordance with the STROBE reporting checklist (available at https://tau.amegroups.com/article/view/10.21037/tau-24-121/rc) (16).
Methods
Study population
Cross-sectional data were obtained from National Health and Nutrition Examination Survey (NHANES), a national study to evaluate the nutrition and health status in the United States conducted by the National Center for Health Statistics (NCHS). The study was conducted in accordance with the Declaration of Helsinki (as revised in 2013). It was conducted with a complex multistage probability design to obtain a nationally representative group of non-institutionalized United States residents (17). The Research Ethics Review Board of the NCHS approved all NHANES study protocols, and written informed consent was obtained from all survey participants. All detailed NHANES study designs and data are publicly available at www.cdc.gov/nchs/nhanes/. This study selected 9,895 male participants from 2013 to 2016, and 2,209 people were determined according to the screening criteria to explore the relationship between CMI and testosterone. The exclusion criteria of this study were age <20 years old, lack of testosterone, height, waist, TG, and HDL-C indicators. The process of sample collection is displayed in Figure 1.
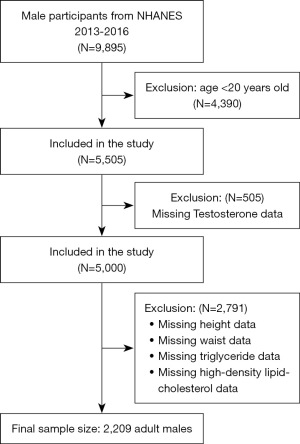
Exposure and outcome definitions
CMI served as an exposure variable and was calculated as TG (mmol/L)/HDL-C (mmol/L) × WHtR. Both TG and HDL-C measured through enzymatic assay, utilizing the Roche Modular P and Roche Cobas 6000 chemistry analyzers. Total testosterone was measured via isotope dilution liquid chromatography tandem mass spectrometry (ID-LC-MS/MS) based on the National Institute for Standards and Technology’s (NIST) reference method. Testosterone deficiency was defined as total testosterone <300 ng/dL according to American Urological Association guidelines (18).
Assessment of covariates
This study used a standard questionnaire to collect demographic data, including age, race, marital status, education level, family income to poverty ratio, smoking status, drinking status, and personal medical history (hypertension and diabetes). Smoking status was defined based on the self-report of Smoking-Cigarette Use questionnaire “smoked at least 100 cigarettes in life” (yes or no). Drinking status was determined based on average alcohol consumption in the past 12 months: ≤1 drink once was a non or a light drinker, 2–8 was a moderate drinker, and >8 was a heavy drinker. The body measures data, including BMI, standing height, and waist circumference, were collected at the Mobile Examination Center (MEC). The participants were divided into three groups based on BMI, normal or underweight: underweight: BMI ≤18.4 kg/m2, normal: 18.4< BMI ≤24.9 kg/m2, overweight: 24.9< BMI ≤30 kg/m2, and obese: BMI >30 kg/m2. Blood collection was performed in the morning after fasting to collect TC, high-density lipoprotein (HDL), low-density lipoprotein (LDL), TG, fasting blood glucose, insulin, and testosterone. Hypertension and diabetes were obtained from the self-report of a health questionnaire; the investigators asked the question “Ever told you by doctor have diabetes?” or “Ever told you by doctor have high blood pressure?”. An answer of “yes” indicates a “diabetes” case or “hypertension” case. Homeostatic Model Assessment of Insulin Resistance (HOMA-IR) index calculation: HOMA-IR = fasting plasma glucose (mmol/L) × fasting insulin (mU/mL)/22.5.
Statistical analysis
Continuous variables described by a normal distribution were presented as mean ± standard deviation (M ± SD). Non-normally distributed data were presented as median (interquartile range). Categorical variables were presented with numbers (n) and percentages (%). The Chi-squared test or Kruskal-Wallis H test was used for quartile groups of different CMIs. Regression model analysis assessed the association between CMI and testosterone, using β values and 95% confidence intervals (CIs) indicating the relationship. We applied Model 1 with adjusting none, Model 2 with adjusted age and race, and Model 3 with adjusted age, race, marital status, height, BMI, TG, high-density lipoprotein, hypertension, diabetes, and smoking status. We then used restricted cubic spline curves based on regression Model 3 to explore any non-linear relationship between CMI and testosterone. Further, stratified analyses were conducted according to age, race, marital status, education level, BMI status, smoking status, drinking status, and histories of chronic diseases. Their interactions were tested by log-likelihood ratio test. At last, the ability of the CMI index to predict testosterone deficiency was analyzed by the receiver operating characteristic (ROC) curve, and the area under the ROC curve (AUROC) was calculated. Z test was applied to test differences between AUROCs. We applied weighted analysis to explain the complex sampling of NHANES. Details can be found on this website (https://wwwn.cdc.gov/nchs/Thestatisticalsoftwareusedbynhanes/tutorials/module3.aspx). All analyses were performed using R version 4.3.2 (http://www.R-project.org, The R Foundation) and Empower software (www.empowerstats.com; X&Y Solutions, Inc., Boston, MA, USA). P<0.05 was considered as the statistically significant level.
Results
Population characteristics of participants
A total of 2,209 male participants were included in this study. These individuals were divided into four groups based on quartiles of CMI. The baseline characteristics of each group are shown in Table 1. The mean age of the participants was 49.7±17.3 years, with a mean CMI of 2.5±3.6 and an mean testosterone level of 456.4±196.2. Compared to participants with lower CMI values, those with higher CMI values exhibited elevated lower levels of testosterone, but higher BMI, TC, TC, fasting blood glucose and fasting insulin. They also had lower levels of HDL-C. Furthermore, this group showed a higher proportion of participants who were married or cohabiting, as well as an increased prevalence of hypertension and diabetes. In particular, individuals in the fourth quartile (Q4) showed the highest TG levels (248.37±180.09), HOMA-IR index values (7.53±16.43), and the lowest testosterone levels (383.91±181.18). Additionally, they had a higher likelihood of being obese (BMI 31.39±6.06) and experiencing complications such as hypertension (40.51%) and diabetes (17.9%). Significant differences were observed among the four groups for all variables, except for education level, family income to poverty ratio, and drinking status (all P<0.05).
Table 1
Characteristics | Q1 (N=552) | Q2 (N=552) | Q3 (N=552) | Q4 (N=553) | P value |
---|---|---|---|---|---|
Age (years) | 48.31±18.76 | 50.52±17.71 | 51.02±16.96 | 48.79±15.71 | 0.02 |
Race | <0.001 | ||||
Mexican American | 52 (9.42) | 77 (13.95) | 87 (15.76) | 104 (18.81) | |
Other Hispanic | 42 (7.61) | 53 (9.60) | 76 (13.77) | 69 (12.48) | |
Non-Hispanic White | 232 (42.03) | 209 (37.86) | 217 (39.31) | 243 (43.94) | |
Non-Hispanic Black | 151 (27.36) | 117 (21.20) | 89 (16.12) | 54 (9.76) | |
Other races | 75 (13.59) | 96 (17.39) | 83 (15.04) | 83 (15.01) | |
Marital status | <0.001 | ||||
Married/living with partner | 327 (59.24) | 378 (68.48) | 384 (69.57) | 388 (70.16) | |
Widowed/divorced/separated | 80 (14.49) | 67 (12.14) | 85 (15.40) | 84 (15.19) | |
Never married | 145 (26.27) | 107 (19.38) | 83 (15.04) | 81 (14.65) | |
Education level | 0.56 | ||||
Less than 9th grade | 50 (9.06) | 46 (8.33) | 52 (9.42) | 54 (9.76) | |
9th–11th grade | 75 (13.59) | 71 (12.86) | 82 (14.86) | 81 (14.65) | |
High school graduate/GED or equivalent | 132 (23.91) | 142 (25.72) | 114 (20.65) | 118 (21.34) | |
Some college or AA degree | 138 (25.00) | 148 (26.81) | 159 (28.80) | 166 (30.02) | |
College graduate or above | 157 (28.44) | 145 (26.27) | 144 (26.09) | 134 (24.23) | |
Ratio of family income to poverty | 2.62±1.68 | 2.68±1.64 | 2.52±1.62 | 2.45±1.57 | 0.22 |
Smoked at least 100 cigarettes | 0.045 | ||||
Yes | 276 (50.00) | 289 (52.36) | 317 (57.43) | 315 (56.96) | |
No | 274 (49.64) | 262 (47.46) | 235 (42.57) | 238 (43.04) | |
Drink status | 0.28 | ||||
None or light drinker | 105 (27.06) | 102 (27.64) | 107 (26.82) | 108 (27.69) | |
Moderate drinker | 267 (68.81) | 250 (67.75) | 273 (68.42) | 251 (64.36) | |
Heavy drinker | 16 (4.12) | 17 (4.61) | 19 (4.76) | 31 (7.95) | |
Hypertension | <0.001 | ||||
Yes | 163 (29.53) | 181 (32.79) | 226 (40.94) | 224 (40.51) | |
No | 389 (70.47) | 370 (67.03) | 325 (58.88) | 328 (59.31) | |
Diabetes | <0.001 | ||||
Yes | 42 (7.61) | 71 (12.86) | 97 (17.57) | 99 (17.90) | |
No | 494 (89.49) | 468 (84.78) | 441 (79.89) | 432 (78.12) | |
Borderline | 16 (2.90) | 13 (2.36) | 14 (2.54) | 21 (3.80) | |
BMI (kg/m2) | 25.26±4.50 | 27.95±5.84 | 29.95±6.37 | 31.39±6.06 | <0.001 |
Standing height (circumference) | 152.68±38.16 | 141.98±39.36 | 134.80±37.45 | 131.24±34.60 | <0.001 |
Waist (circumference) | 91.89±12.76 | 99.42±14.90 | 105.17±15.93 | 108.17±15.10 | <0.001 |
TG (mg/dL) | 57.36±19.15 | 85.81±25.05 | 125.22±39.16 | 248.37±180.09 | <0.001 |
TC (mg/dL) | 175.63±35.11 | 178.55±37.80 | 189.62±42.44 | 201.31±45.98 | <0.001 |
LDL-C (mg/dL) | 100.90±31.07 | 110.36±33.93 | 118.79±37.49 | 118.36±38.26 | <0.001 |
HDL-C (mg/dL) | 63.27±16.40 | 51.05±10.28 | 45.76±9.19 | 36.90±7.87 | <0.001 |
Fasting glucose (mg/dL) | 5.76±1.48 | 5.98±1.48 | 6.40±2.14 | 6.91±2.79 | <0.001 |
Insulin (uU/mL) | 7.98±10.70 | 10.69±12.74 | 14.98±16.76 | 22.01±36.50 | <0.001 |
Testosterone (ng/dL) | 535.59±198.64 | 479.64±189.93 | 426.60±181.14 | 383.91±181.18 | <0.001 |
HOMA-IR index | 2.30±5.78 | 3.05±5.90 | 4.54±6.59 | 7.53±16.43 | <0.001 |
Data are presented as mean ± SD or n (%). GED, General Educational Development; AA, associates; BMI, body mass index; TG, triglyceride; TC, total cholesterol; LDL-C, low-density lipoprotein cholesterol; HDL-C, high-density lipoprotein cholesterol; HOMA-IR, homeostatic model assessment index; Q, quartile; SD, standard deviation.
Associations between CMI and testosterone
The association between CMI and testosterone is seen in Table 2. Both the crude model and the minimally adjusted model revealed a significant negative correlation between CMI and testosterone (Model 1, β=−10.58, 95% CI: −12.80, −8.37; Model 2, β=−10.56, 95% CI: −12.76, −8.36). However, in the fully adjusted model, there was no significant correlation observed (Model 3, β=−0.04, 95% CI: −4.88, 4.81). This association remained significant even when CMI was categorized into quartiles. In Model 1 and 2, the testosterone levels of the other three groups (Q2, Q3, and Q4) were significantly different from that of quartile 1 (all P<0.001). In Model 3, after comprehensive adjustment for confounding variables, testosterone levels were significantly lower in the two highest CMI groups (Q3, Q4) compared to the lowest group (Q1).
Table 2
CMI | Crude model (Model 1) | Minimally adjusted model (Model 2) | Fully adjusted model (Model 3) | |||||
---|---|---|---|---|---|---|---|---|
β (95% CI) | P | β (95% CI) | P | β (95% CI) | P | |||
Continuous | −10.58 (−12.80, −8.37) | <0.001 | −10.56 (−12.76, −8.36) | <0.001 | −0.04 (−4.88, 4.81) | 0.99 | ||
CMI quartile | ||||||||
Q1 | Reference | – | Reference | – | Reference | – | ||
Q2 | −55.95 (−78.11, −33.79) | <0.001 | −53.03 (−75.01, −31.05) | <0.001 | −13.98 (−37.17, 9.21) | 0.24 | ||
Q3 | −108.99 (−131.16, −86.83) | <0.001 | −105.27 (−127.38, −83.17) | <0.001 | −41.99 (−68.82, −15.15) | 0.002 | ||
Q4 | −151.68 (−173.83, −129.53) | <0.001 | −151.92 (−174.14, −129.70) | <0.001 | −58.71 (−93.75, −23.68) | 0.001 | ||
P for trend | <0.001 | <0.001 | <0.001 |
Model 1: no covariates were adjusted; Model 2: adjusted for age and race; Model 3: adjusted for age, race, marital status, height, body mass index, triglyceride, high-density lipoprotein, hypertension, diabetes, and smoking status. CMI, cardiometabolic index; Q, quartile; β, effect size for linear regression; 95% CI, 95% confidence interval.
Furthermore, we conducted smoother curve fitting analysis to further investigate the relationship between CMI and testosterone, which consistently demonstrated a negative association (Figure 2).
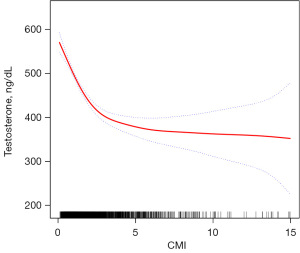
Subgroup analysis
We then conducted a subgroup analysis to assess whether the relationship between CMI and testosterone was stable across various demographic situations. As shown in Figure 3, the relationship between CMI and testosterone was affected by age, race, education level, hypertension, and smoking status (P-interaction <0.05).
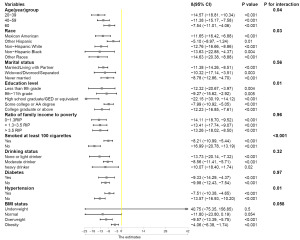
Comparison of different markers in predicting testosterone deficiency
The result of ROC is shown in Figure 4. The AUROC of CMI, TyG index, triglyceride-glucose-body mass index (TyG-BMI), HOMA-IR index and BMI in predicting testosterone deficiency was 0.68 (95% CI: 0.65, 0.71), 0.67 (95% CI: 0.65, 0.70), 0.76 (95% CI: 0.73, 0.78), 0.69 (95% CI: 0.66, 0.72) and 0.74 (95% CI: 0.71, 0.76) respectively. The results showed that TyG-BMI was similar to BMI and better than CMI, TyG, and HOMA-IR index in predicting testosterone deficiency for U.S. adult males, with a cutoff value of 223.26, a sensitivity and a specificity of 0.70 and 0.71, respectively (Table 3).
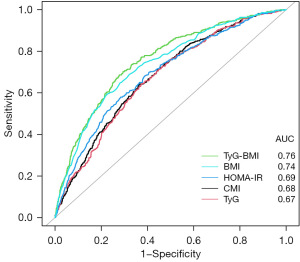
Table 3
Markers | Cutoff (sensitivity, specificity) | AUC (95% CI) |
---|---|---|
CMI | 1.31 (0.64, 0.64) | 0.68 (0.65, 0.71) |
TyG index | 8.46 (0.64, 0.62) | 0.67 (0.65, 0.70) |
TyG-BMI index | 223.26 (0.70, 0.71) | 0.76 (0.73, 0.78) |
HOMA-IR index | 1.77 (0.58, 0.72) | 0.69 (0.66, 0.72) |
BMI | 25.15 (0.60, 0.77) | 0.74 (0.71, 0.76) |
Z-test was used to compare statistically significant differences between AUCs. ROC, receiver operating characteristic CMI, cardiometabolic index; TyG, triglyceride and glucose index; BMI, body mass index; TyG-BMI, triglyceride-glucose-body mass index; HOMA-IR, Homeostatic Model Assessment for Insulin Resistance; AUC, area under the curve; CI, confidence interval.
Discussion
This cross-sectional study, which encompassed a representative sample on a national scale, sought to investigate the association between CMI and testosterone levels. The findings of our study indicated a negative correlation between elevated CMI and serum testosterone levels among adult males in the United States. Furthermore, this negative correlation appeared to be influenced by variables such as age, race, education level, hypertension, and smoking status. Additionally, our study employed ROC analysis and demonstrated that CMI outperformed TyG as a predictor of testosterone deficiency. Notably, this study represents the first of its kind to explore the relationship between CMI and testosterone in the male population, thus contributing unique insights to existing knowledge.
The synthesis of testosterone is intricately regulated by the hypothalamic-pituitary-testicular (HPT) endocrine axis, which plays a crucial role in maintaining hormonal balance. Within this axis, basophils in the anterior pituitary gland secrete luteinizing hormone (LH), which binds to G protein-coupled receptors on the surface of Leydig cells. This binding initiates a cascade of stimulation signals that promote the synthesis and secretion of testosterone within these cells (19). Testosterone, with its wide-reaching physiological effects, exerts substantial influence on diverse aspects of male biology. It contributes to the development of male reproductive organs and plays a pivotal role in musculoskeletal synthesis and metabolism. However, it is worth noting that testosterone can also stimulate the release of inflammatory factors, thereby participating in the inflammatory response (20). When testosterone levels are low, the proper functioning of multiple organs can be adversely affected (21). This can lead to conditions such as male gonadal hypogonadism and atherosclerosis, a form of cardiovascular disease characterized by the narrowing and hardening of arteries. Furthermore, low testosterone levels can increase the incidence of varicocele (enlarged veins in the scrotum), prostate enlargement, and osteoporosis. In summary, testosterone synthesis and its physiological effects are regulated by the HPT axis, and deviations from optimal testosterone levels can have wide-ranging consequences on male health, including effects on reproductive organs, musculoskeletal system, and increased risks of certain conditions.
Low levels of testosterone have been showed to be associated with various metabolic abnormalities and the development of conditions such as dyslipidemia, insulin resistance, impaired glucose regulation, obesity, hypertension, and diabetes, which are collectively known as metabolic syndrome. Macdonald et al. proposed a negative correlation between obesity and testosterone levels, as well as sex hormone-binding protein (hormone-binding globulin, SH-BG) (22). A bi-directional Mendelian randomization analysis by Joel Eriksson and colleagues showed that there was a causal effect of BMI on serum testosterone levels in men (23). A meta-analysis of 2,159 datasets from 1,774 participants shows weight loss increases testosterone levels (24). Additionally, a single-factor analysis of a cross-sectional study involving non-smoking men aged 54 or older revealed that WHtR exhibited a stronger correlation with testosterone compared to WC or BMI (25). In a study by Fan et al. involving 6,150 Chinese subjects, the levels of serum testosterone and blood lipids were measured. The results of regression analysis indicated a negative association between serum testosterone levels and lipid levels, as well as prevalent dyslipidemia (26). Furthermore, obesity was found to mediate the effects of serum testosterone on dyslipidemia in men. A cross-sectional study based on the NHANES dataset demonstrated a negative correlation between male testosterone levels and the TyG index, TyG-BMI index, and IR index (27). Moreover, several studies have suggested that testosterone levels are positively associated with HDL-C and negatively associated with TG, while no significant associations between testosterone and TC were observed in men (28-30). Overall, research indicates that low testosterone levels are linked to metabolic abnormalities, including dyslipidemia, insulin resistance, and obesity.
Previous studies have often used BMI, waist circumference, hip circumference, waist-to-hip ratio, and other measures to assess obesity and lipid metabolism when predicting testosterone levels. However, these methods have certain limitations. BMI, for instance, only provides information about overall obesity and does not differentiate between subcutaneous fat and visceral fat. It is important to note that visceral fat accumulation is more closely associated with metabolic disorders than subcutaneous fat. To address this limitation, we utilized WHtR as an improved index in our prediction model. WHtR provides a better reflection of abnormal fat metabolism within the body. Furthermore, we developed the CMI formula by incorporating WHtR along with measures of TG and HDL-C (9). This comprehensive approach allows us to obtain a more holistic understanding of obesity levels and overall lipid metabolism (31). Compared to traditional prediction models, our optimized model provides enhanced accuracy and reliability in estimating testosterone levels by considering the interplay between body fat distribution and lipid metabolism.
The mechanisms underlying the relationship between CMI and decreased testosterone levels in men remain unclear. However, there are potential mechanisms that could help explain this association. Firstly, CMI serves as an indicator of abdominal obesity, and obesity itself can directly impact testosterone levels. Increased obesity leads to elevated levels of leptin, a hormone that stimulates the release of LH through hypothalamic GnRH neurons. However, the hypothalamus may develop resistance to the effects of leptin, resulting in reduced feedback stimulation of testosterone production (32,33). Additionally, adipocytes, which are abundant in obese individuals, have high expression of aromatase and can produce inflammatory cytokines. Aromatase converts testosterone into estradiol, thereby decreasing circulating androgen levels. Moreover, elevated estrogen levels and inflammatory factors secreted by adipocytes activate a negative feedback mechanism on the hypothalamus-pituitary (HP) axis, inhibiting gonadotropin-releasing hormone (GnRH) and subsequent LH release, leading to decreased testosterone levels (3). Furthermore, studies have indicated that testosterone plays a role in improving glucose and lipid metabolism and preventing obesity-related diseases by maintaining mitochondrial function and enhancing the functionality of vascular endothelial cells (34). Importantly, recent research has suggested that testosterone levels can influence metabolic diseases induced by intestinal flora (35,36).
Our research revealed a negative correlation between CMI and male testosterone levels. Furthermore, we found that CMI outperformed the TyG index in predicting the occurrence of testosterone deficiency in adult males in the United States. While the AUROC area for CMI may be slightly lower than that of TyG-BMI, BMI, and the HOMA-IR index, CMI incorporates the influence of cardiovascular factors, thus offering further optimization compared to traditional prediction models.
The relationship between male CMI and testosterone has been relatively understudied, despite its significant clinical value. Understanding this relationship has critical implications for infertility, erectile dysfunction, and secondary hypogonadism associated with obesity. CMI serves as an important indicator for testosterone screening and comprehensive evaluation, especially in the assessment of interventions aimed at promoting testosterone health. By utilizing the CMI range of patients, a comprehensive health management plan can be formulated, focusing on improving abdominal obesity and regulating lipid levels.
However, it is important to acknowledge the limitations of this study. Firstly, it was a cross-sectional study, which restricts our ability to establish a causal relationship between CMI and testosterone. Secondly, the assessment of other visceral indexes was conducted at different time points, which may have influenced the study’s outcomes. Moreover, our findings indicate lower levels of testosterone, but higher BMI, TC, fasting blood glucose, and fasting insulin among participants, suggesting complex interactions between CMI and metabolic parameters. Therefore, further exploration of the causal relationship between CMI and testosterone is warranted through prospective studies involving larger sample populations across multiple centers.
Conclusions
Our study demonstrated that elevated CMI level was associated with decreased testosterone, with TyG-BMI emerging as the strongest predictor of testosterone deficiency. Further large-scale prospective studies are required to validate these findings and explore the predictive value of CMI and TyG-BMI across diverse populations. Future research directions should focus on elucidating the underlying mechanisms linking CMI, TyG-BMI, and testosterone levels, as well as assessing interventions targeting these metabolic parameters to improve testosterone health and mitigate associated health risks.
Acknowledgments
We are grateful for the information provided by the National Health and Nutrition Examination Survey (2013–2016) of the United States, which is used in epidemiological research and health science research to aid in the development of sound public health policies, direct and design health plans and services, and advance health knowledge.
Funding: This study was supported by
Footnote
Reporting Checklist: The authors have completed the STROBE reporting checklist. Available at https://tau.amegroups.com/article/view/10.21037/tau-24-121/rc
Peer Review File: Available at https://tau.amegroups.com/article/view/10.21037/tau-24-121/prf
Conflicts of Interest: All authors have completed the ICMJE uniform disclosure form (available at https://tau.amegroups.com/article/view/10.21037/tau-24-121/coif). The authors have no conflicts of interest to declare.
Ethical Statement: The authors are accountable for all aspects of the work in ensuring that questions related to the accuracy or integrity of any part of the work are appropriately investigated and resolved. The study was conducted in accordance with the Declaration of Helsinki (as revised in 2013). Health information collected in the NHANES is kept in strictest confidence. During the informed consent process, survey participants were assured that data collected will be used only for stated purposes and will not be disclosed or released to others without the consent of the individual or the establishment in accordance with Section 308 (d) of the Public Health Service Act (42 U.S.C. 242m).
Open Access Statement: This is an Open Access article distributed in accordance with the Creative Commons Attribution-NonCommercial-NoDerivs 4.0 International License (CC BY-NC-ND 4.0), which permits the non-commercial replication and distribution of the article with the strict proviso that no changes or edits are made and the original work is properly cited (including links to both the formal publication through the relevant DOI and the license). See: https://creativecommons.org/licenses/by-nc-nd/4.0/.
References
- Orwoll E. Safety of Testosterone-Replacement Therapy in Older Men. N Engl J Med 2023;389:177-8. [Crossref] [PubMed]
- Lincoff AM, Bhasin S, Flevaris P, et al. Cardiovascular Safety of Testosterone-Replacement Therapy. N Engl J Med 2023;389:107-17. [Crossref] [PubMed]
- Kelly DM, Jones TH. Testosterone and obesity. Obes Rev 2015;16:581-606. [Crossref] [PubMed]
- Whittaker J. Dietary trends and the decline in male reproductive health. Hormones (Athens) 2023;22:165-97. [Crossref] [PubMed]
- Wittert G, Bracken K, Robledo KP, et al. Testosterone treatment to prevent or revert type 2 diabetes in men enrolled in a lifestyle programme (T4DM): a randomised, double-blind, placebo-controlled, 2-year, phase 3b trial. Lancet Diabetes Endocrinol 2021;9:32-45. [Crossref] [PubMed]
- Kapoor D, Goodwin E, Channer KS, et al. Testosterone replacement therapy improves insulin resistance, glycaemic control, visceral adiposity and hypercholesterolaemia in hypogonadal men with type 2 diabetes. Eur J Endocrinol 2006;154:899-906. [Crossref] [PubMed]
- do Val Lima PR, Ronconi KS, Morra EA, et al. Testosterone deficiency impairs cardiac interfibrillar mitochondrial function and myocardial contractility while inducing oxidative stress. Front Endocrinol (Lausanne) 2023;14:1206387. [Crossref] [PubMed]
- Pal M, Khan J, Kumar R, et al. Testosterone supplementation improves insulin responsiveness in HFD fed male T2DM mice and potentiates insulin signaling in the skeletal muscle and C2C12 myocyte cell line. PLoS One 2019;14:e0224162. [Crossref] [PubMed]
- Wakabayashi I, Daimon T. The "cardiometabolic index" as a new marker determined by adiposity and blood lipids for discrimination of diabetes mellitus. Clin Chim Acta 2015;438:274-8. [Crossref] [PubMed]
- Duan S, Yang D, Xia H, et al. Cardiometabolic index: A new predictor for metabolic associated fatty liver disease in Chinese adults. Front Endocrinol (Lausanne) 2022;13:1004855. [Crossref] [PubMed]
- Cai X, Hu J, Wen W, et al. Associations of the Cardiometabolic Index with the Risk of Cardiovascular Disease in Patients with Hypertension and Obstructive Sleep Apnea: Results of a Longitudinal Cohort Study. Oxid Med Cell Longev 2022;2022:4914791. [Crossref] [PubMed]
- Miao M, Deng X, Wang Z, et al. Cardiometabolic index is associated with urinary albumin excretion and renal function in aged person over 60: Data from NHANES 2011-2018. Int J Cardiol 2023;384:76-81. [Crossref] [PubMed]
- Dursun M, Besiroglu H, Otunctemur A, et al. Association between cardiometabolic index and erectile dysfunction: A new index for predicting cardiovascular disease. Kaohsiung J Med Sci 2016;32:620-3. [Crossref] [PubMed]
- Shi WR, Wang HY, Chen S, et al. Estimate of prevalent diabetes from cardiometabolic index in general Chinese population: a community-based study. Lipids Health Dis 2018;17:236. [Crossref] [PubMed]
- Xue H, Zou Y, Yang Q, et al. The association between different physical activity (PA) patterns and cardiometabolic index (CMI) in US adult population from NHANES (2007-2016). Heliyon 2024;10:e28792. [Crossref] [PubMed]
- Cuschieri S. The STROBE guidelines. Saudi J Anaesth 2019;13:S31-4. [Crossref] [PubMed]
- Johnson CL, Dohrmann SM, Burt VL, et al. National health and nutrition examination survey: sample design, 2011-2014. Vital Health Stat 2 2014;1-33. [PubMed]
- Mulhall JP, Trost LW, Brannigan RE, et al. Evaluation and Management of Testosterone Deficiency: AUA Guideline. J Urol 2018;200:423-32. [Crossref] [PubMed]
- Navarro VM, Tena-Sempere M. Neuroendocrine control by kisspeptins: role in metabolic regulation of fertility. Nat Rev Endocrinol 2011;8:40-53. [Crossref] [PubMed]
- Gooren LJ. Androgens and male aging: Current evidence of safety and efficacy. Asian J Androl 2010;12:136-51. [Crossref] [PubMed]
- Kelly DM, Akhtar S, Sellers DJ, et al. Testosterone differentially regulates targets of lipid and glucose metabolism in liver, muscle and adipose tissues of the testicular feminised mouse. Endocrine 2016;54:504-15. [Crossref] [PubMed]
- Macdonald AA, Stewart AW, Farquhar CM. Body mass index in relation to semen quality and reproductive hormones in New Zealand men: a cross-sectional study in fertility clinics. Hum Reprod 2013;28:3178-87. [Crossref] [PubMed]
- Eriksson J, Haring R, Grarup N, et al. Causal relationship between obesity and serum testosterone status in men: A bi-directional mendelian randomization analysis. PLoS One 2017;12:e0176277. [Crossref] [PubMed]
- Ken-Dror G, Fluck D, Fry CH, et al. Meta-analysis and construction of simple-to-use nomograms for approximating testosterone levels gained from weight loss in obese men. Andrology 2024;12:297-315. [Crossref] [PubMed]
- Allan CA, Peverill RE, Strauss BJ, et al. Waist-to-height ratio as a predictor of serum testosterone in ageing men with symptoms of androgen deficiency. Asian J Androl 2011;13:424-31. [Crossref] [PubMed]
- Fan C, Wei D, Wang L, et al. The association of serum testosterone with dyslipidemia is mediated by obesity: the Henan Rural Cohort Study. J Endocrinol Invest 2023;46:679-86. [Crossref] [PubMed]
- Wu S, Wu Y, Fang L, et al. A negative association between triglyceride glucose-body mass index and testosterone in adult males: a cross-sectional study. Front Endocrinol (Lausanne) 2023;14:1187212. [Crossref] [PubMed]
- Akishita M, Fukai S, Hashimoto M, et al. Association of low testosterone with metabolic syndrome and its components in middle-aged Japanese men. Hypertens Res 2010;33:587-91. [Crossref] [PubMed]
- Gomes GK, de Branco FMS, Santos HO, et al. Cholesterol intake and serum total cholesterol levels are not associated with total testosterone levels in men: a cross-sectional study from NHANES 2013-2014. Lipids Health Dis 2023;22:168. [Crossref] [PubMed]
- Agledahl I, Skjaerpe PA, Hansen JB, et al. Low serum testosterone in men is inversely associated with non-fasting serum triglycerides: the Tromsø study. Nutr Metab Cardiovasc Dis 2008;18:256-62. [Crossref] [PubMed]
- Sato F, Nakamura Y, Kayaba K, et al. TG/HDL-C ratio as a predictor of stroke in the population with healthy BMI: The Jichi Medical School Cohort Study. Nutr Metab Cardiovasc Dis 2022;32:1872-9. [Crossref] [PubMed]
- Lima TFN, Nackeeran S, Rakitina E, et al. Association of Leptin with Total and Free Testosterone: Results from the National Health and Nutrition Examination Surveys. Androg Clin Res Ther 2020;1:94-100. [PubMed]
- Navarro G, Allard C, Xu W, et al. The role of androgens in metabolism, obesity, and diabetes in males and females. Obesity (Silver Spring) 2015;23:713-9. [Crossref] [PubMed]
- Yoshida S, Ikeda Y, Aihara K. Roles of the Androgen--Androgen Receptor System in Vascular Angiogenesis. J Atheroscler Thromb 2016;23:257-65. [Crossref] [PubMed]
- Liang Z, Di N, Li L, et al. Gut microbiota alterations reveal potential gut-brain axis changes in polycystic ovary syndrome. J Endocrinol Invest 2021;44:1727-37. [Crossref] [PubMed]
- Sherman SB, Sarsour N, Salehi M, et al. Prenatal androgen exposure causes hypertension and gut microbiota dysbiosis. Gut Microbes 2018;9:400-21. [Crossref] [PubMed]