Impact of diabetes mellitus and hypertension on renal function during first-line targeted therapy for metastatic renal cell carcinoma: a retrospective multicenter study
Highlight box
Key findings
• Patients with diabetes mellitus (DM) and hypertension (HTN) experienced further decline in renal function and had a higher risk of progression to stage 4 chronic kidney disease (CKD) after targeted therapy compared to patients without these comorbidities.
What is known and what is new?
• Although HTN and DM are the most common factors influencing the development and progression of CKD, their impact on renal function during targeted therapy is unclear.
• Patients with DM and HTN had further reduced renal function and were more likely to progress to stage 4 CKD than patients without these risk factors. The coexistence of DM and HTN was a significant predictor of progression to stage 4 CKD after targeted therapy.
What is the implication, and what should change now?
• Recognition and proactive management of DM and HTN are necessary to facilitate the proper administration of life-prolonging oncologic treatments.
Introduction
Renal cell carcinoma (RCC) accounts for 3% of all new malignancies worldwide (1,2). Approximately, one-third of patients have metastatic disease at initial diagnosis and 20–30% of cases of surgically resected localized disease subsequently develop local recurrence or distant metastasis (3,4). Significant improvement in the prognosis of recurrent or metastatic RCC (mRCC) has been achieved with the introduction of molecular targeted agents, such as vascular endothelial growth factor (VEGF)-targeting tyrosine kinase inhibitors (TKIs) and mammalian target of rapamycin inhibitors (mTORi) (5). Among the various targeted therapies, TKI-based sequential therapy has been widely used for the treatment of mRCC for several decades. More recently, TKIs were combined with immune checkpoint inhibitors (ICIs) for the treatment of all International Metastatic Renal Cell Carcinoma Database Consortium (IMDC) risk groups (6,7). Specifically, compared to the ICI + ICI combination, patients who received ICI + TKI combinations showed longer progression-free survival (PFS) and overall survival (OS) in the IMDC intermediate risk group, while similar oncologic outcomes were observed in the IMDC poor risk patients (8). As a result, ICI+TKI combinations have become the current standard of care for advanced mRCC. Furthermore, TKIs are recommended as an alternative treatment for mRCC patients who experienced severe adverse events (AEs) during ICI-based treatment or progressed disease after ICI treatment (6,7). In this context, TKIs continue to play an important role in the management of advanced RCC or mRCC.
Despite an improved prognosis, renal function deterioration during systemic therapy in patients with mRCC or advanced RCC is a long-term concern for treatment planning. However, whether TKIs directly cause renal function deterioration or whether the glomerular filtration rate (GFR) declines significantly after TKI therapy remains controversial (9-11). In particular, in patients with chronic kidney disease (CKD), whether TKIs exacerbate the decline in renal function is a focus of interest and debate.
Hypertension (HTN) and diabetes mellitus (DM) are the most common and important factors that affect CKD development and progression (12,13). In real-world clinical practice, patients with DM and HTN are common among those with surgical CKD resulting from nephrectomy or medical CKD caused by aging and the presence of comorbidities. However, the impact of DM and HTN on renal function in patients with mRCC receiving TKI treatment remains unclear. Thus, this study aimed to determine the impact of DM and HTN on renal function during first-line targeted therapy for patients with mRCC. We present this article in accordance with the STROBE reporting checklist (available at https://tau.amegroups.com/article/view/10.21037/tau-24-231/rc).
Methods
Study approval and patient selection
This was a multicenter, retrospective study. This study was conducted in accordance with the Declaration of Helsinki (as revised in 2013) and was approved by the institutional ethics review board of Inje University Busan Paik Hospital (BPIRB 2023-12-037). The informed consent for this retrospective and observational study was waived according to the provisions of the ethics committee and the ethics guideline in South Korea. A total of 527 patients with mRCC treated with either sunitinib or pazopanib as first-line therapy from February 2007 to February 2019 were retrospectively recruited from four tertiary medical centers in South Korea. Patients who had no follow-up data on renal function and the oncologic outcome of first-line systemic therapy, had stage 4 or 5 CKD, were undergoing hemodialysis at the start of targeted therapy, or had a history of other targeted therapies were excluded (n=103), and the final study cohort comprised 424 patients. Demographic, clinical, and laboratory variables at the time of metastasis diagnosis were collected, including age, gender, prior nephrectomy status, DM and HTN status, and IMDC risk score. Patients with HTN were defined as those whose blood pressure was >140/90 mmHg or who were receiving antihypertensive agents. Patients with DM were defined as those who were receiving hypoglycemic agents and/or insulin injections. The patients were classified into four groups according to their DM and HTN status as follows: group 1, HTN−, DM−; group 2, HTN+, DM−; group 3, HTN−, DM+; and group 4, HTN+, DM+.
Treatment with targeted agents
The targeted agents administered included sunitinib (50 mg orally, once daily in repeated 6-week cycles consisting of 4 weeks on followed by 2 weeks off or 2 weeks on followed by 1 week off) or pazopanib (800 mg orally, once daily). The targeted agents were administered until disease progression or the development of intolerable AEs. The dose was reduced or interrupted based on the guidelines for each agent and the patient’s general condition. AEs were graded using Common Terminology Criteria for Adverse Events v4.0. Progression was defined as clinical progression or fulfillment of radiographic criteria using Response Evaluation Criteria in Solid Tumors v1.1 (14).
Renal function evaluation
Renal function was determined at the initiation and end of targeted therapy based on the estimated GFR (eGFR) using the modification of diet in renal disease formula: eGFR (mL/min/1.73 m2) = 186 × (serum creatinine)−1.154 × (age)−0.203 × (0.742 if female) (15). The change in eGFR (ΔeGFR) was defined as the difference between eGFR at the initiation and termination of first-line targeted therapy. For the detection of actual renal function changes, we calculated the percent ΔeGFR [%ΔeGFR = (ΔeGFR/pretreatment eGFR) ×100] (16,17). The eGFR value at the start and end of targeted therapy was used to define the CKD stage of each patient as follows: stage 1 (>90 mL/min/1.73 m2), stage 2 (60–89.9 mL/min/1.73 m2), stage 3a (45–59.9 mL/min/1.73 m2), stage 3b (30–44.9 mL/min/1.73 m2), and stage 4 (15–29.9 mL/min/1.73 m2) (18).
Statistical analysis
Continuous variables were presented as means with standard deviations or medians with interquartile ranges (IQRs). Categorical variables were presented as frequencies with percentages. Differences in variable distribution among groups were evaluated using Pearson Chi-squared test and linear-by-linear association for categorical variables and Student’s t-test and one-way analysis of variance for continuous variables. The eGFR values before and after first-line targeted therapy were compared using a paired t-test. Progression to stage 4 CKD after first-line targeted therapy was estimated using the Kaplan-Meier method and compared using the log-rank test. Univariate logistic regression was used to identify clinicopathological factors that might have affected the progression to stage 4 CKD after treatment. Finally, a multivariate logistic regression model, which was used in conjunction with the standard entry method, was applied to potential covariates. The odd ratios (ORs) and 95% confidence intervals (CIs) were determined using the reference group. Statistical analysis was performed using SPSS v27.0 (IBM Corp., Armonk, NY, USA) and MedCalc v22.0 (MedCalc Software, Ostend, Belgium). A two-sided P value <0.05 was considered statistically significant for all tests.
Results
The median patient age at the start of first-line targeted therapy was 60.0 years (IQR, 43–85 years). Of the 424 patients with mRCC, 303 (71.5%) and 121 (28.5%) were treated with sunitinib or pazopanib, respectively, as first-line treatment. The median duration of first-line targeted therapy was 10.3 months (IQR, 3.1–37.0 months). Overall, 316 (74.5%) patients died after the initiation of first-line targeted therapy, with a median follow-up of 30.4 months (IQR, 4.6–92.5 months).
Patients were allocated to four groups according to their DM and HTN status, and their clinicopathological characteristics are summarized in Table 1. There were no intergroup differences in baseline characteristics, including the CKD stage before targeted therapy and treatment duration.
Table 1
Characteristics | Hypertension and diabetes mellitus status | P value | |||
---|---|---|---|---|---|
Group 1 (HTN−, DM−) (n=194) | Group 2 (HTN+, DM−) (n=136) | Group 3 (HTN−, DM+) (n=32) | Group 4 (HTN+, DM+) (n=62) | ||
Age (years) | 58 [45–83] | 61 [43–84] | 62 [46–79] | 61 [45–79] | 0.07 |
Sex | |||||
Male | 149 (76.8) | 106 (77.9) | 28 (87.5) | 52 (83.9) | 0.14 |
Female | 45 (23.2) | 30 (22.1) | 4 (12.5) | 10 (16.1) | |
No. of metastatic organ | |||||
Single | 95 (49.0) | 72 (52.9) | 8 (25.0) | 30 (48.4) | 0.13 |
Two | 61 (31.4) | 44 (32.4) | 13 (40.6) | 18 (29.0) | |
Three | 34 (17.5) | 11 (8.1) | 9 (28.1) | 8 (12.9) | |
≥ Four | 4 (2.1) | 9 (6.6) | 2 (6.3) | 6 (9.7) | |
Timing of metastasis | |||||
Synchronous | 111 (57.2) | 83 (61.0) | 14 (43.8) | 32 (51.6) | 0.29 |
Metachronous | 83 (42.8) | 53 (39.0) | 18 (56.2) | 30 (48.4) | |
IMDC risk groups | |||||
Favorable | 44 (22.7) | 36 (26.5) | 5 (15.6) | 9 (14.5) | 0.15 |
Intermediate | 127 (65.5) | 90 (66.2) | 21 (65.6) | 44 (71.0) | |
Poor | 23 (11.9) | 10 (7.3) | 6 (18.8) | 9 (14.5) | |
Nephrectomy | |||||
No | 39 (20.1) | 25 (18.4) | 3 (9.4) | 12 (19.4) | 0.56 |
Yes | 155 (79.9) | 111 (81.6) | 29 (90.6) | 50 (80.6) | |
Stage of CKD before targeted therapy | |||||
CKD 1 | 37 (19.1) | 19 (13.9) | 2 (6.3) | 7 (11.3) | 0.16 |
CKD 2 | 93 (47.9) | 70 (51.5) | 21 (65.6) | 31 (50.0) | |
CKD 3a | 49 (25.3) | 36 (26.5) | 7 (21.9) | 17 (27.4) | |
CKD 3b | 15 (7.7) | 11 (8.1) | 2 (6.3) | 7 (11.3) | |
First-line targeted agent | |||||
Sunitinib | 142 (73.2) | 93 (68.4) | 23 (71.9) | 45 (72.6) | 0.85 |
Pazopanib | 52 (26.8) | 43 (31.6) | 9 (28.1) | 17 (27.4) | |
Duration of treatment (months) | 10.5 [3.0–36.7] | 9.7 [3.1–31.6] | 10.6 [4.2–44.1] | 10.5 [3.2–37.8] | 0.78 |
Data are presented as median [IQR] and n (%). DM, diabetes mellitus; HTN, hypertension; IMDC, international mRCC database consortium; CKD, chronic kidney disease; IQR, interquartile range; mRCC, metastatic renal cell carcinoma.
There were no intergroup differences in mean eGFR before targeted therapy (Table 2). The pretreatment mean eGFR was 70.0±21.4 mL/min/1.73 m2, and it decreased significantly to 60.8±22.3 mL/min/1.73 m2 after treatment (P<0.001) (Figure 1A). In patients with stage 1 and 2 CKD, the post-treatment mean eGFR was significantly lower than the pretreatment mean eGFR (82.5±27.4 and 61.6±19.0 mL/min/1.73 m2 vs. 107.6±16.4 and 72.3±8.5 mL/min/1.73 m2, respectively; all, P<0.001) (Figure 1B). Although the mean eGFR decreased after treatment in all groups (P<0.001 for group 1, group 2, and group 4, P=0.02 for group 3, respectively) (Figure 1C), there were no significant differences in ΔeGFR between groups (P=0.10) (Table 2). However, the actual change in renal function as determined by %ΔeGFR showed differences in renal function change between groups (P=0.02) (Table 2), and the %ΔeGFR of group 4 was significantly lower compared with group 1 (P=0.008) (Figure 1D).
Table 2
Characteristics | Group 1 (HTN−, DM−) | Group 2 (HTN+, DM−) | Group 3 (HTN−, DM+) | Group 4 (HTN+, DM+) | P value |
---|---|---|---|---|---|
Pre-treatment: mean eGFR (mL/min/1.73 m2) | 71.6±20.9 | 70.1±23.5 | 67.1±17.3 | 66.2±19.8 | 0.30 |
Post-treatment: mean eGFR (mL/min/1.73 m2) | 64.8±22.1 | 59.5±19.7 | 57.8±23.2 | 52.4±25.1 | 0.001 |
ΔeGFR (mL/min/1.73 m2) | −6.7±18.6 | −10.6±22.8 | −9.1±21.7 | −13.8±22.6 | 0.10 |
%ΔeGFR (%) | −7.2±24.4 | −11.6±30.3 | −11.7±30.3 | −19.9±33.9 | 0.02 |
Data are presented as mean ± SD. DM, diabetes mellitus; HTN, hypertension; eGRF, estimated glomerular filtration rate; SD, standard deviation.
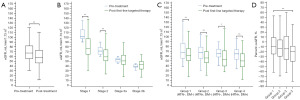
After first-line therapy, 22 (5.2%) patients progressed to stage 4 CKD, and the estimated probability of such progression increased with worsening baseline CKD grade (log-rank test, P=0.003) (Figure 2A). The mean time for progression to stage 4 CKD in group 4 was 38.6 months (95% CI: 33.9–43.4), which was significantly shorter compared with groups 1, 2, and 3 [85.6 months (95% CI: 82.0–89.2), 63.9 months (95%: CI: 61.3–66.6), and 40.7 months (95% CI: 36.1–45.3), respectively; P<0.001] (Figure 2B). Multivariate analysis revealed increased age (OR: 1.07, 95% CI: 1.01–1.13; P=0.008), increased number of metastatic sites (OR: 1.48, 95% CI: 1.01–2.20; P=0.047), and DM and HTN coexistence (OR: 10.13, 95% CI: 3.06–33.55; P<0.001) as predictors of progression to stage 4 CKD (Table 3). There was no difference between the groups in the incidence of AEs ≥ grade 3 during first-line targeted therapy. However, compared with other patients, those with DM and those with coexisting DM and HTN more frequently experienced ≥50% dose reduction of targeted therapy than patients without DM and HTN (40.6% vs. 37.1% vs. 17.5%; P<0.001) (Table 4).
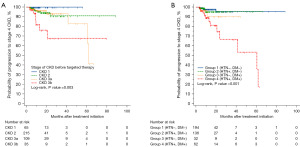
Table 3
Variables | Univariate analysis | Multivariate analysis | |||||
---|---|---|---|---|---|---|---|
OR | 95% CI | P value | OR | 95% CI | P value | ||
Age (years) | 1.06 | 1.01−1.12 | 0.009 | 1.07 | 1.01−1.13 | 0.008 | |
Sex (male vs. female) | 1.11 | 0.39−3.11 | 0.84 | ||||
HTN and DM status | |||||||
Group 1 (HTN−, DM−) | 1 | − | 1 | − | |||
Group 2 (HTN+, DM−) | 1.44 | 0.35−5.86 | 0.61 | 1.17 | 0.28−4.86 | 0.83 | |
Group 3 (HTN−, DM+) | 3.17 | 0.56−18.05 | 0.19 | 2.35 | 0.39−13.86 | 0.34 | |
Group 4 (HTN+, DM+) | 11.40 | 3.52−36.86 | <0.001 | 10.13 | 3.06−33.55 | <0.001 | |
Nephrectomy status (no vs. yes) | 1.48 | 0.43−5.12 | 0.54 | ||||
Timing of metastasis (synchronous vs. metachronous) | 1.32 | 0.56−3.12 | 0.52 | ||||
IMDC risk groups | |||||||
Favorable | 1 | − | |||||
Intermediate | 2.58 | 0.58−11.51 | 0.21 | ||||
Poor | 5.35 | 0.99−28.68 | 0.051 | ||||
No. of metastatic sites (every 1 site increase) | 1.54 | 1.05−2.25 | 0.03 | 1.48 | 1.01−2.20 | 0.047 |
CKD, chronic kidney disease; HTN, hypertension; DM, diabetes mellitus; IMDC, international mRCC database consort; OR, odds ratio; CI, confidence interval; mRCC, metastatic renal cell carcinoma.
Table 4
Variables | Group 1 (HTN−, DM−) (n=194) | Group 2 (HTN+, DM−) (n=136) | Group 3 (HTN−, DM+) (n=32) | Group 4 (HTN+, DM+) (n=62) | P value |
---|---|---|---|---|---|
Grade 3 AEs | 48 (24.7) | 26 (19.1) | 11 (34.4) | 20 (32.3) | 0.19 |
Grade 4 AEs | 1 (0.5) | 1 (0.7) | 1 (3.1) | 2 (3.2) | 0.08 |
Dose reduction of targeted therapy | 120 (61.9) | 86 (63.2) | 17 (53.1) | 45 (72.6) | 0.29 |
≥50% dose reduction of targeted therapy | 34 (17.5) | 30 (22.1) | 13 (40.6) | 23 (37.1) | <0.001 |
Discontinuation of targeted therapy | 21 (10.8) | 20 (14.7) | 5 (15.6) | 10 (16.1) | 0.22 |
Discontinuation of targeted therapy for more than 3 weeks | 14 (7.2) | 17 (12.5) | 2 (6.3) | 7 (11.3) | 0.38 |
Data are presented as n (%). AEs, adverse events; HTN, hypertension; DM, diabetes mellitus.
Discussion
Following the introduction of TKI-based targeted therapies, there has been a steady stream of retrospective studies addressing concerns about the potential for drug-induced renal function deterioration (9-11). To date, no definitive conclusions regarding the association between decline of renal function and use of TKI have been reported, and this remains controversial. Population pharmacokinetic analyses did not reveal any correlation between TKI exposure and renal function in subjects with mild, moderate, or severe renal impairment who were not on dialysis (11). However, similar to our study, several investigations have indicated that extended TKI treatment might result in renal function decline (9,10,19). The most important cause of overall renal function decline in patients undergoing TKI treatment is the aggravation of preexisting renal impairment (20). The discrepancies among previous studies are due to differences in the main parameters used to describe changes in renal function. Studies reporting no effect of TKIs on renal function used simple, traditional parameters of renal function change, such as eGFR reduction or changes in the CKD stage after TKI treatment. However, these parameters have limitations in reflecting actual changes in renal function and progression to renal failure in patients with different levels of baseline renal function. For example, as shown in our study, although patients with stage 1 or 2 CKD showed a greater decline in eGFR following TKI treatment than patients with stage 3 CKD, progression to stage 4 CKD was rarely observed in patients with stage 1 or 2 CKD. Therefore, to compensate for these shortcomings and examine the actual changes in renal function more closely, we used more detailed approaches, such as post-treatment changes in eGFR (ΔeGFR), %ΔeGFR, and changes in CKD stage (16,17), which resulted in the demonstration of declined renal function after TKI treatment and revealed its association with HTN and DM.
To the best of our knowledge, this is the first study to demonstrate DM and HTN as major contributors to renal deterioration in patients with mRCC receiving TKIs. Both HTN and DM are generally recognized as the most important factors influencing CKD progression (12,13). This study showed that renal function in patients with DM and HTN was further reduced after TKI treatment compared with patients without these risk factors. Specifically, patients with DM and HTN had a higher rate of progression to stage 4 CKD and a shorter time to progression to stage 4 CKD than patients without these risk factors.
Considering the mechanism of action of TKIs, it is not unexpected that the most common adverse effect of TKI treatment is HTN (21) and that the incidence of HTN is dose related (22). The proposed mechanism of TKI-induced HTN involves reduced formation of nitric oxide by endothelial cells, increased production of vasoconstrictive factors, and reduced microvascular density (rarefaction) (21,23). This process induces endothelial injury and further accelerates glomerular thrombotic microangiopathy (TMA). Therefore, the cause of further decline in renal function in patients with HTN in our study was the addition of TKI-induced HTN to the HTN preexisting before TKI treatment, which further aggravated the decline in renal function. The decreased renal function after treatment in patients with DM is related to the mechanism of diabetic nephropathy. Under physiological conditions, VEGF is a paracrine-secreted product of podocytes that is involved in endothelial cell homeostasis (24). In early-stage diabetic nephropathy, excessive VEGF production by podocytes induces abnormal renal pathology. However, as the disease progresses, podocyte necrosis and glomerulosclerosis prevent VEGF production (25). This leads to the development of renal TMA, causing further endothelial cell damage and end-stage renal disease. Additionally, the use of TKIs for mRCC treatment may accelerate and worsen TMA because TKIs, including sunitinib and pazopanib, are associated with drug-induced TMA (26). Cases of diabetic nephropathy complicated with renal TMA lesions deteriorate more rapidly than uncomplicated cases of diabetic neuropathy, and decreased VEGF expression in the glomeruli is correlated with decreased eGFR (27,28). Given these mechanisms, it is unsurprising that TKI use and the presence of DM and HTN in patients with mRCC may accelerate the decline in renal function due to the adverse effects of these three factors on each other.
Compared with patients with normal renal function, patients with mRCC and impaired renal function treated with anti-VEGF drugs did not differ in response rate, time to treatment failure, and OS (29). However, Khan et al. reported that kidney injury development during treatment was a risk factor for progressive decline in renal function and increased the risk of dose reduction due to renal insufficiency (9). This phenomenon was also observed in our study. Compared with patients without DM and HTN, patients with both DM and HTN had a greater likelihood of >50% dose reduction of targeted therapy. It is important to recognize that our study provides evidence for close monitoring and management of blood pressure and diabetes control in patients with DM and HTN receiving TKI treatment. Our findings demonstrate that DM and HTN at the time of TKI initiation could lead to treatment interruptions and increase the risk of CKD progression. However, there are still gaps in our knowledge regarding the coexistence of DM and HTN at the time of TKI initiation and the optimal management of these comorbidities. The management of DM and HTN in patients receiving TKI or other anticancer therapy is largely empirical, with no current guidelines or recommendations supporting specific agents or treatment goals in this unique population. Therefore, future research should focus on investigating the impact of DM and HTN on disease progression, renal function, and treatment outcomes in patients with mRCC who receive systemic therapy. The research should address epidemiological aspects related to common risk factors and mechanisms of renal deterioration, as well as explore strategies for managing DM and HTN during and after systemic therapy.
Although this was a multicenter study, our findings were limited by its retrospective and observational design. First, unmeasured or immeasurable confounders of renal function and treatment interruption due to other side effects during TKI treatment may have affected our results. Second, although we investigated the association between the prevalence of DM, HTN, and reduced renal function, we did not analyze whether active control of this comorbidity was achieved or the effect of comorbidity control on renal function. In addition, the impact of DM exacerbated by antineoplastic treatment and new-onset HTN secondary to TKI treatment on renal function and treatment interruption should be investigated in future studies. Third, data on the presence of proteinuria, which is considered an indicator of renal impairment when using TKIs, and the urinary albumin-to-creatinine ratio, which is a more reliable proteinuria assessment parameter, were unavailable due to our study’s retrospective design. Fourth, our study did not include patients who received ICI-based systemic treatment, which was recently proposed as the standard of care for mRCC. Although ICI-based systemic therapy is the standard of care, long-term follow-up data on ICI-based first-line treatment of mRCC in clinical practice are lacking. Therefore, there is a need for further studies to fill in the gaps and limitations of the present study.
Conclusions
Long-term first-line TKI treatment in patients with mRCC was associated with declined renal function. Additionally, preexisting comorbidities, such as DM and HTN, may accelerate the decline in renal function. Because treatment discontinuation or modification due to comorbidities may pose difficulties in achieving long-term disease control, recognition and proactive management of DM and HTN are necessary to facilitate the proper administration of life-prolonging oncological treatments.
Acknowledgments
Funding: This work was supported by
Footnote
Reporting Checklist: The authors have completed the STROBE reporting checklist. Available at https://tau.amegroups.com/article/view/10.21037/tau-24-231/rc
Data Sharing Statement: Available at https://tau.amegroups.com/article/view/10.21037/tau-24-231/dss
Peer Review File: Available at https://tau.amegroups.com/article/view/10.21037/tau-24-231/prf
Conflicts of Interest: All authors have completed the ICMJE uniform disclosure form (available at https://tau.amegroups.com/article/view/10.21037/tau-24-231/coif). C.H.L. reports the funding from the 2023 Inje University research grant. The other authors have no conflicts of interest to declare.
Ethical Statement: The authors are accountable for all aspects of the work in ensuring that questions related to the accuracy or integrity of any part of the work are appropriately investigated and resolved. This study was conducted in accordance with the Declaration of Helsinki (as revised in 2013) and was approved by the institutional ethics review board of Inje University Busan Paik Hospital (BPIRB 2023-12-037). The informed consent for this retrospective and observational study was waived according to the provisions of the ethics committee and the ethics guideline in South Korea.
Open Access Statement: This is an Open Access article distributed in accordance with the Creative Commons Attribution-NonCommercial-NoDerivs 4.0 International License (CC BY-NC-ND 4.0), which permits the non-commercial replication and distribution of the article with the strict proviso that no changes or edits are made and the original work is properly cited (including links to both the formal publication through the relevant DOI and the license). See: https://creativecommons.org/licenses/by-nc-nd/4.0/.
References
- Capitanio U, Bensalah K, Bex A, et al. Epidemiology of Renal Cell Carcinoma. Eur Urol 2019;75:74-84. [Crossref] [PubMed]
- Bukavina L, Bensalah K, Bray F, et al. Epidemiology of Renal Cell Carcinoma: 2022 Update. Eur Urol 2022;82:529-42. [Crossref] [PubMed]
- Chandrasekar T, Klaassen Z, Goldberg H, et al. Metastatic renal cell carcinoma: Patterns and predictors of metastases-A contemporary population-based series. Urol Oncol 2017;35:661.e7-661.e14. [Crossref] [PubMed]
- Dabestani S, Marconi L, Kuusk T, et al. Follow-up after curative treatment of localised renal cell carcinoma. World J Urol 2018;36:1953-9. [Crossref] [PubMed]
- Choueiri TK, Motzer RJ. Systemic Therapy for Metastatic Renal-Cell Carcinoma. N Engl J Med 2017;376:354-66. [Crossref] [PubMed]
- Ljungberg B, Albiges L, Abu-Ghanem Y, et al. European Association of Urology Guidelines on Renal Cell Carcinoma: The 2022 Update. Eur Urol 2022;82:399-410. [Crossref] [PubMed]
- Motzer RJ, Jonasch E, Agarwal N, et al. NCCN Guidelines® Insights: Kidney Cancer, Version 2.2024. J Natl Compr Canc Netw 2024;22:4-16. [Crossref] [PubMed]
- Santoni M, Buti S, Myint ZW, et al. Real-world Outcome of Patients with Advanced Renal Cell Carcinoma and Intermediate- or Poor-risk International Metastatic Renal Cell Carcinoma Database Consortium Criteria Treated by Immune-oncology Combinations: Differential Effectiveness by Risk Group? Eur Urol Oncol 2024;7:102-11. [Crossref] [PubMed]
- Khan G, Golshayan A, Elson P, et al. Sunitinib and sorafenib in metastatic renal cell carcinoma patients with renal insufficiency. Ann Oncol 2010;21:1618-22. [Crossref] [PubMed]
- Ishihara H, Kondo T, Fukuda H, et al. Evaluation of renal function change during first-line tyrosine kinase inhibitor therapy for metastatic renal cell carcinoma. Jpn J Clin Oncol 2017;47:1175-81. [Crossref] [PubMed]
- Mielczarek Ł, Brodziak A, Sobczuk P, et al. Renal toxicity of targeted therapies for renal cell carcinoma in patients with normal and impaired kidney function. Cancer Chemother Pharmacol 2021;87:723-42. [Crossref] [PubMed]
- Kazancioğlu R. Risk factors for chronic kidney disease: an update. Kidney Int Suppl (2011) 2013;3:368-71. [PubMed]
- Banerjee D, Winocour P, Chowdhury TA, et al. Management of hypertension and renin-angiotensin-aldosterone system blockade in adults with diabetic kidney disease: Association of British Clinical Diabetologists and the Renal Association UK guideline update 2021. BMC Nephrol 2022;23:9. [Crossref] [PubMed]
- Eisenhauer EA, Therasse P, Bogaerts J, et al. New response evaluation criteria in solid tumours: revised RECIST guideline (version 1.1). Eur J Cancer 2009;45:228-47. [Crossref] [PubMed]
- Levey AS, Coresh J, Greene T, et al. Using standardized serum creatinine values in the modification of diet in renal disease study equation for estimating glomerular filtration rate. Ann Intern Med 2006;145:247-54. [Crossref] [PubMed]
- Turin TC, Coresh J, Tonelli M, et al. Short-term change in kidney function and risk of end-stage renal disease. Nephrol Dial Transplant 2012;27:3835-43. [Crossref] [PubMed]
- Coresh J, Turin TC, Matsushita K, et al. Decline in estimated glomerular filtration rate and subsequent risk of end-stage renal disease and mortality. JAMA 2014;311:2518-31. [Crossref] [PubMed]
- Inker LA, Astor BC, Fox CH, et al. KDOQI US commentary on the 2012 KDIGO clinical practice guideline for the evaluation and management of CKD. Am J Kidney Dis 2014;63:713-35. [Crossref] [PubMed]
- Lai S, Amabile MI, Mazzaferro S, et al. Effects of sunitinib on endothelial dysfunction, metabolic changes, and cardiovascular risk indices in renal cell carcinoma. Cancer Med 2020;9:3752-7. [Crossref] [PubMed]
- Baek SH, Kim H, Lee J, et al. Renal adverse effects of sunitinib and its clinical significance: a single-center experience in Korea. Korean J Intern Med 2014;29:40-8. [Crossref] [PubMed]
- Pandey AK, Singhi EK, Arroyo JP, et al. Mechanisms of VEGF (Vascular Endothelial Growth Factor) Inhibitor-Associated Hypertension and Vascular Disease. Hypertension 2018;71:e1-8. [Crossref] [PubMed]
- Izzedine H, Ederhy S, Goldwasser F, et al. Management of hypertension in angiogenesis inhibitor-treated patients. Ann Oncol 2009;20:807-15. [Crossref] [PubMed]
- Robinson ES, Khankin EV, Karumanchi SA, et al. Hypertension induced by vascular endothelial growth factor signaling pathway inhibition: mechanisms and potential use as a biomarker. Semin Nephrol 2010;30:591-601. [Crossref] [PubMed]
- Sison K, Eremina V, Baelde H, et al. Glomerular structure and function require paracrine, not autocrine, VEGF-VEGFR-2 signaling. J Am Soc Nephrol 2010;21:1691-701. [Crossref] [PubMed]
- Nakagawa T. Uncoupling of the VEGF-endothelial nitric oxide axis in diabetic nephropathy: an explanation for the paradoxical effects of VEGF in renal disease. Am J Physiol Renal Physiol 2007;292:F1665-72. [Crossref] [PubMed]
- Valério P, Barreto JP, Ferreira H, et al. Thrombotic microangiopathy in oncology - a review. Transl Oncol 2021;14:101081. [Crossref] [PubMed]
- Paueksakon P, Revelo MP, Ma LJ, et al. Microangiopathic injury and augmented PAI-1 in human diabetic nephropathy. Kidney Int 2002;61:2142-8. [Crossref] [PubMed]
- Hernández-Arteaga K, Soto-Abraham V, Pérez-Navarro M, et al. Thrombotic microangiopathy in patients with diabetic nephropathy is associated with low VEGF expression and end-stage renal disease . Clin Nephrol 2018;89:429-37. [Crossref] [PubMed]
- Macfarlane R, Heng DY, Xie W, et al. The impact of kidney function on the outcome of metastatic renal cell carcinoma patients treated with vascular endothelial growth factor-targeted therapy. Cancer 2012;118:365-70. [Crossref] [PubMed]