Optimizing prostate cancer detection in transition zone: an analysis of apparent diffusion coefficient values in prostate magnetic resonance imaging evaluation with Prostate Imaging Reporting and Data System (PI-RADS) assessment
Highlight box
Key findings
• The minimum apparent diffusion coefficient (ADC) value significantly improves the detection of clinically significant prostate cancer (csPCa) in the transition zone (TZ) among patients with Prostate Imaging Reporting and Data System (PI-RADS) 3–5 lesions, with a higher csPCa detection rate (59.2%) observed for patients with a minimum ADC value below 561 µm2/s compared to the overall rate (33.7%) among PI-RADS 3–5 lesions.
What is known and what is new?
• Prostate multiparametric magnetic resonance imaging using PI-RADS scoring is recommended for csPCa detection.
• ADC values have been studied in csPCa diagnosis, with varying results, especially in the TZ.
• This study emphasizes the importance of the minimum ADC value for enhancing csPCa detection in TZ lesions, addressing a critical gap in current diagnostic methods and refining lesion identification and biopsy decisions.
What is the implication, and what should change now?
• Routine assessment of minimum ADC values should be considered in clinical practice for evaluating PI-RADS 3–5 lesions to aid in accurately identifying suspicious lesions and guiding biopsy decisions.
• Clinicians should integrate minimum ADC value assessment into their diagnostic protocols for evaluating PI-RADS 3–5 lesions to potentially reduce unnecessary biopsies and enhance the efficiency of prostate cancer diagnosis and management.
Introduction
Prostate cancer (PCa) is one of the most common cancers worldwide, such that 1.41 million cases were diagnosed in 2020 (1). The National Comprehensive Cancer Network guidelines recommend multiparametric magnetic resonance imaging (mpMRI) as a pre-biopsy procedure for the early detection of clinically significant PCa (csPCa) (2). csPCa is defined by histopathological features of International Society of Urological Pathologists grade group (GG) ≥2, tumor volume of 0.5 cc, or extraprostatic extension at the time of radical prostatectomy (3,4). The primary goal of prostate mpMRI is identifying and localizing abnormalities associated with csPCa. The Prostate Imaging Reporting and Data System (PI-RADS), a five-point scoring system for the risk stratification of lesions detected on multiparametric imaging, was introduced to standardize the mpMRI evaluation (5). The mpMRI assessment using PI-RADS is based on T2-weighted imaging (T2WI), diffusion-weighted imaging (DWI), and dynamic contrast-enhanced imaging. DWI is the primary sequence used for evaluation of the peripheral zone (PZ). Conversely, T2WI is the most important sequence for detection and characterization of the transition zone (TZ). Additionally, dynamic contrast-enhanced imaging is used to facilitate assessment (6).
The detection rate of csPCa exhibits considerable variability, with rates ranging from 21.3% to 70.5% for PI-RADS 4 lesions and 35.6% to 95.0% for PI-RADS 5 lesions (7-11). Kurokawa et al. found an improvement in the csPCa detection rate using MRI-targeted/transrectal ultrasound (TRUS) fusion prostate biopsy (MRI-FBx) (12). However, the csPCa detection rates remained relatively low for PI-RADS 3 (24.3%) and PI-RADS 4 (67.1%). Additionally, interobserver agreement is lower for TZ lesions than for PZ lesions, indicating the inherent limitations of imaging techniques (13). Despite these challenges, several studies have demonstrated that the apparent diffusion coefficient (ADC) can effectively differentiate various types of cancer (8,9). In particular, ADC values are significantly lower in PCa tissues than in normal tissues within the TZ, suggesting that ADC values can be used to distinguish between benign and malignant lesions (3). However, the role of ADC values in identifying PCa in the TZ remains uncertain, due to conflicting results in previous studies (14-18). Based on these considerations, we investigated the associations between ADC values in PI-RADS 3–5 lesions and csPCa in TZ lesions, with the goal of addressing a substantial gap in current diagnostic methodologies. We present this article in accordance with the STROBE reporting checklist (available at https://tau.amegroups.com/article/view/10.21037/tau-24-235/rc).
Methods
Study design
This study is a cross-sectional study designed to evaluate the relationship between ADC values and csPCa in lesions of the TZ detected using mpMRI.
Patient demographics
We analyzed the medical records of 90 patients with PI-RADS 3–5 lesions who underwent MRI-FBx between September 2019 and March 2023 at a single academic medical center (The Jikei University Hospital). mpMRI was performed for individuals with a high serum prostate-specific antigen (PSA) level or abnormal findings during digital rectal examination. Based on the MRI findings, patients with potentially cancerous lesions (PI-RADS version v2.1 categories 3–5) underwent MRI-FBx and a systematic core biopsy.
Figure 1 presents a flowchart of patient selection. We selected 220 patients who had undergone FBx at The Jikei University Hospital between September 2019 and March 2023. In total, 130 patients who underwent MRI-FBx of the PZ were excluded. Additionally, five patients who underwent 1.5 T mpMRI and two patients who used non-designated MRI models were excluded, resulting in a sample size of 83 individuals and 243 sites. If csPCa and non-csPCa were identified in the same target lesion, only the pathological diagnosis of csPCa was documented. Finally, the analysis included 196 lesions. The primary endpoint of this study was the csPCa detection rate in targeted biopsy specimens of PI-RADS 3–5 lesions. In this study, csPCa was defined as GG ≥2. The study was conducted in accordance with the Declaration of Helsinki (as revised in 2013). The study was approved by The Jikei University Institutional Review Board [No. 33-260(10878)], and individual consent for this retrospective analysis was waived.
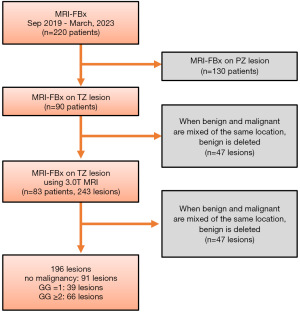
MRI
Patients were evaluated using 3.0 Tesla mpMRI (Siemens MAGNETOM series, Erlangen, Germany). The diagnostic protocol consisted of a simplified non-contrast biparametric approach with T2WI and DWI or a comprehensive clinical protocol including dynamic contrast-enhanced MRI. mpMRI images were interpreted by a radiologist who specialized in urological imaging.
Fusion biopsy
Software-based MRI-FBx followed by core systematic biopsies were obtained by a urologist using the transperineal approach under spinal anesthesia. Prior to the procedure, MRI-FBx was planned using T2WI and dedicated Biojet software. The planning images were combined with real-time TRUS images to identify areas of potential malignancy. Up to three core biopsy samples were obtained from each targeted lesion using an end-fire ultrasound probe. The samples were analyzed by two genitourinary pathologists.
DWI analysis
In areas with PI-RADS 3 or higher, a region of interest (ROI) corresponding to the inner tumor margin was drawn in a freehand manner on the ADC map generated from DWI with b values of 2,000 s/mm2, particularly on the image with the largest tumor size. Subsequently, maximum, minimum, and mean ADC values were automatically measured. All tumor ROIs were drawn on the ADC map by a reader who was blinded to the GG and other pathological information.
Statistical analysis
Statistical analyses were conducted using two-sided tests with a threshold for statistical significance set at P<0.05. Data were analyzed using R software (version 4.0.3; R Foundation for Statistical Computing, Vienna, Austria). Unpaired t-tests were used to compare the two groups. Logistic regression analysis was utilized to identify independent predictors of csPCa.
Potential confounders, such as age, initial PSA (iPSA) level, and ROI area, were adjusted for in the multivariate logistic regression model. Specifically, variables that were significant in the univariate analysis (P<0.05) were included in the multivariate analysis to control for their potential confounding effects.
Regarding missing data, any data points that were missing were excluded from the analysis. This approach ensured that the dataset remained consistent and that the statistical analyses were conducted on complete cases only.
To detect csPCa in the TZ, an optimal cutoff value was determined based on receiver operating characteristic (ROC) curve analysis. Using a data-driven approach, we selected the cutoff point nearest the upper left-hand corner of the ROC curve. Sensitivity and specificity were calculated for the determined cutoff value.
The statistical power of the study was evaluated post hoc based on the sample size and the effect sizes observed. With a sample size of 196 lesions, the study was adequately powered to detect significant differences in ADC values between csPCa and non-csPCa groups.
Results
Table 1 presents the patient characteristics and MRI findings. The overall detection rate for PCa was 53.6% (105/196), and the detection rate for csPCa was 33.7% (66/196). The presence of csPCa was significantly associated with older age, a higher iPSA level, and a larger ROI area. Moreover, in patients diagnosed with csPCa, the minimum and mean ADC values were 484.9±112.3 and 740.3±123.9 µm2/s, respectively. These values were significantly lower than the values for patients diagnosed with benign diseases or non-csPCa, in whom they were 707.3±414.4 and 919.9±237.2 µm2/s, respectively. These findings may suggest that lower ADC values are indicative of higher cellular density and reduced extracellular space, characteristic of malignant tissues.
Table 1
Variables | Benign/ncsPC | csPC | std diff |
---|---|---|---|
Number of patients | 130 | 66 | |
Age (years), mean (SD) | 67.8 (7.2) | 69.8 (5.7) | 0.043 |
Initial PSA (ng/mL), mean (SD) | 7.7 (4.1) | 9.85 (5.3) | 0.002 |
Total prostate volume (mL), mean (SD) | 45.2 (19.8) | 47.75 (26.7) | 0.45 |
PI-RADS score, n (%) | – | ||
3 | 19 (14.6) | 8 (12.1) | |
4 | 87 (66.9) | 34 (51.5) | |
5 | 24 (18.5) | 24 (36.4) | |
ROI area (mm2), mean (SD) | 88.7 (102.8) | 153.32 (176.6) | 0.001 |
ADC value (μm2/s) | |||
Max (SD) | 1,392.1 (342.2) | 1,278.65 (302.2) | 0.02 |
Min (SD) | 707.3 (414.4) | 484.9 (112.3) | <0.001 |
Mean (SD) | 919.9 (237.2) | 740.3 (123.9) | <0.001 |
Grade group, n (%) | – | ||
Benign | 91 (70.0) | – | |
1 | 39 (30.0) | – | |
2 | – | 43 (65.2) | |
3 | – | 16 (24.2) | |
4 | – | 7 (10.6) |
ncsPC, non-csPC; csPC, clinically significant prostate cancer; std diff, standardised difference; SD, standard deviation; PSA, prostate-specific antigen; PI-RASDS, Prostate Imaging Reporting and Data System; ROI, region of interest; ADC, apparent diffusion coefficient; Max, maximum; Min, minimum.
Table 2 presents the univariate and multivariate analyses of predictors for csPCa. Univariate analysis revealed that age (P=0.04), iPSA (P=0.004), ROI area (P=0.01), and maximum (P=0.03), minimum (P<0.001), and mean (P<0.001) ADC values were significantly associated with csPCa. Multivariate analysis demonstrated that ROI area (P=0.01), minimum ADC value (P<0.001), and mean ADC value (P<0.001) were independent predictors of csPCa in patients with PI-RADS 3–5 lesions (Table S1).
Table 2
Variables | Univariate analysis | Multivariate analysis | |||
---|---|---|---|---|---|
Odds ratio (95% CI) | P value | Odds ratio (95% CI) | P value | ||
Age | 1.050 (1.000–1.100) | 0.04 | 1.050 (0.988–1.100) | 0.12 | |
Initial PSA | 1.110 (1.030–1.180) | 0.004 | 1.070 (0.993–1.150) | 0.08 | |
Total prostate volume | 1.010 (0.992–1.020) | 0.45 | – | – | |
ROI area | 1.010 (1.000–1.010) | 0.01 | 1.000 (0.999–1.010) | 0.01 | |
ADC value | |||||
Max | 0.999 (0.998–1.000) | 0.03 | – | – | |
Min | 0.994 (0.991–0.996) | <0.001 | 0.994 (0.992–0.997) | <0.001 | |
Mean | 0.994 (0.991–0.996) | <0.001 | – | – |
CI, confidence interval; PSA, prostate-specific antigen; ROI, region of interest; ADC, apparent diffusion coefficient; Max, maximum; Min, minimum.
Figure 2 presents the ROC curve of the minimum ADC value for predicting csPCa. The area under the ROC curve for the minimum ADC value was 0.778 [95% confidence interval (CI): 0.713–0.844]. The optimal cutoff value for the minimum ADC was 561 µm2/s for predicting the presence of PI-RADS 3–5 csPCa. Additionally, the ROC curve for the mean ADC value for predicting csPCa revealed an area under the curve of 0.761 (95% CI: 0.694–0.828) (Figure S1). When patients were divided into two groups based on the minimum ADC value of 561 µm2/s, 94 had lesions with a minimum ADC <561 µm2/s and 102 had lesions with a minimum ADC ≥561 µm2/s. Table 3 presents the biopsy findings in the two groups. The detection rate of csPCa among the 94 patients with an ADC <561 µm2/s was 57.4% (54/94), which was higher than the rate for all 196 patients with PI-RADS 3–5 lesions (33.7%).
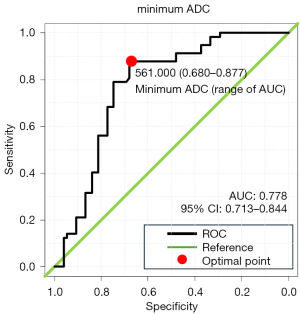
Table 3
Minimum ADC category | ncsPC | csPC | Total |
---|---|---|---|
Minimum ADC <561 μm2/s | 40 | 54 | 94 |
Minimum ADC ≥561 μm2/s | 90 | 12 | 102 |
Total | 130 | 66 | 196 |
ADC, apparent diffusion coefficient; ncsPC, non-csPC; csPC, clinically significant prostate cancer.
Table 3 presents the biopsy findings based on the minimum ADC value cutoff of 561 µm2/s. Among the 94 patients with an ADC <561 µm2/s, the csPCa detection rate was 57.4% (54/94), which was higher than the overall detection rate of 33.7% among all 196 patients with PI-RADS 3–5 lesions. Conversely, among the 102 patients with an ADC ≥561 µm2/s, the csPCa detection rate was 11.8% (12/102), suggesting that 90 (88.2%) of these patients could potentially avoid unnecessary prostate biopsies.
Discussion
In this study, we investigated the clinical utility of ADC values in terms of evaluating PI-RADS 3–5 lesions and csPCa in TZ lesions. We found that the minimum ADC value exhibited utility in the detection of csPCa. Previous studies have suggested that ADC values can be used to minimize false-positive results in PI-RADS assessments (16,18-20). Wu et al. demonstrated that the minimum ADC value of a tumor was able to distinguish between Gleason scores 3+4 and 3+3 (21). However, they evaluated ADC values for the entire prostate without differentiating between prostate zones. It is important to distinguish between the PZ and TZ because the ADC values of benign and malignant lesions in the TZ are generally lower than those values in the PZ (22,23). Recently, Ono et al. found that the minimum ADC value improved the detection rate of csPCa in the TZ (24). Our findings also suggest that a lower minimum ADC value can improve the detection of csPCa. In contrast to a previous study, which utilized cognitive fusion biopsy, we used the Biojet procedure for fusion biopsy. Differences among biopsy techniques can greatly influence the accuracy of pathological tissue sampling. Indeed, the specificity in our study was 88%, compared with 83% in the work of Ono et al. (24), which may be related to the diagnostic power of the biopsy technique. Furthermore, in our study, the GG was assessed by two genitourinary pathologists (S.S. and H.T), which may have improved the detection of csPCa. A notable strength of the present study was that we demonstrated the importance of the minimum ADC value, despite using a more accurate biopsy technique. However, it is challenging to compare the cutoff values between the present study and the study performed by Ono et al.; additional cases are needed (24).
Our study also considered the issue of false-positive results and the reproducibility of our methods. While the minimum ADC value proved useful in differentiating between benign and malignant lesions, there remains a possibility of false positives. To mitigate this, we employed the Biojet procedure for fusion biopsy and had samples reviewed by experienced genitourinary pathologists. However, false positives can still occur due to inherent limitations in imaging and biopsy techniques.
Previous studies have demonstrated that the mean ADC value is useful for detection of csPCa in the TZ (25,26). However, these studies did not compare minimum and mean ADC values in the context of csPCa detection. Nevertheless, studies of liver and breast tumors have demonstrated that the minimum ADC value is more sensitive and specific than the mean ADC value (27,28). The minimum ADC value is less influenced by the manual delineation of target lesions, facilitating the identification of ROIs in a simple manner. Therefore, although the mean ADC value is a predictor of csPCa in the TZ region, we suggest routine evaluation of the minimum ADC value in clinical practice. This approach could provide a more robust and accurate method for the identification of csPCa, enhancing the accuracy and efficiency of PCa diagnosis and management.
Regarding the reproducibility of our methods, we acknowledge that manual delineation of ROIs can lead to variability in ADC measurements. To address this, the delineation was performed by a blinded reader who specialized in urogenital imaging, reducing subjective bias. Despite this, variability is an inherent challenge, and future studies should explore automated or semi-automated techniques to enhance reproducibility.
In total, 94 patients had lesions with a minimum ADC value <561 µm2/s. The detection rate for csPCa at this cutoff value was 57.4% (54/94), which was higher than the overall detection rate of 33.7% among the 196 patients with PI-RADS 3–5 lesions. Conversely, the remaining 102 patients had lesions with a minimum ADC value ≥561 µm2/s, and the csPCa detection rate was 11.8% (12/102), indicating that 90 (88.2%) of the 102 patients could potentially avoid unnecessary prostate biopsies. However, using this cutoff value, csPCa was missed in 12 (11.8%) of 102 patients. Therefore, further studies are needed to integrate the minimum ADC value with other markers. Nevertheless, in clinical practice, low ADC values may suggest areas of csPCa, providing patients and physicians with useful information before biopsy.
Although this study provided new insights regarding PI-RADS assessment for patients with csPCa in the TZ, it had some limitations. First, it was a retrospective study conducted at a single institution; thus, the generalizability of the results was limited due to the relatively small sample size. Second, we could not exclude the possibility of false-positive results in the PI-RADS assessment or sampling errors during MRI-FBx. Although a radiologist with expertise in urogenital imaging performed the PI-RADS assessments and conducted MRI-FBx for lesions rated PI-RADS 3–5, sampling errors were possible. Finally, the ROIs were manually delineated, potentially resulting in low reproducibility due to the freehand method used.
In conclusion, the minimum ADC value enhances the detection of csPCa in the TZ, which could enhance the detection rates of potentially cancerous lesions, particularly those rated as PI-RADS 3–5. Additionally, it provides valuable information related to the need for follow-up biopsies in areas that are initially cancer-free. Our findings will contribute to the advancement of diagnostic imaging techniques for csPCa in the TZ, potentially reducing unnecessary needle biopsies.
Conclusions
The minimum ADC value has demonstrated added value in indicating the presence of csPCa in the TZ. This finding could enhance the detection rates of lesions deemed suspicious for cancer, particularly those rated as PI-RADS 3 or higher. Additionally, it may provide valuable guidance on whether to conduct follow-up biopsies in areas initially deemed negative. We anticipate that these insights will contribute to the advancement of imaging diagnostic capabilities for csPCa in the TZ, potentially leading to a decrease in unnecessary prostate needle biopsies. The findings from this study suggest that incorporating minimum ADC values into clinical practice can provide clinicians with valuable pre-biopsy information, potentially improving diagnostic accuracy and patient outcomes. Future research should focus on validating these results in larger, multicenter studies and exploring automated methods for ADC measurement to enhance reproducibility and scalability in clinical settings. By doing so, we can advance the standardization of MRI evaluation protocols and optimize PCa diagnostic pathways.
Acknowledgments
The authors thank Textcheck for performing English language editing of this manuscript.
Funding: None.
Footnote
Reporting Checklist: The authors have completed the STROBE reporting checklist. Available at https://tau.amegroups.com/article/view/10.21037/tau-24-235/rc
Data Sharing Statement: Available at https://tau.amegroups.com/article/view/10.21037/tau-24-235/dss
Peer Review File: Available at https://tau.amegroups.com/article/view/10.21037/tau-24-235/prf
Conflicts of Interest: All authors have completed the ICMJE uniform disclosure form (available at https://tau.amegroups.com/article/view/10.21037/tau-24-235/coif). The authors have no conflicts of interest to declare.
Ethical Statement: The authors are accountable for all aspects of the work in ensuring that questions related to the accuracy or integrity of any part of the work are appropriately investigated and resolved. The study was conducted in accordance with the Declaration of Helsinki (as revised in 2013). The study was approved by the Jikei University Institutional Review Board [No. 33-260(10878)], and individual consent for this retrospective analysis was waived.
Open Access Statement: This is an Open Access article distributed in accordance with the Creative Commons Attribution-NonCommercial-NoDerivs 4.0 International License (CC BY-NC-ND 4.0), which permits the non-commercial replication and distribution of the article with the strict proviso that no changes or edits are made and the original work is properly cited (including links to both the formal publication through the relevant DOI and the license). See: https://creativecommons.org/licenses/by-nc-nd/4.0/.
References
- Thornbury JR, Ornstein DK, Choyke PL, et al. Prostate cancer: what is the future role for imaging? AJR Am J Roentgenol 2001;176:17-22. [Crossref] [PubMed]
- Dickinson L, Ahmed HU, Allen C, et al. Magnetic resonance imaging for the detection, localisation, and characterisation of prostate cancer: recommendations from a European consensus meeting. Eur Urol 2011;59:477-94. [Crossref] [PubMed]
- Barentsz JO, Choyke PL, Cornud F, et al. Reply to Erik Rud and Eduard Baco's Letter to the Editor re: Re: Jeffrey C. Weinreb, Jelle O. Barentsz, Peter L. Choyke, et al. PI-RADS Prostate Imaging - Reporting and Data System: 2015, Version 2. Eur Urol 2016;69:16-40. Eur Urol 2016;70:e137-8. [Crossref] [PubMed]
- Weinreb JC, Barentsz JO, Choyke PL, et al. PI-RADS Prostate Imaging - Reporting and Data System: 2015, Version 2. Eur Urol 2016;69:16-40. [Crossref] [PubMed]
- Barentsz JO, Richenberg J, Clements R, et al. ESUR prostate MR guidelines 2012. Eur Radiol 2012;22:746-57. [Crossref] [PubMed]
- Arumainayagam N, Ahmed HU, Moore CM, et al. Multiparametric MR imaging for detection of clinically significant prostate cancer: a validation cohort study with transperineal template prostate mapping as the reference standard. Radiology 2013;268:761-9. [Crossref] [PubMed]
- Cash H, Maxeiner A, Stephan C, et al. The detection of significant prostate cancer is correlated with the Prostate Imaging Reporting and Data System (PI-RADS) in MRI/transrectal ultrasound fusion biopsy. World J Urol 2016;34:525-32. [Crossref] [PubMed]
- Mertan FV, Greer MD, Shih JH, et al. Prospective Evaluation of the Prostate Imaging Reporting and Data System Version 2 for Prostate Cancer Detection. J Urol 2016;196:690-6. [Crossref] [PubMed]
- Greer MD, Shih JH, Lay N, et al. Validation of the Dominant Sequence Paradigm and Role of Dynamic Contrast-enhanced Imaging in PI-RADS Version 2. Radiology 2017;285:859-69. [Crossref] [PubMed]
- John S, Cooper S, Breau RH, et al. Multiparametric magnetic resonance imaging - Transrectal ultrasound-guided cognitive fusion biopsy of the prostate: Clinically significant cancer detection rates stratified by the Prostate Imaging and Data Reporting System version 2 assessment category. Can Urol Assoc J 2018;12:401-6. [Crossref] [PubMed]
- Westphalen AC, McCulloch CE, Anaokar JM, et al. Variability of the Positive Predictive Value of PI-RADS for Prostate MRI across 26 Centers: Experience of the Society of Abdominal Radiology Prostate Cancer Disease-focused Panel. Radiology 2020;296:76-84. [Crossref] [PubMed]
- Kurokawa G, Mori K, Sasaki H, et al. Effectiveness of Magnetic Resonance Imaging/Ultrasound-guided Target Biopsy in Detecting Clinically Significant Prostate Cancer. Anticancer Res 2024;44:679-86. [Crossref] [PubMed]
- Rosenkrantz AB, Ginocchio LA, Cornfeld D, et al. Interobserver Reproducibility of the PI-RADS Version 2 Lexicon: A Multicenter Study of Six Experienced Prostate Radiologists. Radiology 2016;280:793-804. [Crossref] [PubMed]
- Kim E, Kim CK, Kim HS, et al. Histogram analysis from stretched exponential model on diffusion-weighted imaging: evaluation of clinically significant prostate cancer. Br J Radiol 2020;93:20190757. [Crossref] [PubMed]
- Hauth E, Halbritter D, Jaeger H, et al. Diagnostic value of semi-quantitative and quantitative analysis of functional parameters in multiparametric MRI of the prostate. Br J Radiol 2017;90:20170067. [Crossref] [PubMed]
- Moraes MO, Roman DHH, Copetti J, et al. Effects of the addition of quantitative apparent diffusion coefficient data on the diagnostic performance of the PI-RADS v2 scoring system to detect clinically significant prostate cancer. World J Urol 2020;38:981-91. [Crossref] [PubMed]
- Westphalen AC, Fazel F, Nguyen H, et al. Detection of clinically signifi cant prostate cancer with PIRADS v2 scores, PSA density, and ADC values in regions with and without mpMRI visible lesions. Int Braz J Urol 2019;45:713-23. [Crossref] [PubMed]
- Pepe P, D’Urso D, Garufi A, et al. Multiparametric MRI Apparent Diffusion Coefficient (ADC) Accuracy in Diagnosing Clinically Significant Prostate Cancer. In Vivo 2017;31:415-8. [Crossref] [PubMed]
- Kim TH, Kim CK, Park BK, et al. Relationship between Gleason score and apparent diffusion coefficients of diffusion-weighted magnetic resonance imaging in prostate cancer patients. Can Urol Assoc J 2016;10:E377-82. [Crossref] [PubMed]
- Meyer HJ, Wienke A, Surov A. Discrimination between clinical significant and insignificant prostate cancer with apparent diffusion coefficient - a systematic review and meta analysis. BMC Cancer 2020;20:482. [Crossref] [PubMed]
- Wu X, Reinikainen P, Vanhanen A, et al. Correlation between apparent diffusion coefficient value on diffusion-weighted MR imaging and Gleason score in prostate cancer. Diagn Interv Imaging 2017;98:63-71. [Crossref] [PubMed]
- Yoshizako T, Wada A, Hayashi T, et al. Usefulness of diffusion-weighted imaging and dynamic contrast-enhanced magnetic resonance imaging in the diagnosis of prostate transition-zone cancer. Acta Radiol 2008;49:1207-13. [Crossref] [PubMed]
- Gaur S, Harmon S, Rosenblum L, et al. Can Apparent Diffusion Coefficient Values Assist PI-RADS Version 2 DWI Scoring? A Correlation Study Using the PI-RADSv2 and International Society of Urological Pathology Systems. AJR Am J Roentgenol 2018;211:W33-41. [Crossref] [PubMed]
- Ono A, Hashimoto T, Shishido T, et al. Clinical value of minimum apparent diffusion coefficient for prediction of clinically significant prostate cancer in the transition zone. Int J Clin Oncol 2023;28:716-23. [Crossref] [PubMed]
- Chatterjee A, Gallan AJ, He D, et al. Revisiting quantitative multi-parametric MRI of benign prostatic hyperplasia and its differentiation from transition zone cancer. Abdom Radiol (NY) 2019;44:2233-43. [Crossref] [PubMed]
- Bajgiran AM, Mirak SA, Sung K, et al. Apparent Diffusion Coefficient (ADC) Ratio Versus Conventional ADC for Detecting Clinically Significant Prostate Cancer With 3-T MRI. AJR Am J Roentgenol 2019;213:W134-42. [Crossref] [PubMed]
- Hirano M, Satake H, Ishigaki S, et al. Diffusion-weighted imaging of breast masses: comparison of diagnostic performance using various apparent diffusion coefficient parameters. AJR Am J Roentgenol 2012;198:717-22. [Crossref] [PubMed]
- Namimoto T, Nakagawa M, Kizaki Y, et al. Characterization of Liver Tumors by Diffusion-Weighted Imaging: Comparison of Diagnostic Performance Using the Mean and Minimum Apparent Diffusion Coefficient. J Comput Assist Tomogr 2015;39:453-61. [Crossref] [PubMed]