TRIM47 promotes the Warburg effect and reduces ferroptosis in prostate cancer by FBP1 and FOXO1
Highlight box
Key findings
• Tripartite motif 47 (TRIM47) promotes the Warburg effect in prostate cancer (PC) by increasing fructose-1,6-bisphosphatase 1 (FBP1) and forkhead box protein O1 (FOXO1).
What is known, and what is new?
• TRIM47 promotes cell proliferation in ovarian cancer.
• Targeting TRIM47 could potentially serve as a viable therapeutic strategy for the treatment of PC.
What is the implication, and what should change now?
• TRIM47 might benefit the treatment of PC at various stages of tumor progression.
Introduction
Prostate cancer (PC), a malignancy arising in the male prostate tissue, has a high incidence rate. It accounts for 14% of all tumor cases, and is common in middle-aged and elderly men (1). Early-stage PC typically presents without discernible clinical symptoms (2). With the development of the disease, its main manifestations include increased urinary frequency and urgency, and dysuria, which have a certain impact on the life of patients (3).
Radical prostatectomy is the prevailing clinical intervention for PC management (4). It has a good therapeutic effect; however, it can cause adverse events, such as urinary incontinence and abnormal urinary control function, which affect the work and life of patients (5).
PC has emerged as a significant public health concern, and poses a threat to human wellbeing (5). Regrettably, due to the lack of emphasis on the early screening of PC, it is often diagnosed at an advanced or metastatic stage (6). Despite the diligent application of androgen deprivation therapy, patients with PC may develop treatment resistance, culminating in the manifestation of castration-resistant PC (6).
The Warburg effect is a phenomenon observed in cancer cells where they preferentially undergo glycolysis, a process that produces energy in the form of adenosine triphosphate (ATP) through the breakdown of glucose, even in the presence of sufficient oxygen. This is in contrast to normal cells, which mainly rely on oxidative phosphorylation to generate ATP under aerobic conditions. The Warburg effect was first described by Otto Warburg in the 1920s and is considered one of the hallmarks of cancer. It is believed to occur due to several factors, including genetic mutations, alterations in metabolic enzymes, and changes in the tumor microenvironment.
The Warburg effect is a subject of considerable controversy; however, its significance in clinical tumor diagnosis, and innovative progress in related theories have renewed interest in the role of the Warburg effect in tumor development and treatment in PC (6,7). The rapid proliferation of tumor cells necessitates high glucose and oxygen consumption, resulting in relative nutrient depletion, and the creation of a hypoxic microenvironment. Physicochemical conditions, such as low oxygen levels, acidic potential hydrogen (pH), and nutrient scarcity, favor tumor cell proliferation (8-10).
Currently, research on ferroptosis in PC in China is limited, and most studies have focused on the cytotoxic effects of ferroptosis inducers in PC cells (11,12). Thus, the pathogenesis of PC requires further investigation. The induction of ferroptosis is a promising anti-cancer strategy, and it provides new therapeutic targets (13-15). Calcium ion homeostasis is closely linked to proliferation, migration, apoptosis, drug resistance, and associated autophagy pathways in PC cells.
Cancer cells mainly obtain energy through aerobic glycolysis (16). Gluconeogenesis and glycolysis are two nearly opposite processes in PC. Inhibiting fructose-1,6-bisphosphatase 1 (FBP1) may potentially suppress the Warburg effect (17). Therefore, research on FBP1 in cancer is highly valued (18). Research has revealed that the mechanism of FBP1 in cancer inhibition may involve blocking the cell cycle (19). FBP1 is localized in the cytoplasm, nucleus, and extracellular vesicles. Gene Ontology analysis has indicated that the molecular functions of FBP1 include adenine nucleotide binding. As a pivotal enzyme in gluconeogenesis, FBP1 controls the rate of glucose production in the liver and kidneys. It is ubiquitously expressed across various prokaryotic and eukaryotic organisms with the liver and kidneys serving as the primary sites of expression in mammals (19).
Forkhead box proteins (FOXOs) participate in the β-catenin signaling pathway, while other molecules are involved in cell growth, apoptosis, and antioxidant defense, which mediate the cellular stress response in PC (20). Phosphorylated protein kinase B (Akt) inhibits the transcriptional activity of forkhead box protein O1 (FOXO1), reducing its regulation of key enzymes in gluconeogenesis, thus regulating glycogen synthesis, glycogen breakdown, and gluconeogenesis (20-22). The functional state of osteoblasts in the bone marrow microenvironment is crucial for maintaining the normal expression of hematopoietic stem cells, and the up-regulation of FOXO1 expression can disrupt osteoblast function and involve downstream cells, leading to the occurrence of precancerous states (23).
The tripartite motif (TRIM) family consists of numerous members with diverse and complex functions (24,25). These members can mediate pancreatic cancer proliferation, invasion, epithelial mesenchymal transition, and resistance to chemotherapy drugs through the ubiquitination of cancer proteins and tumor-related factors, post-transcriptional regulation, cellular glycolysis metabolism, and the phosphatidylinositol-4,5-bisphosphate 3-kinase (PI3K)/AKT/mammalian target of rapamycin (mTOR) signaling pathway in PC (26,27). Wu et al. showed that the over-expression of TRIM44 increased ferroptosis (28). Liu et al. reported that TRIM59 mediated ferroptosis in neuroblastoma therapy (29). Zhang et al. reported that TRIM59 promoted ferroptosis in non-alcoholic fatty liver disease (30). Liu et al. showed that TRIM22 inhibited osteosarcoma progression through the Warburg effect (31). TRIM47 is stably activated through protein kinase C-e/protein kinase D3/nuclear factor-kappa B signaling, and contributes to endocrine therapy of drug-resistant PC patients (32). Thus, TRIM47 might regulate ferroptosis or the Warburg effect in PC.
In this article, we explored the role of TRIM47 in the progression of PC and its underlying mechanism. A protocol was prepared before the study, which was not registered. We present this article in accordance with the MDAR and ARRIVE reporting checklists (available at https://tau.amegroups.com/article/view/10.21037/tau-23-605/rc).
Methods
Clinical samples of PC
The study was conducted in accordance with the Declaration of Helsinki (as revised in 2013). All the subjects signed informed consent forms. This study was approved by the Ethics Committee of Shenzhen People’s Hospital (No. 20180815017).
The pathological evaluation was performed according to the classification system of the World Health Organization. Paracancerous tissue (>3 cm from the cancer tissue) was acquired and served as the control tissue. The survival analysis was performed using the Kaplan-Meier method, and the log-rank test was used to examine the survival curves.
Cell culture and transfection
A normal cell line (RwPE-2, the control), and human PC cell lines (LNCaP, PC-3, DU145, and LAPC-4) were treated in accordance with the American Type Culture Collection (ATCC) protocols and incubated in an atmosphere of 5% carbon dioxide at 37 °C. TRIM47 (sc-432202, Santa Cruz Biotechnology, Shanghai, China) or small interfering (si)-TRIM47 (sc-93720, Santa Cruz Biotechnology) plasmids were transfected into the cells using Lipofectamine 2000 (Thermo Fisher Scientific Inc., Shanghai, China).
Quantitative polymerase chain reaction (qPCR)
Total RNAs were isolated with total RNA extraction reagent (Takara, Dalian, China) and complementary DNA was synthesized using the PrimeScipt RT Master Mix (Takara). qPCR was performed using the ABI Prism 7500 sequence detection system (Applied Bio-systems, Shanghai, China). Relative expression levels of the sample messenger RNA (mRNA) were calculated, and expressed as 2−ΔΔCt. The primers used in this study: Actb-F: 5'-TGTTACCAACTGGGACGACA-3', Actb-R: 5'-CTGGGTCATCTTTTCACGGT-3'; Trim47-F: 5'-GGTAAACACAGTCGCTAAGAGGTCAAA-3', Trim47-R: 5'-TGGTCTAGGGATGCCAGGGTTCT-3'.
Bioluminescence imaging
All the experiments related to animals were approved by the Animal Care and Use Committee of Shenzhen People’s Hospital (No. 20210814M14) and were performed in compliance with the national guidelines for the care and use of animals. The LNCaP-hFBP1P-Luc transgenic mice and the LNCaP-h FOXO1-Luc transgenic mice were treated as previously described (15,33). Bioluminescent imaging was performed using an invasive live imaging system (IVIS) imaging system (Bio-Real, QuickView3000, Austria).
Immunofluorescent staining
The cells were fixed with 4% paraformaldehyde for 15 min and incubated with 0.15% Triton X‑100 for 15 min at room temperature. The cells were incubated with TRIM47 (1:500, Abcam, Shanghai, China), FBP1 (1:500, Cell Signaling Technology, Inc., Shanghai, China), FOXO1 (1:500, Abcam) at 4 °C overnight after blocking with 5% bovine serum albumin (BSA) for 1 h. The cells were incubated with goat anti-rabbit IgG-cFL 488 or anti-rabbit IgG-cFL 555 antibody (1:100, HA1117) for 2 h at room temperature, stained with 4',6-diamidino-2-phenylindole (DAPI) for 15 min, and washed with phosphate buffer solution for 15 min. Images of the cells were obtained using a Zeiss Axioplan 2 fluorescent microscope (Carl Zeiss AG, Oberkochen, Germany).
The extracellular acidification rate (ECAR), oxygen consumption rate (OCR), glucose consumption rate, lactate production level, and ATP level were measured. The ECAR and OCR were determined using a Seahorse XFe96 analyzer (Seahorse Bioscience, Agilent, Shanghai, China). The glucose consumption rate was determined using a glucose assay kit (Sigma, St-Louis, MO, USA). The lactate level was measured using a lactate colorimetric/fluorometric assay kit (BioVision, Mountain View, CA, USA) The ATP level was determined using an ATP determination kit (Thermo Fisher Scientific, Waltham, MA, USA).
Proliferation assays and ethynyl deoxyuridine (EdU) staining
After 48 h of transfection, approximately 2×103 cells/well were seeded in a 96-well plate. After culturing for the indicated time, cellular proliferation was detected using cell counting kit-8 (CCK-8) in accordance with the manufacturer’s instructions (C0037, Beyotime, Nanjing, China).
EdU (10 mM) was added to each well, and the cells were fixed with 4% formaldehyde for 30 min. After washing, EdU was detected using an EdU Kit, and the images were visualized by fluorescent microscopy (Olympus, Shanghai, China).
Transwell migration assays
The migration assays were performed using the Costar Transwell Invasion chamber (Merck KgaA, Shanghai, China) in accordance with the manufacturer’s instructions (34,35). Cells (2×104) were added to the upper chamber in a volume of serum-free Dulbecco’s modified Eagle medium (DMEM), and the invasion assays were performed using Millicell cell culture inserts (Millipore, Shanghai, China). Images were visualized using fluorescent microscope (Olympus).
Western blot
Total proteins were separated by sodium dodecyl sulfate polyacrylamide gel electrophoresis (SDS-PAGE) and transferred onto polyvinylidene difluoride membranes. After blocking with 5% BSA in tris buffered saline (TBS), the membranes were incubated with the following primary antibodies: TRIM47 (ab72234, 1:2,000, Abcam), FBP1 (ab109732, 1:2,000, Abcam), FOXO1 (ab312326, 1:2,000, Abcam), glutathione peroxidase 4 (GPX4) (ab125066, 1:2,000, Abcam), and β-Actin (GB11001-100, 1:5,000, Servicebio, Wuhan, China). The membranes were then incubated with peroxidase-conjugated secondary antibodies (GB23303, 1:5,000, Servicebio). The signals were detected with the ECL system and exposed using the ChemiDoc XRS system with Image Labsoftware (Bio-rad, Shanghai, China).
Statistical analysis
Graphad Prism 6 (GraphPad Software) was used for the statistical analysis. A P value <0.05 was considered statistically significant. Data between groups were compared using the Student’s t-test or, a one-way analysis of variance, followed by Tukey’s post-hoc test.
Results
TRIM47 expression in PC model
The expression of the TRIM47 mRNA in patients with PC or PC cells was up-regulated (Figure 1A,1B). The expression of the TRIM47 protein in patients with PC or PC cells was also increased (Figure 1C,1D). The survival analysis revealed that PC patients with high expression levels of TRIM47 had poorer survival rates than those with low expression levels (Figure 1E,1F). Taken together, these findings suggest that the TRIM47 gene plays an activator role in PC.
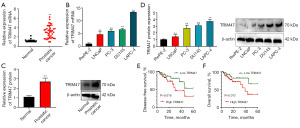
The TRIM47 gene promoted cell growth in PC cells
This study examined the role of TRIM47 in cell growth in PC cells. As Figure 1 shows, the expression level of TRIM47 was the lowest in the LNCaP cells, and the highest in the LAPC-4 cells. TRIM47 plasmid was transfected into the LNCaP cells, and the LNCaP cells were used to conduct the relevant experiments. Si-TRIM47 plasmid was transfected into the LAPC-4 cells. The over-expression of TRIM47 increased the expression of TRIM47 mRNA, promoted cell growth and migration, and facilitated 5-Ethynyl-2'-deoxyuridine (Edu)-positivity in the in vitro model (Figure 2A-2D). In contrast, the knockdown of TRIM47 decreased the expression of TRIM47 mRNA, reduced cell growth and migration, and reduced Edu-positivity in the in vitro model (Figure 2E-2H).
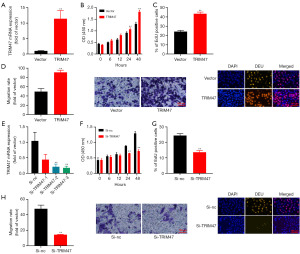
The TRIM47 gene promoted the Warburg effect in PC cells
Next, the up-regulation of TRIM47 elevated glucose consumption, lactate production, and ATP levels in the PC cells (Figure 3A-3C). Conversely, the down-regulation of TRIM47 decreased glucose consumption, lactate production, and ATP quantity in the PC cells (Figure 3A-3C). Moreover, the up-regulation of TRIM47 promoted the ECAR, and reduced mitochondrial respiratory capacity in the PC cells (Figure 3D,3E). Conversely, the down-regulation of TRIM47 reduced the ECAR, and reduced mitochondrial respiratory capacity in the PC cells (Figure 3D,3E). Our findings suggest that TRIM47 promotes the cell proliferation of PC cells by activating the Warburg effect.
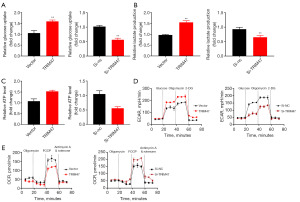
The TRIM47 gene reduced ferroptosis in PC cells via mitochondrial damage
The experiment investigated the potential mechanism by which TRIM47 regulates cell growth in PC cells. The up-regulation of TRIM47 reduced lactate dehydrogenase (LDH) activity levels and the number of propidium-iodide (PI) positive cells, inhibited the iron content, and induced GPX4 protein expression in the PC cells (Figure 4A-4D). The down-regulation of TRIM47 increased the LDH activity level and the number of PI positive cells, promoted iron content, and suppressed GPX4 protein expression in the PC cells (Figure 4A-4C,4E). Further, the up-regulation of TRIM47 increased the calcein levels and 5,5',6,6'-Tetrachloro-1,1',3,3'-tetraethylbenzimidazolylcarbocyanine iodide (JC-1) disaggregation, and the down-regulation of TRIM47 reduced calcein levels and JC-1 disaggregation in the PC cells (Figure 4F,4G). Electron microscopy showed that the up-regulation of TRIM47 mitigated mitochondrial damage, while the down-regulation of TRIM47 exacerbated mitochondrial damage in the PC cells (Figure 4H-4I). These results suggest that mitochondrial damage mediated by pyroptosis accounts for the effects of TRIM47-reduced ferroptosis in PC cells.
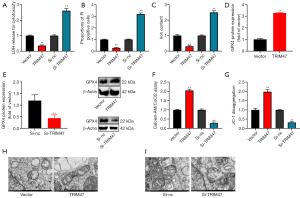
TRIM47 gene induced FBP1 and FOXO1 protein expression in PC cells
Next, we confirmed the effect of TRIM47 on the Warburg effect and ferroptosis in PC cells. The up-regulation of TRIM47 increased TRIM47, FBP1, and FOXO1 protein expression in PC cells (Figure 5A). Conversely, the down-regulation of TRIM47 suppressed TRIM47, FBP1, and FOXO1 protein expression in PC cells (Figure 5B).
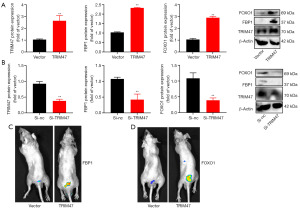
In vivo imaging showed that the up-regulation of TRIM47 increased FBP1 expression in the prostate tissue of the mice model (Figure 5C). Additionally, the up-regulation of TRIM47 also increased FOXO1 expression in the prostate tissue of the mice model (Figure 5D). We also found that si-FBP1 reduced FBP1 protein expression, inhibited cell growth, and the Warburg effect in PC cells via the up-regulation of TRIM47 (Figure 6). These results suggest that TRIM47 promotes the Warburg effect in PC cells via the activation of FBP1.
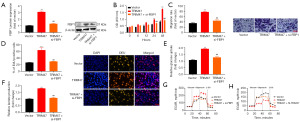
Further, we examined the potential role of TRIM47 in regulating ferroptosis in PC cells via mitochondrial damage. FOXO1 inhibitor (20 µM of JY-2) reduced the expression of the FOXO1 and GPX4 proteins, suppressed cell growth, and enhanced ferroptosis in PC cells via the up-regulation of TRIM47 (Figure 7). These results suggest that TRIM47 activates the FOXO1 axis to mitigate ferroptosis in PC cells.
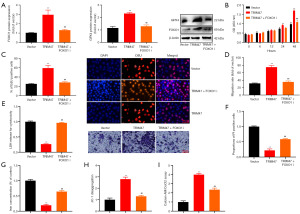
The TRIM47 protein is interlinked with the FBP1 protein or FOXO1 protein
We next investigated the mechanism by which TRIM47 regulates FBP1 or TRIM47 in the PC model. Three-dimensional (3D) model prediction and IP showed TRIM47 at 30-His, 33-GLN, 34-PHE combined with FBP1 at 284-GLN, 299-ARG, and 357-LEN (Figure 8A,8B). Immunofluorescence staining demonstrated that the up-regulation of TRIM47 increased TRIM47 and FBP1 expression in PC cells (Figure 8C). Additionally, 3D model prediction showed TRIM47 at 77-THR, 84-THR, 86-TYR combined with FOXO1 at 251-VAL, 499-ALA, and 501-ASP (Figure 8D,8E). Immunofluorescence staining showed that the TRIM47 protein was interlinked with the FOXO1 protein in the PC cells (Figure 8F). These results suggest that the TRIM47 protein interacts with either the FBP1 protein or TRIM47 protein in PC cells.
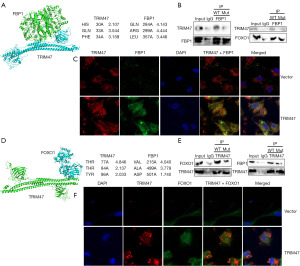
Discussion
At present, PC is the second most common cancer in middle-aged and elderly men, and the third and sixth most common cause of cancer-related death in developed and developing countries, respectively (36). The etiology of PC is complex, and PC has various risk factors, including genetic and environmental factors (37). With changes in lifestyle and the production mode of the Chinese population, the incidence of PC has also increased in younger patients (38).
Interestingly, we showed for the first time that TRIM47 mRNA expression was up-regulated in patients with PC or in PC cells. The survival rate of PC patients with high expression levels of TRIM47 was lower than that of those with low expression levels of TRIM47. Azuma et al. showed that TRIM47 is a significant predictor of a poor prognosis in both breast cancer and PC (27). The findings from this study indicate that TRIM47 participates in the disease progression of PC.
As a typical manifestation of malignant tumor glucose metabolism disorder, the Warburg effect promotes the use of aerobic glycolysis instead of oxidative phosphorylation in malignant tumors, allowing them to undergo glycolysis, even under conditions of sufficient oxygen, thereby rapidly obtaining ATP for rapid growth and metabolism (39). With the advancement and refinement of medical practices, radical prostatectomy has gained widespread acceptance in clinical settings, particularly laparoscopic radical prostatectomy, which is renowned for its minimal invasiveness and high safety profile (39). However, the efficacy of routine rehabilitation training following radical prostatectomy for PC remains limited due to a lack of standardization (40).
In our study, we found that the TRIM47 gene promoted cell growth in PC cells. While Si-TRIM47 reduced cell growth in PC cells. Li et al. showed that TRIM47 accelerates tumor progression in pancreatic cancer (41). Our results showed that the TRIM47 gene promoted PC cell proliferation. However, this study only analyzed the effects of TRIM47 on cell growth in PC cells in an in vitro model, which was one limitation of this study. We intend to use more models to validate our conclusion in the future.
Abnormal metabolism represents a crucial hallmark of tumorigenesis (42). Tumor cells exhibit a propensity to derive energy via glycolysis, even in the presence of ample oxygen, instead of relying on the citric acid cycle and oxidative phosphorylation (42). This phenomenon is one of the most basic metabolic changes in the process of cell malignant transformation, and is called ‘Warburg effect’ (43).
PC is often characterized as a metabolic disease due to its propensity to alter nutrient absorption and metabolic pathways in tumor cells via metabolic reprogramming. This reprogramming includes the activation of aerobic glycolysis (the Warburg effect), the modulation of fatty acid metabolism, and modifications in the tricarboxylic acid cycle to fulfill the biosynthetic and energetic demands of tumors (44). Notably, heightened glucose uptake and lactate production stand out as significant hallmarks of the Warburg effect in tumors (45).
Our results revealed that the TRIM47 gene promoted the Warburg effect in PC cells. We also showed that the inhibition of the TRIM47 gene reduced the Warburg effect in PC cells. Wang et al. demonstrated that TRIM47 promoted cell proliferation in ovarian cancer (32). Therefore, TRIM47 increases the Warburg effect in PC cells and thus promotes cell proliferation in PC.
In recent years, a growing body of research has focused on cell death pathways in PC, which include apoptosis, autophagy, and ferroptosis (46). Numerous studies have noted the considerable research potential of ferroptosis in the onset, progression, and diagnosis of PC (47). Ferroptosis is primarily characterized by its dependence on iron and lipid peroxidation (47). The presence of iron ions precipitates lipid peroxidation, culminating in organelle and cell membrane damage. Microscopically, this often manifests as mitochondrial aberrations, including mitochondrial condensation, the loss of cristae, and the rupture of the outer membrane, which ultimately disrupts cellular energy metabolism and leads to cell demise (48,49). In this study, we found that the TRIM47 gene reduced ferroptosis in PC cells via mitochondrial damage. Wang et al. showed that TRIM47 is partially localized in the mitochondria (50). Thus, these findings suggest that the TRIM47 gene reduces mitochondrial damage to inhibit the ferroptosis of PC cells, which in turn promotes cell growth in PC cells.
FBP1, a pivotal enzyme in the gluconeogenesis process (51), has garnered increasing attention for its role in cancer in recent years (52). These results have promising implications for tumor molecular markers, targeted drug research, and prognosis-related investigations (53). We showed that the TRIM47 gene increased FBP1 and FOXO1 protein expression in PC cells. TRIM47 was found at 30-His, 33-GLN, 34-PHE combined with FBP1 at 284-GLN, 299-ARG, and 357-LEN. Li et al. showed that TRIM47 accelerated the Warburg effect via FBP1 in pancreatic cancer (41). Thus, TRIM47 induces the expression of FBP1, thereby enhancing the Warburg effect in PC cells.
FOXO1 serves as a pivotal regulatory factor in autophagy in the heart, skeletal muscles, liver, and brain. Its role in inhibiting inflammation and cellular apoptosis through deacetylation is crucial for maintaining autophagic degradation activity and promoting the morphological maturation of developing neurons (54). Moreover, FOXO1 activates TLR4 signaling by binding to multiple enhancer elements of the TLR4 gene (55).
Conversely, PI3K activation induces Akt activation, leading to the phosphorylation and inactivation of FOXO1, thereby inhibiting the TLR4 signaling pathway and forming a negative feedback regulatory mechanism of inflammation (56). We found TRIM47 at 30-His, 33-GLN, 34-PHE combined with FBP1 at 284-GLN, and 299-ARG, 357-LEN. We found TRIM47 at 77-THR, 84-THR, 86-TYR combined with FOXO1 at 251-VAL, and 499-ALA, 501-ASP. Wei et al. confirmed that TRIM47 promotes FOXO1 expression in glioma (57). Thus, these findings suggest that TRIM47 promotes the expression of the FOXO1 protein in PC cells, leading to a decrease in ferroptosis in PC cells.
Conclusions
We found that TRIM47 induced the FBP1 and FOXO1 axis to promote the Warburg effect of PC through FBP1 and reduced the ferroptosis of PC by FOXO1. Our findings provide insights into the molecular mechanisms by which TRIM47 regulates the Warburg effect and ferroptosis of PC. Thus, TRIM47 plays a broad role in various aspects of the Warburg effect and the ferroptosis of PC, and the application of TRIM47 might benefit the treatment of PC at various stages of tumor progression.
Acknowledgments
Funding: None.
Footnote
Reporting Checklist: The authors have completed the MDAR and ARRIVE reporting checklists. Available at https://tau.amegroups.com/article/view/10.21037/tau-23-605/rc
Data Sharing Statement: Available at https://tau.amegroups.com/article/view/10.21037/tau-23-605/dss
Peer Review File: Available at https://tau.amegroups.com/article/view/10.21037/tau-23-605/prf
Conflicts of Interest: All authors have completed the ICMJE uniform disclosure form (available at https://tau.amegroups.com/article/view/10.21037/tau-23-605/coif). The authors have no conflicts of interest to declare.
Ethical Statement: The authors are accountable for all aspects of the work in ensuring that questions related to the accuracy or integrity of any part of the work are appropriately investigated and resolved. The study was conducted in accordance with the Declaration of Helsinki (as revised in 2013). This study was approved by the Ethics Committee of Shenzhen People’s Hospital (No. 20180815017). All the subjects signed informed consent forms. All the experiments related to animals were approved by the Animal Care and Use Committee of Shenzhen People’s Hospital (No. 20210814M14) and were performed in compliance with the national guidelines for the care and use of animals.
Open Access Statement: This is an Open Access article distributed in accordance with the Creative Commons Attribution-NonCommercial-NoDerivs 4.0 International License (CC BY-NC-ND 4.0), which permits the non-commercial replication and distribution of the article with the strict proviso that no changes or edits are made and the original work is properly cited (including links to both the formal publication through the relevant DOI and the license). See: https://creativecommons.org/licenses/by-nc-nd/4.0/.
References
- Rizzo A, Santoni M, Mollica V, et al. Microbiota and prostate cancer. Semin Cancer Biol 2022;86:1058-65. [Crossref] [PubMed]
- Biswas S, Wasai A, Ghosh M, et al. A mononuclear N,N,N,O donor schiff base Cu(II) complex inhibits bacterial biofilm formation and promotes apoptosis and cell cycle arrest in prostate cancer cells. J Inorg Biochem 2023;247:112314. [Crossref] [PubMed]
- Buro AW, Carson TL, Small BJ, et al. Sociocultural factors associated with physical activity in Black prostate cancer survivors. Support Care Cancer 2023;31:482. [Crossref] [PubMed]
- Schatten H. Brief Overview of Prostate Cancer Statistics, Grading, Diagnosis and Treatment Strategies. Adv Exp Med Biol 2018;1095:1-14. [Crossref] [PubMed]
- Feng D, Li L, Li D, et al. Prolyl 4-hydroxylase subunit beta (P4HB) could serve as a prognostic and radiosensitivity biomarker for prostate cancer patients. Eur J Med Res 2023;28:245. [Crossref] [PubMed]
- Pu Z, Xu M, Yuan X, et al. Circular RNA circCUL3 Accelerates the Warburg Effect Progression of Gastric Cancer through Regulating the STAT3/HK2 Axis. Mol Ther Nucleic Acids 2020;22:310-8. [Crossref] [PubMed]
- Liu J, Chen G, Liu Z, et al. Aberrant FGFR Tyrosine Kinase Signaling Enhances the Warburg Effect by Reprogramming LDH Isoform Expression and Activity in Prostate Cancer. Cancer Res 2018;78:4459-70. [Crossref] [PubMed]
- Ibs KH, Rink L. Zinc-altered immune function. J Nutr 2003;133:1452S-6S. [Crossref] [PubMed]
- Maan M, Peters JM, Dutta M, et al. Lipid metabolism and lipophagy in cancer. Biochem Biophys Res Commun 2018;504:582-9. [Crossref] [PubMed]
- Recouvreux MV, Moldenhauer MR, Galenkamp KMO, et al. Glutamine depletion regulates Slug to promote EMT and metastasis in pancreatic cancer. J Exp Med 2020;217:e20200388. [Crossref] [PubMed]
- Wang X, Li Q, Sui B, et al. Schisandrin A from Schisandra chinensis Attenuates Ferroptosis and NLRP3 Inflammasome-Mediated Pyroptosis in Diabetic Nephropathy through Mitochondrial Damage by AdipoR1 Ubiquitination. Oxid Med Cell Longev 2022;2022:5411462. [Crossref] [PubMed]
- Wang J, Zeng L, Wu N, et al. Inhibition of phosphoglycerate dehydrogenase induces ferroptosis and overcomes enzalutamide resistance in castration-resistant prostate cancer cells. Drug Resist Updat 2023;70:100985. [Crossref] [PubMed]
- Nie J, Zhang P, Liang C, et al. ASCL1-mediated ferroptosis resistance enhances the progress of castration-resistant prostate cancer to neurosecretory prostate cancer. Free Radic Biol Med 2023;205:318-31. [Crossref] [PubMed]
- Xie W, Agarwal S, Yu J. Ferroptosis: the vulnerability within a cancer monster. J Clin Invest 2023;133:e170027. [Crossref] [PubMed]
- Zhang W, Liu Y, Zhou J, et al. Chicoric acid advanced PAQR3 ubiquitination to ameliorate ferroptosis in diabetes nephropathy through the relieving of the interaction between PAQR3 and P110α pathway. Clin Exp Hypertens 2024;46:2326021. [Crossref] [PubMed]
- Tournant F. Nonenzymatic prevention of insulin hyperresponsiveness by FBP1. Nat Rev Endocrinol 2023;19:380. [Crossref] [PubMed]
- Liang X, Liu X, Li W, et al. A novel variant in the FBP1 gene causes fructose-1,6-bisphosphatase deficiency through increased ubiquitination. Arch Biochem Biophys 2023;742:109619. [Crossref] [PubMed]
- Chen Z, Bao H, Long J, et al. GBE1 Promotes Glioma Progression by Enhancing Aerobic Glycolysis through Inhibition of FBP1. Cancers (Basel) 2023;15:1594. [Crossref] [PubMed]
- Brauer N, Maruta Y, Lisci M, et al. Immunodeficiency with susceptibility to lymphoma with complex genotype affecting energy metabolism (FBP1, ACAD9) and vesicle trafficking (RAB27A). Front Immunol 2023;14:1151166. [Crossref] [PubMed]
- Remadevi V, Muraleedharan P, Sreeja S. FOXO1: a pivotal pioneer factor in oral squamous cell carcinoma. Am J Cancer Res 2021;11:4700-10. [PubMed]
- Wang Y, Lyu Z, Qin Y, et al. FOXO1 promotes tumor progression by increased M2 macrophage infiltration in esophageal squamous cell carcinoma. Theranostics 2020;10:11535-48. [Crossref] [PubMed]
- Xing YQ, Li A, Yang Y, et al. The regulation of FOXO1 and its role in disease progression. Life Sci 2018;193:124-31. [Crossref] [PubMed]
- Kurakazu I, Akasaki Y, Hayashida M, et al. FOXO1 transcription factor regulates chondrogenic differentiation through transforming growth factor β1 signaling. J Biol Chem 2019;294:17555-69. [Crossref] [PubMed]
- Qian Y, Wang Z, Lin H, et al. TRIM47 is a novel endothelial activation factor that aggravates lipopolysaccharide-induced acute lung injury in mice via K63-linked ubiquitination of TRAF2. Signal Transduct Target Ther 2022;7:148. [Crossref] [PubMed]
- Liang Q, Tang C, Tang M, et al. TRIM47 is up-regulated in colorectal cancer, promoting ubiquitination and degradation of SMAD4. J Exp Clin Cancer Res 2019;38:159. [Crossref] [PubMed]
- Azuma K, Ikeda K, Suzuki T, et al. TRIM47 activates NF-κB signaling via PKC-ε/PKD3 stabilization and contributes to endocrine therapy resistance in breast cancer. Proc Natl Acad Sci U S A 2021;118:e2100784118. [Crossref] [PubMed]
- Azuma K, Inoue S. Efp/TRIM25 and Its Related Protein, TRIM47, in Hormone-Dependent Cancers. Cells 2022;11:2464. [Crossref] [PubMed]
- Wu L, Jia M, Xiao L, et al. TRIM-containing 44 aggravates cardiac hypertrophy via TLR4/NOX4-induced ferroptosis. J Mol Med (Berl) 2023;101:685-97. [Crossref] [PubMed]
- Liu Y, Jiang N, Chen W, et al. TRIM59-mediated ferroptosis enhances neuroblastoma development and chemosensitivity through p53 ubiquitination and degradation. Heliyon 2024;10:e26014. [Crossref] [PubMed]
- Zhang J, Xie H, Yao J, et al. TRIM59 promotes steatosis and ferroptosis in non-alcoholic fatty liver disease via enhancing GPX4 ubiquitination. Hum Cell 2023;36:209-22. [Crossref] [PubMed]
- Liu W, Zhao Y, Wang G, et al. TRIM22 inhibits osteosarcoma progression through destabilizing NRF2 and thus activation of ROS/AMPK/mTOR/autophagy signaling. Redox Biol 2022;53:102344. [Crossref] [PubMed]
- Wang X, Fu Y, Xing Y. TRIM47 promotes ovarian cancer cell proliferation, migration, and invasion by activating STAT3 signaling. Clinics (Sao Paulo) 2022;77:100122. [Crossref] [PubMed]
- Pu Z, Wang W, Xie H, et al. Apolipoprotein C3 (ApoC3) facilitates NLRP3 mediated pyroptosis of macrophages through mitochondrial damage by accelerating of the interaction between SCIMP and SYK pathway in acute lung injury. Int Immunopharmacol 2024;128:111537. [Crossref] [PubMed]
- Pu Z, Zhang W, Wang M, et al. Schisandrin B Attenuates Colitis-Associated Colorectal Cancer through SIRT1 Linked SMURF2 Signaling. Am J Chin Med 2021;49:1773-89. [Crossref] [PubMed]
- Pu Z, Han C, Zhang W, et al. Systematic understanding of the mechanism and effects of Arctigenin attenuates inflammation in dextran sulfate sodium-induced acute colitis through suppression of NLRP3 inflammasome by SIRT1. Am J Transl Res 2019;11:3992-4009. [PubMed]
- Huang Q, Grossett V, Daley S, et al. Engagement with a local African-Caribbean community to explore perspectives and research priorities among Black men for prostate cancer using a co-participatory approach. Br J Gen Pract 2023;73:bjgp23X733797.
- Li X, Wang Y, Deng S, et al. Loss of SYNCRIP unleashes APOBEC-driven mutagenesis, tumor heterogeneity, and AR-targeted therapy resistance in prostate cancer. Cancer Cell 2023;41:1427-1449.e12. [Crossref] [PubMed]
- Haining L, Chunyan Z, Xiaopei L, et al. Prolonged APTT secondary to factor XII deficiency in a patient with prostate cancer: A case report. Asian J Surg 2023;46:5182-3. [Crossref] [PubMed]
- Wilson PFR, Gilany M, Jamzad A, et al. Self-Supervised Learning with Limited Labeled Data for Prostate Cancer Detection in High Frequency Ultrasound. IEEE Trans Ultrason Ferroelectr Freq Control 2023;70:1073-83. [Crossref] [PubMed]
- Lubarsky R, Ambinder D, Barnett J, et al. Prostate Cancer: Under-representation of African American Men in Research Studies Used in the Latest NCCN Guidelines. Urology 2023;180:28-34. [Crossref] [PubMed]
- Li L, Yu Y, Zhang Z, et al. TRIM47 accelerates aerobic glycolysis and tumor progression through regulating ubiquitination of FBP1 in pancreatic cancer. Pharmacol Res 2021;166:105429. [Crossref] [PubMed]
- Singh KB, Hahm ER, Alumkal JJ, et al. Reversal of the Warburg phenomenon in chemoprevention of prostate cancer by sulforaphane. Carcinogenesis 2019;40:1545-56. [Crossref] [PubMed]
- Song C, Zhang J, Liu X, et al. PTEN loss promotes Warburg effect and prostate cancer cell growth by inducing FBP1 degradation. Front Oncol 2022;12:911466. [Crossref] [PubMed]
- van der Mijn JC, Kuiper MJ, Siegert CEH, et al. Lactic Acidosis in Prostate Cancer: Consider the Warburg Effect. Case Rep Oncol 2017;10:1085-91. [Crossref] [PubMed]
- Russo GI, Asmundo MG, Lo Giudice A, et al. Is There a Role of Warburg Effect in Prostate Cancer Aggressiveness? Analysis of Expression of Enzymes of Lipidic Metabolism by Immunohistochemistry in Prostate Cancer Patients (DIAMOND Study). Cancers (Basel) 2023;15:948. [Crossref] [PubMed]
- Wang X, Shen C, Wang X, et al. Schisandrin protects against ulcerative colitis by inhibiting the SGK1/NLRP3 signaling pathway and reshaping gut microbiota in mice. Chin Med 2023;18:112. [Crossref] [PubMed]
- Jia G, Wu W, Chen L, et al. HSF1 is a novel prognostic biomarker in high-risk prostate cancer that correlates with ferroptosis. Discov Oncol 2023;14:107. [Crossref] [PubMed]
- Liang J, Liao Y, Wang P, et al. Ferroptosis landscape in prostate cancer from molecular and metabolic perspective. Cell Death Discov 2023;9:128. [Crossref] [PubMed]
- Campbell T, Hawsawi O, Henderson V, et al. Novel roles for HMGA2 isoforms in regulating oxidative stress and sensitizing to RSL3-Induced ferroptosis in prostate cancer cells. Heliyon 2023;9:e14810. [Crossref] [PubMed]
- Wang Y, Kuang M, Lu Y, et al. Characterization and biological function analysis of the TRIM47 gene from common carp (Cyprinus carpio). Gene 2017;627:188-93. [Crossref] [PubMed]
- Zhang G, Tao J, Lin L, et al. Repurposing FBP1: dephosphorylating IκBα to suppress NFκB. Cell Res 2023;33:419-20. [Crossref] [PubMed]
- Zhao W, Zhao J, Li K, et al. Oncogenic Role of the NFATC2/NEDD4/FBP1 Axis in Cholangiocarcinoma. Lab Invest 2023;103:100193. [Crossref] [PubMed]
- Wang Z, He T, Lv W, et al. Down-regulation of FBP1 in lung adenocarcinoma cells promotes proliferation and invasion through SLUG mediated epithelial mesenchymal transformation. Transl Cancer Res 2023;12:236-46. [Crossref] [PubMed]
- Chae YC, Kim JY, Park JW, et al. FOXO1 degradation via G9a-mediated methylation promotes cell proliferation in colon cancer. Nucleic Acids Res 2019;47:1692-705. [Crossref] [PubMed]
- Chen J, Lu Y, Tian M, et al. Molecular mechanisms of FOXO1 in adipocyte differentiation. J Mol Endocrinol 2019;62:R239-53. [Crossref] [PubMed]
- Adiguzel D, Celik-Ozenci C. FoxO1 is a cell-specific core transcription factor for endometrial remodeling and homeostasis during menstrual cycle and early pregnancy. Hum Reprod Update 2021;27:570-83. [PubMed]
- Wei H, Ding C, Zhuang H, et al. TRIM47 Promotes the Development of Glioma by Ubiquitination and Degradation of FOXO1. Onco Targets Ther 2020;13:13401-11. [Crossref] [PubMed]
(English Language Editor: L. Huleatt)