Lycopene’s therapeutic mechanisms in epididymitis: a network pharmacology and experimental study
Highlight box
Key findings
• This study found that lycopene (LYC) significantly alleviates epididymitis.
• The results indicate that LYC reduces damage to the epididymal tissue by inhibiting inflammatory factors and enhancing the activity of antioxidant enzymes.
What is known and what is new?
• LYC is known for its anti-inflammatory and antioxidant properties, but the specific mechanisms related to its treatment of epididymitis are unclear.
• This study is the first to systematically reveal the mechanisms by which LYC exerts therapeutic effects in epididymitis.
What is the implication, and what should change now?
• The findings suggest that LYC could be a potential therapeutic agent for epididymitis, warranting further research into its clinical applications.
• Additionally, current treatment strategies may need to be adjusted to incorporate the mechanisms of LYC to optimize therapeutic outcomes.
Introduction
Epididymitis, a common disease involving the male reproductive system, is caused by nonspecific infections, with a higher incidence reported in middle-aged and young males. Epididymitis can be divided into acute epididymitis and chronic epididymitis, with the latter being more prevalent (1). Chronic epididymitis usually develops from prolonged acute epididymitis and can also occur as a complication of chronic prostatitis (2). The typical clinical presentation of epididymitis is the gradual onset of scrotal pain, which may be accompanied by urinary system symptoms such as dysuria and frequent urination (3). Physical examinations often reveal epididymal tenderness (which can extend to the adjacent testicle), scrotal swelling, and pain relief when the scrotum is elevated (Prehn sign). Antibiotics are the most commonly used drugs for treating epididymitis. However, this treatment method is not always effective due to increasing antibiotic resistance, and antibiotics alone cannot reverse the histopathological changes associated with epididymitis, which may lead to persistent reproductive issues (4,5). These limitations highlight the need for alternative or adjunct therapies that address both infection and tissue damage in the treatment of epididymitis. Therefore, there is a critical need to develop drugs as alternatives or adjuncts to potent broad-spectrum antibiotics in the treatment of epididymitis.
As a natural carotenoid extracted from plants, lycopene (LYC) is characterized by a long chain and multiple conjugated double bonds. It is widely present in such plants as carrots, watermelons, and tomatoes (6,7). In addition to this, LYC also plays an important role in anti-inflammation and immune regulation, which may further help reduce tissue damage and inflammation in epididymitis. Due to these antioxidant and anti-inflammatory properties, LYC could offer therapeutic benefits beyond those provided by conventional antibiotics (8). LYC cannot be synthesized autonomously in the human body but can only be obtained through certain diets. Several clinical studies have been conducted on the treatment of epididymitis with LYC. For example, Li et al. found that oral administration of LYC can alleviate inflammation and oxidative stress in lipopolysaccharide (LPS)-induced epididymitis in rats by regulating lipids (9). Raj et al. reported that LYC exerts an ameliorating effect on the epididymal toxicity caused by polychlorinated biphenyls (10). These studies provide supporting evidence for the efficacy of LYC in the treatment of epididymitis.
Network pharmacology is an emerging discipline that is based on the interaction between diseases, genes, targets, and drugs, and network analyses can be conducted through virtual computer calculation and high-throughput omics data. Network pharmacology is primarily used to predict drug targets from a holistic perspective and improve the efficiency of drug discovery. Aided by this method, investigators can better understand the interactions between drugs and diseases and accelerate the development of novel drugs (11). In the conventional drug development model, the dogmatic approach of “one disease, one target, one drug” has significantly hindered innovation. For instance, the majority of drugs demonstrate highly limited efficacy in treating complex diseases, impairing the success rate of newly developed drugs. The emergence of network pharmacology has transformed the methods of defining, diagnosing, and treating diseases. Through combining network pharmacology and drug repositioning, we can formulate precise and effective therapeutic strategies and accelerate relevant clinical translation (12).
In this study, network pharmacology, molecular docking, and in vivo experiments were integrated to provide reliable evidence for clarifying the potential mechanisms underlying the therapeutic effect of LYC on epididymitis. This study specifically aims to investigate how LYC’s antioxidant and anti-inflammatory effects might alleviate epididymitis, focusing on key signaling pathways involved in inflammation and oxidative stress. We present this article in accordance with the ARRIVE reporting checklist (available at https://tau.amegroups.com/article/view/10.21037/tau-24-567/rc).
Methods
Animals and induction of epididymitis mice
Male C57BL/6 mice (8 weeks old) were obtained from GemPharmatech Co., Ltd. (Nanjing, China). All the mice were housed in a specific-pathogen-free (SPF) environment at GemPharmatech and treated strictly according to protocols approved by the company. All animal experiments were approved by the Animal Experiment Ethics Committee of the Eastern Theater General Hospital (approval No. DZGZRDW2400120) and GemPharmatech Co., Ltd. (approval No. GPTAP20231225-1), in accordance with national guidelines for the care and use of animals in research. The mice were randomly allocated to the control group, LPS group, and LPS + LYC group using a computer-generated random sequence. This randomization ensured balanced distribution among the groups and minimized selection bias. Epididymitis mouse modeling was performed as described in previous studies (13,14). Briefly, after the mice were anesthetized with pentobarbital sodium, 12.5 µg of LPS was injected longitudinally near the tail of the epididymitis model mice (Figure 1). Meanwhile, an equal volume of saline was injected into the mice in the control group. A protocol was prepared before the study without registration.
LYC administration
After modeling, all mice were divided into a control group, LPS group, and LPS + LYC group, with 7 mice in each group. The mice in the LPS + LYC group were orally administered 20 mg/kg of LYC 24 hours after modeling (13), while those in the control group and LPS group were orally administered an equal volume of saline. The mice in all groups received intervention for 2 consecutive weeks. After the 2 weeks, mice were anesthetized with pentobarbital sodium, and blood samples were collected from the orbital vein. Subsequently, mice were humanely euthanized via cervical dislocation. The epididymal tissue was then extracted for further experimentation.
Collection of drug targets
The structure of LYC was obtained from the Traditional Chinese Medicine Systems Pharmacology Database and Analysis Platform (TCMSP) (https://old.tcmsp-e.com/tcmsp.php) and imported into the PharmMapper database (https://www.lilab-ecust.cn/pharmmapper/). Subsequently, drug targets with a Norm Fit prediction score greater than 0 were selected as potential drug targets. The target names were corrected using the UniProt database (https://www.uniprot.org/).
Collection of disease targets
The disease-related targets were collected from the Online Mendelian Inheritance in Man (OMIM) (http://www.omim.org), Disgenet (https://www.disgenet.org), and GeneCards (http://www.genecards.org) platforms. Keywords such as “epididymitis” and “epididymis inflammation” were used for retrieval. After the disease-related targets were obtained, duplicates were removed to create a refined list of unique targets related to epididymitis. Each target was then standardized using UniProt identifiers to maintain consistency across datasets and facilitate further analysis. The study was conducted in accordance with the Declaration of Helsinki (as revised in 2013).
Prediction of potential targets of LYC in the treatment of epididymitis
The drug targets and disease targets were input into the Venny (2.1.0) platform, with the LYC targets and epididymitis targets being input to obtain the intersection targets.
Construction and analysis of a protein-protein interaction (PPI) network
The common targets were imported into the Search Tool for the Retrieval of Interacting Genes/Proteins (STRING) database (https://string-db.org/) for retrieval. “Homo sapiens” was set as the protein type to obtain information on PPIs, based on which a PPI network was constructed to identify the relationship between targets. The key targets were selected according to network topology theory, which holds that within a network, targets that exhibit higher information transfer efficiency and influence a greater number of nodes play a more significant role in the PPI network. The PPI network was visualized using Cytoscape (3.10.0), and the topological analysis of the PPI network was conducted using the CytoNCA plugin. The analysis metrics included degree centrality (DC), betweenness centrality (BC), eigenvector centrality (EC), and closeness centrality (CC). The key targets identified were those nodes with several metrics greater than the median of all nodes (15).
Enrichment analysis
To further clarify the biological functions of the target genes, all compound target genes were imported into the Database for Annotation, Visualization and Integrated Discovery (DAVID; https://david.ncifcrf.gov/) for pathway enrichment analyses. In the “Gene List Manager” panel of DAVID, the gene list was pasted, “OFFICIAL GENE SYMBOL” was selected for the “Select Identifier” option, “List Type” was set as “Gene List”, and the gene list was submitted. For the submitted gene list, “Homo sapiens” was selected as the species to perform the Gene Ontology (GO) functional enrichment analysis and Kyoto Encyclopedia of Genes and Genomes (KEGG) pathway enrichment analysis, with P<0.05 being the cutoff.
Construction of the target-pathway network diagram
The 20 pathways with the smallest P values from the KEGG analysis of LYC treatment for epididymitis and related targets were integrated in Cytoscape (3.10.0) to construct a target-pathway network diagram.
Molecular docking
Molecular docking and visualization of key targets in the phosphatidylinositol 3-kinase/protein kinase B (PI3K/AKT) pathway and LYC were conducted with the aid of BIOVIA Discovery Studio 2019 version 19.1.0 and AutoDock Tools version 4.2.
Enzyme-linked immunosorbent assay
The mouse blood was collected from the orbital vein, and the serum was separated by centrifugation. The serum levels of interleukin-1 beta (IL-1β), interleukin-6 (IL-6), and tumor necrosis factor alpha (TNF-α) were measured with a Mouse IL-1β ELISA Kit (E-EL-M0037; Elabscience, Houston, TX, USA), Mouse IL-6 ELISA Kit (E-EL-M0044; Elabscience), and Mouse TNF-α ELISA Kit (E-EL-M3063; Elabscience), respectively, according to the manufactures’ protocol.
Hematoxylin and eosin (HE) staining
The mouse epididymis was fixed in a solution containing 4% paraformaldehyde (4% PFA) for more than 24 hours, embedded in paraffin, and then sliced into sections with a thickness of 4 µm. The sections were then subjected to a series of treatment procedures, including immersion in dimethylbenzene I and II, anhydrous ethanol I and II, and 75% alcohol, followed by a rinsing with tap water. For HE staining, the sections were placed in the hematoxylin staining solution for 3–5 min, differentiated using a differentiation solution, and then counterstained with eosin after dehydration in 85% and 95% ethanol. Subsequently, the sections were mounted with a transparent and neutral mounting medium after sequential dehydration in anhydrous ethanol I–III and dimethylbenzene I and II. The images were captured and analyzed with the aid of an optical microscope.
Immunofluorescence staining
Immunofluorescence staining was conducted with a TSAPLus Fluorescent Triple Staining Kit (G1236; Servicebio, Wuhan, China) in accordance with the instructions provided by the manufacturer. After the staining procedure, the images were captured with a fluorescence microscope and subsequently analyzed in ScopeImage 9.0 software (V1.0, developed by Ningbo Yongxin Optical Co., Ltd., Ningbo, China). The negative controls were incubated solely with secondary antibodies (Figure S1). The primary antibodies and secondary antibodies are listed in Table S1.
Western blotting (WB)
The WB procedure was conducted in accordance with a previously outlined method (16). Briefly, the epididymis of mice was lysed, and the concentration of the proteins was measured. Subsequently, the proteins were segregated via sodium dodecyl sulfate-polyacrylamide gel electrophoresis (SDS-PAGE) and transferred onto a polyvinylidene fluoride membrane. After the treatment with a 2% bovine serum albumin (BSA) blocking agent, the membrane was incubated overnight with primary antibodies (Table S1) at 4 °C, followed by the incubation with secondary antibodies (Table S1). The bands were detected using chemiluminescence and quantified using ScopeImage 9.0.
Terminal deoxynucleotidyl transferase-mediated dUTP nick end labelling (TUNEL) assay
The apoptosis in the epididymis of mice was evaluated using the One-Step TUNEL In Situ Apoptosis Kit [green, fluorescein isothiocyanate (FITC)] (E-CK-A320; Elabscience). Following deparaffinization and rehydration, mouse epididymis sections were treated with the proteinase K solution (20 µg/mL) at room temperature for 20 min, followed by three 5-min washes with phosphate-buffered saline (PBS). Subsequently, the sections were incubated in the terminal deoxynucleotidyl TUNEL solution at 37 °C for 1 hour. Next, the sections were stained with 4’,6-diamidino-2-phenylindole (DAPI; 2 µg/mL) and then incubated in darkness at room temperature for 10 min. The fluorescent signals were observed under a fluorescence microscope (Nikon DS-U3, Nikon Instruments Inc., Tokyo, Japan). The total count of apoptotic cells within 10 lumens was tallied, and then the average number of tubules was computed.
Statistical analysis
The data were statistically analyzed using SPSS 26 software (IBM Corp., Armonk, NY, USA). The measurement data are expressed as mean ± standard deviation. The independent samples t-test was employed for comparisons of two sets of data, while one-way analysis of variance (ANOVA) was used for comparisons involving multiple sets of data. A P value ≤0.05 was considered statistically significant.
Results
Potential targets of LYC for the treatment of epididymitis
The disease-associated target genes were identified based on the OMIM, Disgenet, and GeneCards databases. After the duplicates were removed, a total of 2,150 target genes related to epididymitis were obtained. Additionally, 188 LYC-related target genes were identified on the PharmMapper database. A Venn diagram was generated based on the intersection of LYC target genes and epididymitis-related target genes, which revealed 78 common target genes between the two sets (Figure 2). These common target genes serve as potential points of intervention for LYC in the treatment of epididymitis, particularly in pathways related to inflammation and apoptosis.
PPI network establishment and core target identification
To further investigate the interactions between these potential candidate targets, we obtained PPI data from the STRING database. After the 78 common targets were imported into the STRING database, 72 showed interactions. Subsequently, the data were exported and visualized using Cytoscape 3.10.0 (Figure 3). This network included 72 nodes and 537 edges. In further topological analysis, CytoNCA was employed to calculate the DC, BC, EC, and CC values of the network nodes. The median values were as follows: DC =11, BC =24.256598, EC =0.073143, and CC =0.505344. According to network pharmacology screening criteria (DC >11, BC >24.256598, EC >0.073143, and CC >0.505344), a total of 41 key targets were identified, including ALB, PPARG, EGFR, SRC, ESR1, HSP90AA1, CASP3, IGF1, MAPK14, GRB2, and PIK3R1. Among these targets, inflammation-related proteins (CASP3, BCL2L1, PIK3R1, AKT2, and IL2) and apoptosis-related proteins (BCL2L1, PIK3R1, and AKT2) were prominent, suggesting that LYC may exert its therapeutic effects by modulating inflammatory and apoptotic pathways. These proteins represented the core target proteins in the PI3K/AKT signaling pathway, which is known to regulate both inflammation and apoptosis in cellular environments.
GO and KEGG enrichment analyses
A total of 177 significant GO terms (P<0.05) were identified through a GO functional enrichment analysis based on DAVID and included 105 biological processes (BPs) terms, 33 cellular components (CCs) terms, and 39 molecular functions (MFs) terms. The BPs included negative regulation of the apoptotic process, peptidyl-tyrosine phosphorylation, and peptidyl-tyrosine autophosphorylation. The CCs with the most significant correlation with these targets included cytosol, extracellular exosome, and cytoplasm. The MF with the most significant correlation with these targets included RNA polymerase II transcription factor activity, ligand-activated sequence-specific DNA binding, protein tyrosine kinase activity, and protein serine/threonine/tyrosine kinase activity (Figure 4A).
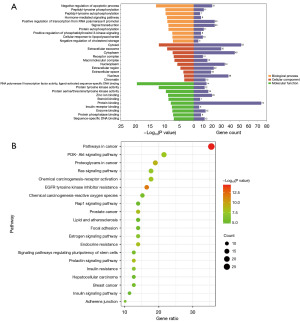
In addition, a total of 58 significantly enriched pathways (P<0.05) were identified through KEGG pathway enrichment analysis and included cancer pathways, the PI3K/AKT signaling pathway, proteoglycans in cancer, the Ras signaling pathway, and the chemical carcinogenesis-receptor activation pathway. Among these pathways, the PI3K/AKT signaling pathway, chemical carcinogenesis-receptor activation pathway, EGFR tyrosine kinase inhibitor resistance pathway, and Rap1 signaling pathway were closely correlated with inflammation. The PI3K/AKT pathway was particularly emphasized in this study, as it plays a critical role in inflammation modulation, cellular proliferation, and apoptosis inhibition. The focus on key molecules within this pathway, such as PIK3R1, AKT, and BCL2L1, underscores the potential of LYC in targeting these inflammatory and apoptotic processes. In these pathways, the PI3K/AKT/PTEN signaling pathways ranked highly (Figure 4B).
Molecular docking validation
In the molecular docking analysis, LYC was docked with PIK3R1 [Protein Data Bank (PDB): 1pbw], AKT1 (PDB: 3e88), and BCL2L1 (PDB: 6f46). The binding energy of the three active compounds to the protein targets was all less than −5.0 kcal/mol, indicating excellent binding affinity (Figure 5 and Table 1). These binding affinities suggest that LYC could effectively interact with key targets in the PI3K/AKT pathway, potentially modulating their activities to exert anti-inflammatory and anti-apoptotic effects.
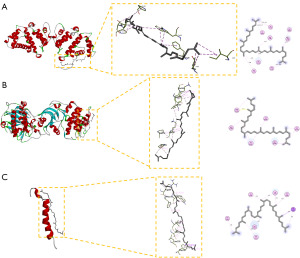
Table 1
Target | PDB ID | Binding energy (kcal/mol) |
---|---|---|
PIK3R1 | 1pbw | −6.3 |
AKT2 | 3e88 | −7.0 |
BCL2L1 | 6f46 | −6.7 |
PDB ID, Protein Data Bank identification; PIK3R1, phosphoinositide-3-kinase regulatory subunit 1; AKT2, AKT serine/threonine kinase 2; BCL2L1, B-cell lymphoma 2-like 1.
Experimental validation
The HE staining results revealed significant changes in the epididymal tissue of mice in the LPS group compared with the control group. These changes included a marked increase in inflammatory cell infiltration, widening of gaps between epididymal epithelial cells, reduced sperm count, inflammatory cell infiltration into the tubular lumen, and disrupted and irregular epididymal epithelial cell structures. However, after the intervention with LYC, inflammatory cell infiltration was alleviated significantly, cell structures became clearer, and lesion severity was reduced (Figure 6).
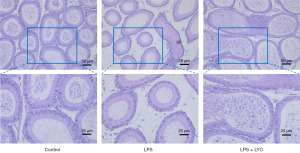
Effect of LYC on inflammatory factors
LPS can trigger a strong immune response to mediate a series of systemic and local inflammations. To evaluate the systemic inflammation induced by LPS in mice, the level of serum cytokines, including proinflammatory cytokines, was measured. In the LPS group, the levels of IL-1β, IL-6, and TNF-α in the serum were significantly increased (P<0.001); compared with those in the LPS group, the levels of these cytokines in the LPS + LYC group were decreased to varying degrees (P<0.001, P<0.01, and P<0.001, respectively) (Figure 7). These results indicated that LPS resulted in the excessive production of cytokines in the serum, stimulating a systemic inflammatory response. However, the oral administration of LYC significantly reduced the levels of these cytokines in the serum, alleviating the systemic inflammatory response.
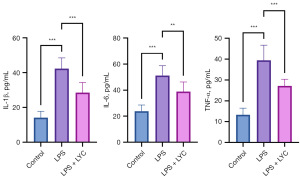
The impact of LPS-induced inflammation on the epididymis was also evaluated. Initially, immunofluorescence assays were conducted to measure the levels of IL-1β, IL-6, and TNF-α in the epididymal tissue. The results revealed a significant increase in the fluorescence intensity of these inflammatory markers in the LPS group compared with the control group (P<0.001). Moreover, the LPS + LYC group exhibited a significant decrease in the average fluorescence intensity of IL-1β, IL-6, and TNF-α compared with the LPS group (P<0.001) (Figure 8).
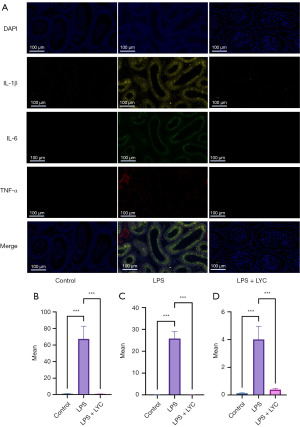
To further confirm the presence of inflammation in the epididymis, WB was performed to measure the protein expression levels of IL-1β, IL-6, and TNF-α. The results indicated a marked elevation in the protein expression level of these inflammatory markers in the epididymal tissue of mice in the LPS group compared with the control group (P<0.001, P<0.01, and P<0.01, respectively). Conversely, the LPS + LYC group displayed significantly downregulated protein expression levels of IL-1β, IL-6, and TNF-α as compared with the LPS group (P<0.05, P<0.05, and P<0.01, respectively) (Figure 9). These results suggested that LYC can reduce the release of inflammatory factors in epididymitis mice.
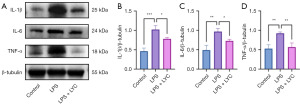
Effects of LYC on the enhancement of the antioxidant defense capability against the epididymis
To evaluate the oxidative stress levels in the epididymitis mice before and after LYC intervention, the activity of antioxidant enzymes and the level of the lipid peroxidation product malondialdehyde (MDA) in the epididymitis mice were measured. In the epididymal homogenate of mice in the LPS group, the activity of the antioxidant enzymes [including glutathione peroxidase (GSH-PX), superoxide dismutase (SOD), and catalase (CAT)] and the total antioxidant capacity (T-AOC) decreased significantly (P<0.001, P<0.001, P<0.05, P<0.001, and P<0.001, respectively); meanwhile, the level of the lipid peroxidation product MDA increased significantly (P<0.001). Compared with the LPS group, LPS + LYC group demonstrated significantly increased levels of GSH-PX, SOD, CAT, and T-AOC and a significantly decreased level of MDA in the epididymal homogenate (P<0.01, P<0.05, P<0.01, P<0.001, and P<0.001, respectively) (Figure 10). The results of these oxidative stress-related indicators suggested that LPS disrupted the oxidative-antioxidant balance in the epididymis, leading to oxidative stress damage. LYC significantly increased the activity of antioxidant enzymes in the epididymal tissue and enhanced antioxidant defense.
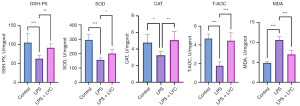
Effects of LYC on apoptosis in the epididymal tissue of mice with epididymitis
The results showed that the epididymal tissue of mice in the control group had a clear structural hierarchy and a low number of apoptotic cells, with some regions showing no apoptotic cells and others showing very few apoptotic cells. In the LPS group, the number of apoptotic cells increased significantly (P<0.001), and the lumen was arranged irregularly. Compared to that in the LPS group, the number of apoptotic cells in the LPS + LYC group was significantly lower (P<0.001), and the lumen structure remained relatively intact (Figure 11). These experimental results indicated that LYC can effectively inhibit apoptosis in the epididymal tissue of mice.
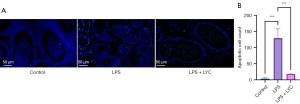
Effects of LYC on the PI3K/AKT signaling pathway in mice with epididymitis
WB was conducted to measure the expression of the proteins involved in the PI3K/AKT signaling pathway, including phospho-PI3K (p-PI3K), PI3K, phospho-AKT (p-AKT), AKT, Bcl-2-associated X protein (BAX), and B-cell lymphoma-extra large (Bcl-xL). The results were consistent with the significantly increased p-PI3K:PI3K ratio in the LPS group as compared to that in the control group (P<0.05), which decreased significantly after intervention with LYC (P<0.01). The p-AKT:AKT ratio also increased in the LPS group (P<0.05) but decreased after intervention with LYC (P<0.05). LPS treatment significantly decreased the Bcl-xL:BAX ratio (P<0.05), which was reversed after intervention with LYC (P<0.05) (Figure 12).
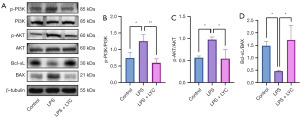
Discussion
Antibiotics are commonly used treatment methods for epididymitis. Despite routine treatment with antibiotics, 40–80% of patients may still experience impaired semen parameters and persistent epididymal swelling for up to 3 months (17,18). Therefore, it is impossible to cure epididymitis by solely relying on antibiotics (19,20). In recent years, researchers have explored antioxidants as a new approach to the treatment of epididymitis (19-21). LYC, a potent antioxidant, is capable of scavenging free radicals, reducing inflammation, and promoting tissue repair, which constitutes the theoretical basis supporting its application in epididymitis treatment (22,23). By reducing oxidative stress and inflammation, LYC may alleviate epididymal tissue damage and potentially reduce infections.
In this study, network pharmacology and molecular docking techniques were integrated to explore the potential mechanisms of LYC in the treatment of epididymitis, and the results were validated through animal experiments. First, 78 common targets of LYC in treating epididymitis were identified through network pharmacology. These common targets may play crucial roles in efficient information transmission within networks and contribute significantly to the anti-inflammatory effect of LYC. Additionally, these targets are involved in multiple cellular processes, such as antioxidative stress, anti-inflammation, and apoptosis regulation. For instance, key proteins such as PIK3R1, AKT2, and BCL2L1 are directly associated with the PI3K/AKT pathway, which is known to modulate inflammation and apoptosis. This connection reinforces the hypothesis that LYC exerts its effects by targeting these pathways. The experimental results demonstrated that LYC significantly decreased the levels of such key inflammatory factors including IL-1β, IL-6, and TNF-α. Specifically, compared with the LPS group, the LPS + LYC group exhibited significantly decreased levels of these cytokines, confirming the anti-inflammatory effect of LYC at the molecular level. These cytokines are critical mediators in the inflammatory response, and their regulatory effects can effectively control the progression and intensity of inflammation. The pathological changes observed indicated that mice in the LPS group experienced epididymal tissue structure damage and significant infiltration of inflammatory cells. In contrast, this pathological state was significantly improved in the LPS + LYC group, which demonstrated reduced inflammatory cell infiltration and a more normal arrangement of epididymal epithelial cells. These results highlight the significant anti-inflammatory effects of LYC, which could be attributable to the reduction of inflammatory mediator release and improvement of local blood flow. This result was consistent with the finding of Raj et al., who reported the mitigating effect of LYC on epididymal toxicity induced by polychlorinated biphenyls, further supporting the potential application of LYC in the treatment of epididymitis (10). Furthermore, the molecular docking results showed strong binding affinities between LYC and key targets like PIK3R1, AKT1, and BCL2L1, which are part of the PI3K/AKT signaling pathway. This consistency between molecular docking and experimental data reinforces the hypothesis that LYC modulates these proteins to achieve its anti-inflammatory and anti-apoptotic effects.
Regarding antioxidant properties, LYC significantly enhanced the levels of SOD, CAT, GSH-PX, and T-AOC, while decreasing the level of MDA, indicating the alleviation of oxidative stress. Oxidative stress is closely related to many pathological processes of inflammation. The antioxidant effect of LYC can prevent the cell damage and inflammatory responses caused by free radicals (24).
In this study, the KEGG pathway enrichment analysis results revealed that LYC targets for treating epididymitis were mainly enriched in such pathways as PI3K/AKT signaling, Ras signaling, and HIF-1 signaling, which were associated with inflammation and cell proliferation. Among them, the PI3K/AKT pathway played a crucial role in the development of inflammation. Through the intervention in this pathway, mitochondrial function, oxidative stress, and inflammatory responses can be regulated (25). LYC can effectively alleviate epididymal inflammatory responses and mitigate tissue damage by regulating this pathway, thus exerting anti-inflammatory, antioxidant, and antiapoptotic effects. The topological analysis results revealed 41 key nodes, and 17 important targets related to the PI3K/AKT pathway were further identified. Through the in-depth analysis of targets and pathways, it was found that LYC can influence several key proteins, including PIK3R1, AKT2, and BCL2L1. This intervention modulated the PI3K/AKT signaling pathway, which played a crucial regulatory role in various inflammatory diseases.
TUNEL staining revealed significantly increased cell apoptosis, characterized by disordered cell arrangement, in the epididymal tissue of mice in the LPS group, which occurred due to local inflammatory reactions and cellular stress induced by LPS. However, in the LPS + LYC group, the number of apoptotic cells significantly decreased, and cell structure was more intact, attesting to the significant protective effect of LYC on cells. The decrease in apoptosis may be attributed to the inhibitory effect of LYC on inflammatory mediator production and regulatory effects on apoptotic pathways (26). PIK3R1, as the regulatory subunit of PI3K, directly affects the activation state of PI3K. Therefore, the effect of LYC on PI3K activity may be assessed by detecting PI3K and its phosphorylated state (p-PI3K) through WB. Similarly, AKT2, a member of the AKT family, is associated with the regulation of cell survival and proliferation. Therefore, the potential regulatory effects of LYC on AKT signal transmission may be observed by detecting AKT and its phosphorylated form (p-AKT). BCL2L1 can encode Bcl-xL, which is a crucial antiapoptotic protein regulating cell survival. Measuring Bcl-xL expression directly reflects the effects of LYC on the apoptotic process. Moreover, BAX, a proapoptotic protein that can regulate cell death, was also examined in this study. By measuring the BAX:Bcl-xL ratio we evaluated the regulatory effect of LYC on apoptotic pathways. It was found that the activation of BAX promoted apoptosis, whereas Bcl-xL inhibited this process.
The WB results revealed that p-PI3K and p-AKT levels were decreased in the LPS + LYC group, highlighting the downregulation of the PI3K/AKT pathway. This indicated that LYC significantly inhibited LPS-induced phosphorylation of PI3K and AKT, while also regulating the expression of Bcl-xL downstream of this pathway. As a downstream gene of the PI3K/AKT pathway, BCL2L1 can encode Bcl-xL, thus playing a crucial role in inhibiting cell apoptosis by suppressing various apoptotic signaling pathways to maintain cell survival. Bcl-xL is an antiapoptotic protein, while BAX is a proapoptotic protein. In the LPS-treated group, the Bcl-xL:BAX ratio decreased, whereas this ratio increased in the LPS + LYC group, suggesting that LYC can reduce the apoptotic pressure induced by inflammation. This effect may be achieved through the antioxidant properties of LYC or its direct regulation of relevant protein expression. Similar results were reported by Li et al., who found that LYC upregulated the expression of Bcl-2, downregulated the expression of BAX, and inhibited the cleavage forms of caspase-9 and caspase-3, ultimately alleviating the apoptosis in the mitochondrial pathway induced by aristolochic acid I (AAI) (9). LYC can activate antioxidant signaling pathways to maintain renal oxidative stress homeostasis, ultimately reducing renal inflammatory responses and cell apoptosis (13). In our study, the molecular docking results showed that LYC bound well with such key targets as PIK3CD, AKT1, and PIK3R1, further validating the accuracy of active ingredient and target selection through network pharmacology.
The combination of network pharmacology and experimental validation provides a comprehensive perspective for understanding the multitarget mechanism of action in LYC-based therapy. Moreover, the importance of simultaneously targeting inflammation and apoptosis for achieving favorable outcomes in the treatment of epididymitis was supported by our findings. The ability of LYC to regulate the PI3K/AKT pathway makes it a promising candidate for further clinical research.
Despite the positive results obtained in this study, some limitations should be noted, Firstly, the sample size in our in vivo experiments was relatively small, which may limit the generalizability of the findings. Additional studies with larger sample sizes are recommended to confirm these results. Additionally, the use of a single animal model (LPS-induced epididymitis in mice) may not fully replicate the complexity of human epididymitis. Future research should consider using multiple animal models and eventually clinical trials to validate the therapeutic effects of LYC in humans. Lastly, while molecular docking provides valuable predictions about binding affinities, it does not fully capture the complexity of in vivo interactions, such as conformational changes and metabolic factors. Thus, further studies are necessary to validate these interactions under physiological conditions.
Conclusions
The potential mechanisms of LYC in the treatment of epididymitis were investigated through network pharmacology, molecular docking techniques, and in vivo experiments. The results demonstrated that LYC may exert its anti-inflammatory effects by modulating key pathways such as PI3K/AKT signaling pathway. These findings suggest that LYC could serve as a promising adjunctive therapy for epididymitis, offering anti-inflammatory and anti-apoptotic benefits that complement conventional antibiotic treatments. LYC may be particularly useful in cases where antibiotic resistance is a concern or when patients have an incomplete response to standard therapies. Future studies should focus on validating the efficacy and safety of LYC in human clinical trials. Additionally, exploring combination therapies with antibiotics could enhance treatment effectiveness and broaden the clinical application of LYC in managing epididymitis.
Acknowledgments
Funding: This work was supported by
Footnote
Reporting Checklist: The authors have completed the ARRIVE reporting checklist. Available at https://tau.amegroups.com/article/view/10.21037/tau-24-567/rc
Data Sharing Statement: Available at https://tau.amegroups.com/article/view/10.21037/tau-24-567/dss
Peer Review File: Available at https://tau.amegroups.com/article/view/10.21037/tau-24-567/prf
Conflicts of Interest: All authors have completed the ICMJE uniform disclosure form (available at https://tau.amegroups.com/article/view/10.21037/tau-24-567/coif). All authors report that this work was supported by the National Natural Science Foundation of China (Nos. 81771646 and 2018YFC1004300). The authors have no other conflicts of interest to declare.
Ethical Statement: The authors are accountable for all aspects of the work in ensuring that questions related to the accuracy or integrity of any part of the work are appropriately investigated and resolved. The study was conducted in accordance with the Declaration of Helsinki (as revised in 2013). All animal experiments were approved by the Animal Experiment Ethics Committee of the Eastern Theater General Hospital (approval No. DZGZRDW2400120) and GemPharmatech Co., Ltd. (approval No. GPTAP20231225-1), in accordance with national guidelines for the care and use of animals in research.
Open Access Statement: This is an Open Access article distributed in accordance with the Creative Commons Attribution-NonCommercial-NoDerivs 4.0 International License (CC BY-NC-ND 4.0), which permits the non-commercial replication and distribution of the article with the strict proviso that no changes or edits are made and the original work is properly cited (including links to both the formal publication through the relevant DOI and the license). See: https://creativecommons.org/licenses/by-nc-nd/4.0/.
References
- Andrade AD, Almeida PGC, Mariani NAP, et al. Regional modulation of toll-like receptor signaling pathway genes in acute epididymitis in mice. Andrology 2024;12:1024-37. [Crossref] [PubMed]
- Trojian TH, Lishnak TS, Heiman D. Epididymitis and orchitis: an overview. Am Fam Physician 2009;79:583-7.
- Tracy CR, Steers WD, Costabile R. Diagnosis and management of epididymitis. Urol Clin North Am 2008;35:101-8. vii. [Crossref] [PubMed]
- Ludwig M, Johannes S, Bergmann M, et al. Experimental Escherichia coli epididymitis in rats: a model to assess the outcome of antibiotic treatment. BJU Int 2002;90:933-8. [Crossref] [PubMed]
- Klein B, Pant S, Bhushan S, et al. Dexamethasone improves therapeutic outcomes in a preclinical bacterial epididymitis mouse model. Hum Reprod 2019;34:1195-205. [Crossref] [PubMed]
- Gao X, Lin B, Chen C, et al. Lycopene from tomatoes and tomato products exerts renoprotective effects by ameliorating oxidative stress, apoptosis, pyroptosis, fibrosis, and inflammatory injury in calcium oxalate nephrolithiasis: the underlying mechanisms. Food Funct 2024;15:4021-36. [Crossref] [PubMed]
- Jiang FW, Guo JY, Lin J, et al. MAPK/NF-κB signaling mediates atrazine-induced cardiorenal syndrome and antagonism of lycopene. Sci Total Environ 2024;922:171015. [Crossref] [PubMed]
- Imran M, Ghorat F, Ul-Haq I, et al. Lycopene as a Natural Antioxidant Used to Prevent Human Health Disorders. Antioxidants (Basel) 2020;9:706. [Crossref] [PubMed]
- Li Y, Zhu J, Zhao X, et al. Oral Lycopene Administration Attenuates Inflammation and Oxidative Stress by Regulating Plasma Lipids in Rats with Lipopolysaccharide-Induced Epididymitis. J Inflamm Res 2022;15:6517-31. [Crossref] [PubMed]
- Raj MV, Selvakumar K, Krishnamoorthy G, et al. Impact of lycopene on epididymal androgen and estrogen receptors' expression in polychlorinated biphenyls-exposed rat. Reprod Sci 2014;21:89-101. [Crossref] [PubMed]
- Hopkins AL. Network pharmacology: the next paradigm in drug discovery. Nat Chem Biol 2008;4:682-90. [Crossref] [PubMed]
- Nogales C, Mamdouh ZM, List M, et al. Network pharmacology: curing causal mechanisms instead of treating symptoms. Trends Pharmacol Sci 2022;43:136-50. [Crossref] [PubMed]
- Liu JC, Wang P, Zeng QX, et al. Myd88 Signaling Is Involved in the Inflammatory Response in LPS-Induced Mouse Epididymitis and Bone-Marrow-Derived Dendritic Cells. Int J Mol Sci 2023;24:7838. [Crossref] [PubMed]
- Wang Y, Liu Z, Ma J, et al. Lycopene attenuates the inflammation and apoptosis in aristolochic acid nephropathy by targeting the Nrf2 antioxidant system. Redox Biol 2022;57:102494. [Crossref] [PubMed]
- Mallik MK. An attempt to understand glioma stem cell biology through centrality analysis of a protein interaction network. J Theor Biol 2018;438:78-91. [Crossref] [PubMed]
- Wang X, Kong C, Liu P, et al. Therapeutic Effects of Retinoic Acid in Lipopolysaccharide-Induced Cardiac Dysfunction: Network Pharmacology and Experimental Validation. J Inflamm Res 2022;15:4963-79. [Crossref] [PubMed]
- Rusz A, Pilatz A, Wagenlehner F, et al. Influence of urogenital infections and inflammation on semen quality and male fertility. World J Urol 2012;30:23-30. [Crossref] [PubMed]
- Eickhoff JH, Frimodt-Møller N, Walter S, et al. A double-blind, randomized, controlled multicentre study to compare the efficacy of ciprofloxacin with pivampicillin as oral therapy for epididymitis in men over 40 years of age. BJU Int 1999;84:827-34. [Crossref] [PubMed]
- Michel V, Duan Y, Stoschek E, et al. Uropathogenic Escherichia coli causes fibrotic remodelling of the epididymis. J Pathol 2016;240:15-24. [Crossref] [PubMed]
- McConaghy JR, Panchal B. Epididymitis: An Overview. Am Fam Physician 2016;94:723-6.
- Boleken ME, Kaya M, Zeyrek F, et al. Effects of acute epididymitis on blood plasma oxidative and antioxidative status in an experimental rat model. Urol Int 2008;81:275-8. [Crossref] [PubMed]
- Kaya M, Boleken ME, Zeyrek F, et al. Oxidative and antioxidative status in the testes of rats with acute epididymitis. Urol Int 2006;76:353-8. [Crossref] [PubMed]
- Khalaf RA, Awad M. Lycopene as a Potential Bioactive Compound: Chemistry, Extraction, and Anticancer Prospective. Curr Cancer Drug Targets 2023;23:634-42. [Crossref] [PubMed]
- Guo Q, Li F, Duan Y, et al. Oxidative stress, nutritional antioxidants and beyond. Sci China Life Sci 2020;63:866-74. [Crossref] [PubMed]
- Liu Y, Gong S, Li K, et al. Coptisine protects against hyperuricemic nephropathy through alleviating inflammation, oxidative stress and mitochondrial apoptosis via PI3K/Akt signaling pathway. Biomed Pharmacother 2022;156:113941. [Crossref] [PubMed]
- Geng H, Chen J, Tu K, et al. Carbon dot nanozymes as free radicals scavengers for the management of hepatic ischemia-reperfusion injury by regulating the liver inflammatory network and inhibiting apoptosis. J Nanobiotechnology 2023;21:500. [Crossref] [PubMed]
(English Language Editor: J. Gray)