Anti-cancer role of curcumin in prostate cancer cells via regulation of m6A-modified circ0030568-FMR1 signaling pathway
Highlight box
Key findings
• This study found that curcumin suppresses prostate cancer (PCa) cells by inhibiting YTHDF2 mediated circ0030568 stability.
What is known and what is new?
• N6-methyladenosine (m6A) modification has been shown to regulate PCa progression and curcumin possesses an anti-PCa effect.
• Circ0030568 may act as a promising biomarker and an attractive target for PCa treatment and curcumin could suppress the proliferation and migration of PCa cells by inhibiting m6A-modified circ0030568.
What is the implication, and what should change now?
• Targeting m6A-modified circRNAs may be a potential treatment for PCa.
Introduction
Prostate cancer (PCa), a hormone-dependent disease, remains a leading cause of mortality worldwide (1). PCa is characterized by increased expression of critical enzymes of the lipogenic pathway (2). Moreover, the androgen receptor signaling pathway is the main cause of castration-resistant PCa (3). Although androgen deprivation therapy (ADT) can effectively inhibit the progression of PCa, resistance of PCa cells to ADT can aggravate tumor metastasis. Nevertheless, current therapies for PCa are still limited and thus lead to poor prognosis and quality-of-life. Hence, novel therapeutic targets and molecular mechanisms of PCa need to be further investigated.
Circular RNAs (circRNAs) are originated from the process of RNA transcription fragments (4). CircRNAs exert its regulatory effects on diverse cellular physiological and pathological processes (5). It has been proven that circRNAs are implicated in regulation of tumor-related genes via functioning in protein-coding RNAs or competitive endogenous RNAs (6). Although the role of circRNAs in PCa has been widely confirmed in recent years (7,8), the mechanism of regulating circRNAs and thus anti-PCa is still poorly understood. N6-methyladenosine (m6A) is methylation that exerts vital effects on mRNA stability, nuclear export, and microRNA processing (9-11). M6A modification is a dynamic and reversible process regulated by methylases (“writers”) and demethylases (“erasers”) (12,13). Consequently, m6A methylation is recognized by binding protein “readers”, such as YTH domain family proteins, insulin-like growth factor 2 mRNA-binding proteins (IGF2BPs), and heterogeneous nuclear ribonucleoprotein (HNRNP) family proteins (14). As m6A modification regulators, METTL3 and YTHDF2 have been reported to be frequently upregulated in PCa tissues and cell lines, suggesting that m6A modification are associated with PCa progression (15,16). However, the specific biological role of m6A in PCa has not been rigorously investigated. Thus, we aimed to investigate the role of m6A-modified circRNAs in PCa and further explore new pharmaceutical strategies for PCa treatment.
Natural herbal products can also be considered as PCa preventing agents. Curcumin, the crucial yellow pigment and spice in turmeric and curry, has important anti-inflammatory and antioxidant properties (17-19), which is also reported to be a powerful anti-cancer agent (20,21). Numerous studies clarify that curcumin is capable to inhibit the development, occurrence, radiotherapy and chemotherapy resistance of various malignant tumors by modulating circRNAs or miRNAs (22,23). Although the inhibitory effect of curcumin on PCa progression has been widely established (24), whether curcumin can suppress PCa progression through the m6A-modified circRNAs remains unclear.
In summary, our study found that the level of circ0030568 with m6A modification and expression of circ0030568 were significantly increased in PCa cells. YTHDF2 mediated m6A modification increased the stability of circ0030568 and increased circ0030568 promote the proliferation and migration of PCa cells by activating the oncogene FMR1. Meanwhile, curcumin could suppress PCa progression by inhibiting YTHDF2 mediated circ0030568 expression. Our study aimed to propose novel markers and therapeutic targets for the treatment of PCa. We present this article in accordance with the MDAR reporting checklist (available at https://tau.amegroups.com/article/view/10.21037/tau-24-276/rc).
Methods
Cell culture and transfection
Human normal prostatic matrix immortalized cells (WPMY-1) and PCa cell lines (PC3 and DU145) used in this study were obtained from American Type Culture Collection (ATCC). Cells above were cultured in RPMI-1640 medium (Invitrogen, California, USA; 11875085) supplemented with 10% fetal bovine serum (FBS, Gibco, New York, USA; A5256701), 100 U/mL penicillin and 100 µg/mL streptomycin (Invitrogen, 15140122) with a humidified atmosphere of 5% CO2 at 37 ℃. Cell transfections were carried out and relative segments were transfected into PCa cells that were inoculated in 6-well plates with 80% confluence using Lipofectamine 3000 (Invitrogen, L3000008).
Western blotting
Proteins were extracted using Radio Immunoprecipitation Assay Lysis buffer (RIPA, Beyotime, Shanghai, China) buffer containing protease inhibitor phenylmethylsulfonyl fluoride (PMSF) (Beyotime) on ice and subsequently quantified with a BCA Protein Assay kit (Beyotime, P0012). Then, the extracted proteins were separated on 10% SDS-PAGE gels and transferred to polyvinylidene fluoride (PVDF) membranes (Millipore, Massachusetts, USA). The membranes were blocked with 5% skim milk and incubated with the indicated primary antibodies at 4 ℃ overnight (Supporting Information in Table S1). After washing with TBST, membranes were incubated secondary antibodies at room temperature for 1 h. The blots were developed via the Pierce ECL Western Blotting Substrate.
RNA quantitative real-time polymerase chain reaction (qRT-PCR)
Trizol reagent (Invitrogen) was used to isolate total RNA from cells. Then, RNA was subjected to reverse transcription with Superscript III transcriptase (Invitrogen, 18080093). qRT-PCR was carried out using SYBR Green Master Mix (Bio-Rad, California, USA) on an ABI 7500 Real-Time PCR System (Applied Biosystems). qRT-qPCR was employed to detect the expression of circRNAs in different PCa cell lines and control cell lines and heatmaps were generated based on qRT-PCR values. The results were normalized to the expression of Actin. The primer sequences used are provided in Table S2.
RNA immunoprecipitation (RIP)
RIP assay was conducted using a Magna RIP RNA-Binding Protein Immunoprecipitation Kit (Millipore, No. 17-700) in accordance with the manufacturer’s protocol. Cells were harvested and lysed with RIP lysis buffer on ice. Then, the cell lysates were incubated with Protein A/G agarose beads and antibodies at 4 ℃ overnight. The protein-RNA complexes were washed with RIP washing buffer and digested with proteinase K. The immunoprecipitated RNA was determined by qRT-PCR analysis.
Methylated RIP (MeRIP)
Total RNA from cells was extracted with Trizol (Invitrogen, A33251). Then, RNA was incubated with magnetic beads (Millipore) and anti-m6A antibody or anti-IgG. After washing with elution buffer, the antibody-coated beads were digested with proteinase K. Phenolchloroform RNA extraction methods were used to precipitate m6A-bound RNA. m6A enrichment was determined by qRT-PCR analysis and normalized to the input.
Fluorescence in situ hybridization (FISH) analysis
To identify the colocalization of has_circ_0030568 in the cells, we conducted the RNA FISH experiment. The probes labeled with CY-3 for has_circ_0030568 detection and the FISH experiment was conducted using Fluorescent in situ Hybridization Kit (No. C10910, RiboBio, Guangzhou, China), according to the standard guidelines (25). DAPI was utilized to stain the nuclei. Subsequently, has_circ_0030568 was observed through a confocal microscope (LSM 880 with Airyscan, Carl Zeiss, Germany).
Actinomycin D and RNase R treatment
Cells were seeded into six-well plates. Then, cells were exposed to actinomycin D (5 µg/mL) and total RNA was collected at indicated time points. For RNase R treatment, total RNA was treated with RNase R (3 U/µg) at 37 ℃. After treatment with actinomycin D or RNase R, the RNA expression levels were determined by qRT-PCR analysis.
Nuclear and cytoplasmic extraction
Nuclear and cytoplasmic fractions were isolated using Nuclear and Cytoplasmic Protein Extraction Kit (Beyotime, P0028) as previously described (26). The levels of RNA were assessed by qRT-PCR analysis using SYBR Green Master Mix. The results were normalized to the expression of Actin.
RNA pull down
For RNA pull down assays, cells were lysed in lysis buffer. Biotinylated probe or negative control probe were added to streptavidin-coated magnetic beads (Invitrogen). Then, the cell lysates were incubated with streptavidin-coated magnetic beads bound with biotinylated probe or negative control probe at 4 ℃ with rotation. After washing with NT2 buffer, the pulled-down proteins were collected and identified by western blotting.
CCK-8 assay
The viability of cells was detected using the Cell Counting Kit-8 assay (Dojindo, Kyushu, Japan; CK04) in accordance with the manufacturer’s instructions. 6-well plates were seeded with cells. Then, the cells were incubated with CCK-8 reagent and cultured for different time periods. The absorbance of each well was assessed at OD 450 nm.
Wound healing assay
For wound healing assay, cells were cultured in 6-well plates. Scratch wounds were created with a 10 µL pipette tips. After washing with PBS two times, images of migrated cells were acquired under microscopy at 0 and 24 h.
Statistical analysis
GraphPad Prism 8.0 was used for Statistical analysis. Student’s t test was used to test the significance of differences in two grouped data, and one-way analysis of variance (ANOVA) was applied to three or more independent groups. All experiments were independently performed for three times. Representative data were expressed as mean ± standard error of mean (SEM). P<0.05 was considered as statistically significant.
Results
Characterization of upregulated circ0030568 in PCa cells
Based on the circRNAs that have been reported to be associated with PCa in the literature, we first detected the m6A modification levels of circRNAs in two PCa cell lines. As shown in the heat map in Figure 1A, the m6A modification levels of has_circ_0030568 were significantly elevated in both PC3 and DU145 cells, which was also confirmed by the results of MeRIP-qPCR experiments (Figure 1B). Furthermore, qRT-PCR showed that circ_0030568 was remarkedly upregulated in PCa cells (Figure 1C). By re-analyzing the GSE179321 dataset in the GEO database, we observed that more than 10,000 circRNAs were highly expressed in PCa tissues, as shown in the volcano map results (Figure 1D). The elevated level of hsa_circ_0030568 was also confirmed by GSE179321 dataset (Figure 1E). Hence, circ0030568 was identified as the circRNA of interest and selected to explore its critical role in PCa. Next, circ0030568 was sequenced in PCa cells with high conservation according to circBase (Figure 1F). Moreover, searching from University of California Santa Cruz (UCSC), we confirmed that the ABCC4 was the host gene of circ0030568 and circ0030568 generation from exon 22 to 29 of the ABCC4 gene (Figure 1G). Noticeably, resistance to digestion with RNase R exonuclease confirmed that circ0030568 was resistant to RNase R, whereas linear ABCC4 mRNA was easily degraded (Figure 1H). Finally, qRT-PCR analysis of nuclear and cytoplasmic fractions and FISH further revealed that circ0030568 is mainly localized to the cytoplasm of PC3 and DU145 cells (Figure 1I-1K). These results, collectively, indicated that circ0030568 is an abundant and stable circRNA expressed in PCa cells and may exert an important role in regulating PCa progression.
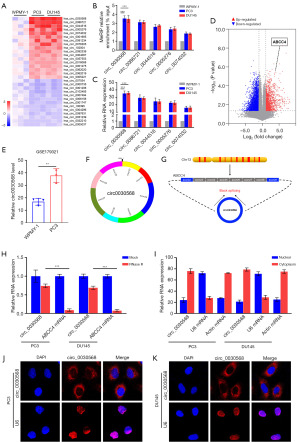
Circ0030568 promotes PCa cells proliferation and migration
To evaluate the biological functions of circ0030568 in PCa cells, we first constructed circ0030568 knockdown or overexpressed PC3 (Figure 2A,2B) and DU145 cells (Figure 2C,2D). Additionally, we found that knockdown or overexpression of circ0030568 had no effect on the expression of the host gene, ABCC4, suggesting that the regulatory effect on PC directly results from circ0030568. Interestingly, the CCK-8 assays showed that knockdown of circ0030568 effectively inhibited the proliferation of PC-3 and DU145 cells at 24, 48, 72, and 96 h, whereas PCa cells transfected with circ0030568 OE plasmid showed significant proliferation (Figure 2E,2F). Consistently, the results of wound healing assays demonstrated that after knockdown of circ0030568, PC3 and DU145 cells migration were both significantly inhibited compared to that of control cells. In contrast, overexpressing circ0030568 had remarkably increased migration in the PCa cells compared to controls (Figure 2G,2H). Taken together, these results confirmed that elevated circ0030568 promotes PCa cells proliferation and migration.
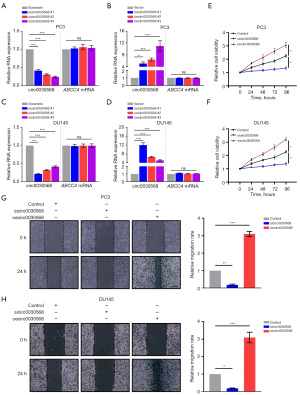
Curcumin inhibits PCa cells proliferation and migration by downregulating circ0030568
Next, we aimed to explore the role of m6A-mediated inhibitory effectiveness of curcumin on PCa. The chemical structure of curcumin is shown in Figure 3A. Circ0030568 levels were detected in two PCa cells (PC3 and DU145) treated with or without curcumin to investigate whether curcumin could inhibit circ0030568 expression in PCa cells. The results showed that 10 µm curcumin could effectively suppress the level of circ0030568 both in PC3 (Figure 3B) and DU145 cells (Figure 3C). As shown in Figure 3D,3E, we found that curcumin effectively inhibited circ0030568 expression, but not the other four upregulated circRNAs in PCa cells. Meantime, CCK-8 and wound healing assays revealed that curcumin treatment could inhibit the proliferation (Figure 3F,3G) and migration (Figure 3H,3I) of PC3 and DU145 cells. Thus, we selected circ0030568 as the potential target of curcumin in the further experiments.
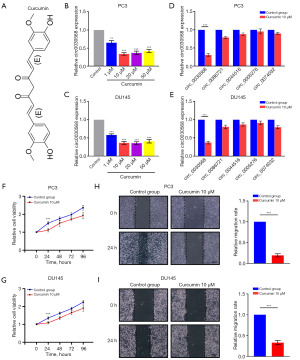
METTL3 mediates m6A modification of circ0030568
Studies have shown that m6A modification is implicated in all aspects of posttranscriptional RNA metabolism, including mRNA localization, splicing, translation, and degradation, which in turn regulates important biological processes (27,28). To test whether circ0030568 is modulated by m6A, we used SRAMP (Figure 4A) and RMBase 2.0 (Figure 4B) to predict potential m6A sites in circ0030568 and found one m6A site shared by the two programs. It is possible that m6A modification plays an important role in the regulation of circ0030568 during the occurrence and development of PCa. METTL3 is a predominant methyltransferase for m6A modification and plays an oncogenic role in tumor formation and progression (29-31). As the crucial m6A writer, METTL3 was also upregulated in The Cancer Genome Atlas (TCGA) PCa tissues (n=498) compared to the normal controls (n=52) (P<0.001) (Figure 4C). We performed RNA binding protein immunoprecipitation (RIP) analysis and proved that compared with the control IgG, METTL3 antibody-precipitated complexes enriched the expression of circ0030568, while METTL14 could not enrich circ0030568 (Figure 4D,4E). We performed an RNA pull-down experiment to further verify the combination of METTL3 and circ0030568 (Figure 4F,4G). Moreover, the results of western blotting showed that METTL3 expression obviously upregulates in both two PCa cells, suggesting that METTL3 mediated m6A modification of circ0030568 plays a critical role in PCa (Figure 4H). We knocked down METTL3 in PC3 (Figure 4I-4K) and DU145 (Figure 4L-4N) cells by constructing siRNA and results showed that METTL3 suppression could reduce circ0030568 expression. In conclusion, these above results confirmed that METTL3 mediates m6A modification of circ0030568 in PCa.
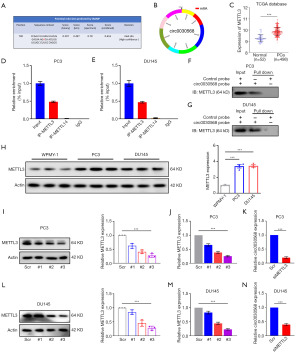
YTHDF2 upregulates circ0030568 expression by increasing its stability
Given that m6A modification of circ0030568 has been shown to play an important role in PCa, we next aimed to explore whether the m6A reader proteins can regulate the expression of circ0030568. Simultaneously, it has been extensively demonstrated that YTHDF2 regulates the stability of m6A-modified RNAs, including noncoding RNAs (16,32,33). Upregulation of YTHDF2 was also observed in TCGA PCa tissues (n=498) compared with the normal controls (n=52) (P<0.001) (Figure 5A). YTHDF2 has a domain that recognizes and binds m6A modification sites (Figure 5B). RNA pull-down assays demonstrated that YTHDF2 and circ0030568 have significant binding and interaction in PC3 (Figure 5C) and DU145 cells (Figure 5D). As shown in Figure 5E, the protein expression level of YTHDF2 was significantly increased in PC3 and DU145 cells compared with the control group. We further constructed YTHDF2 knockdown or overexpressed PC3 (Figure 5F,5G) and DU145 cells (Figure 5H-5J). The results of qRT-PCR demonstrated that after knockdown of YTHDF2, circ0030568 expression markedly decreased in PC3 and DU145 cells compared to that of control cells (Figure 5K). On the contrary, YTHDF2 overexpression significantly increased the level of circ0030568 in the PCa cells. Interestingly, we found that curcumin treatment significantly decreased YTHDF2 expression both in PC3 and DU145 cells (Figure 5L). In addition, YTHDF2 inhibition could significantly reduce circ0030568 expression, while YTHDF2 overexpression could effectively restore circ0030568 expression level reduced by curcumin treatment (Figure 5M). Collectively, these results indicated that YTHDF2 upregulates circ0030568 expression by increasing its stability and curcumin downregulates circ0030568 expression by inhibiting YTHDF2.
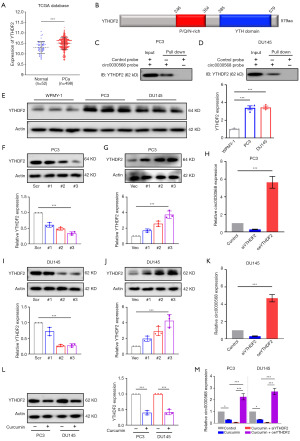
Curcumin inhibits PCa progression via regulation of m6A-modified circ0030568-FMR1 signaling pathway
As depicted in Figure 1 above, the nuclear and cytoplasmic extraction assays yielded results and FISH findings confirmed that circ0030568 stayed in the cytoplasm at large in PC3 and DU145 cells. Concerning the predominance of the competing endogenous RNA (ceRNA) mechanism in noncoding RNAs (ncRNAs) in the cytoplasm, we conducted an AGO2-RIP experiment to reveal whether circ0030568 functions in a ceRNA manner and found that the anti-AGO2 antibody had no interaction with circ0030568, ruling out circ0030568’s claim to be a ceRNA (Figure 6A). We further wondered whether circ0030568 has the potential to encode protein. According to the results searched in circRNAdb database, circ0030568 was predicted to have a relative low possibility of encoding protein (Figure 6B). Subsequently, we turned to the RNA-binding proteins (RBPs) that interact with circ0030568 and harvested 15 candidates from catRAPID omics bioinformatics database (Figure 6C). Several lines of evidence have reported that FMR1 is closely related to tumorigenesis and development by acting as an oncogene in cancers, including PCa. In addition, the observations of RIP analysis were consistent with the above database prediction results (Figure 6D). Western blotting analysis confirmed that FMR1 protein levels elevated significantly in two PCa cells (Figure 6E). We next detected FMR1 expression in two PCa cells after silencing or overexpressing of circ0030568. It was uncovered that inhibition of circ0030568 suppresses FMR1 expression and overexpressing of circ0030568 increases FMR1 expression in PC3 (Figure 6F,6G) and DU145 cells (Figure 6H,6I). We further found that curcumin treatment significantly decreased FMR1 expression both in PC3 and DU145 cells (Figure 6J). In addition, circ0030568 inhibition could reduce FMR1 expression, while circ0030568 overexpression could restore FMR1 expression level reduced by curcumin treatment (Figure 6K). These data indicated that circ0030568 promotes PCa progression via upregulating FMR1 and curcumin could inhibit PCa via regulation of m6A-modified circ0030568-FMR1 pathway.
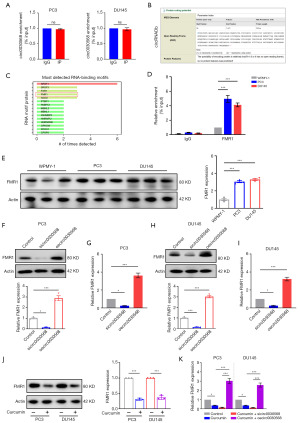
Discussion
PCa, a common heterogeneous disease, is one of the most frequent malignancies worldwide (34,35). Previous studies have uncovered PCa specimens with distant metastasis may contribute to androgen-independent PCa and hormone refractory PCa (36). At the same time, several trials have shown that metastasis of PCa is inevitable and significantly decreases the lifespan and quality of life of patients (37,38). Consequently, we focused on understanding the specific mechanisms that drive the oncogenesis of the tumors in PCa and aimed to propose novel markers and therapeutic targets for the treatment of PCa. By employing MeRIP-qPCR experiments, we selected several upregulated circRNAs characterized with significantly elevated m6A modification levels in different PCa cells. We further demonstrated that circ0030568 could promote PCa cells proliferation and migration, which perhaps act as a valuable target for the PCa treatment.
Many natural products with excellent pharmacological properties are reported to be good candidates for the prevention of cancers. As a natural polyphenol extracted from turmeric curry spice, curcumin has multiple biological properties and health benefits without corresponding toxicity. Studies have confirmed the favorable therapeutic effects of curcumin on a broad array of diseases, especially cancers (39,40). Some cutting-edge research has examined the effects of curcumin treatment on different PCa cells (41). Although these published data indicated that curcumin might also play a role in PCa progression, such a notion has not been fully clarified. As a natural compound, curcumin has been shown to inhibit PCa progression through multiple mechanisms (42,43). In particular, whether curcumin can participate in the progression of PCa by regulating the level of circRNAs remains unknown. Thus, we proposed a novel mechanism by which curcumin inhibits PCa progression by down-regulating m6A-modified circRNA, providing new potential ideas and strategies for the anti-tumor of traditional Chinese medicine.
CircRNAs, single-stranded RNA molecules, are regulators in the expressions of various genes (44). CircRNAs are involved in diverse biological processes, including cell proliferation, differentiation, and energy metabolism. Traditional studies have defined circRNAs as microRNA sponges and transcriptional regulators (45). Over the past decade, studies have confirmed that circRNAs possess essential effects on regulating the occurrence and development of PCa (46). For instance, circCSNK1G3 and circNOLC1 have been reported to promote PCa progression (47,48). Besides, downregulated circANKS1B inhibited the proliferation and metastasis of PCa cells via increasing TGF-α expression (49). In this study, we found that circ0030568 promoted the proliferation and migration of PCa cells by activating the oncogene FMR1, indicating that circ0030568 could be diagnostic biomarkers and therapeutic targets for PCa. However, the generation and source of circ0030568 and its potential mechanism in the progression of PCa need to be further explored.
M6A is the prevalent internal modification of RNAs in eukaryotes (50), influencing various aspects of RNA metabolism, such as mRNA splicing, mRNA translation efficiency, as well as alternative polyadenylation (51-53). Similarly, recent studies have demonstrated that m6A modifications can regulate the expression and function of circRNA, expanding the value of m6A modifications (25,54). In our study, we demonstrated that METTL3 cooperated with YTHDF2 to mediate m6A modification of circ0030568, promoting the progression of PCa. Besides, the role of m6A demethylases in m6A-modified circRNA-mediated PCa has been reported. FTO and ALKBH5 decrease in PCa tissue and knockdown of FTO or ALKBH5 promoted PCa cell invasion and metastasis, whereas overexpressing FTO or ALKBH5 could inhibit PCa. Recently, studies have increasingly recognized the regulatory role of m6A-modified circRNAs in PCa (54,55). Interestingly, our results also confirm that curcumin can treat PCa by inhibiting the expression level of YTHDF2 and thereby regulating the level of m6A-modified circRNAs. Our study found m6A-modified circ0030568 exerts important effects in PCa progression, providing insight into the novel mechanism in PCa and enhancing the efficacy of treatment in PCa.
Conclusions
In this study, we found that the level of circ0030568 with m6A modification and expression of circ0030568 were largely elevated in PCa cells. Moreover, METTL3 mediated m6A modification of circ0030568 in PCa and YTHDF2 upregulated the expression of circ0030568 by increasing its stability. Then, upregulated circ0030568 expression promoted the proliferation and migration of PCa cells via increasing the expression of FMR1. More importantly, curcumin could suppress PCa progression by inhibiting YTHDF2 mediated circ0030568 stability. Taken together, our study first disclosed circ0030568 as a potential therapeutic target in PCa and suggested curcumin’s inhibition of m6A-modified circ0030568 may be a potential mechanism of its anti-PCa (Figure 7).
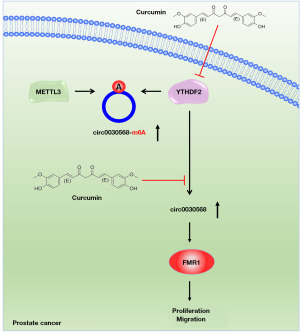
Acknowledgments
Funding: None.
Footnote
Reporting Checklist: The authors have completed the MDAR reporting checklist. Available at https://tau.amegroups.com/article/view/10.21037/tau-24-276/rc
Data Sharing Statement: Available at https://tau.amegroups.com/article/view/10.21037/tau-24-276/dss
Peer Review File: Available at https://tau.amegroups.com/article/view/10.21037/tau-24-276/prf
Conflicts of Interest: All authors have completed the ICMJE uniform disclosure form (available at https://tau.amegroups.com/article/view/10.21037/tau-24-276/coif). The authors have no conflicts of interest to declare.
Ethical Statement: The authors are accountable for all aspects of the work in ensuring that questions related to the accuracy or integrity of any part of the work are appropriately investigated and resolved.
Open Access Statement: This is an Open Access article distributed in accordance with the Creative Commons Attribution-NonCommercial-NoDerivs 4.0 International License (CC BY-NC-ND 4.0), which permits the non-commercial replication and distribution of the article with the strict proviso that no changes or edits are made and the original work is properly cited (including links to both the formal publication through the relevant DOI and the license). See: https://creativecommons.org/licenses/by-nc-nd/4.0/.
References
- Shafi AA, Yen AE, Weigel NL. Androgen receptors in hormone-dependent and castration-resistant prostate cancer. Pharmacol Ther 2013;140:223-38. [Crossref] [PubMed]
- Labanca E, Bizzotto J, Sanchis P, et al. Prostate cancer castrate resistant progression usage of non-canonical androgen receptor signaling and ketone body fuel. Oncogene 2021;40:6284-98. [Crossref] [PubMed]
- Grasso CS, Cani AK, Hovelson DH, et al. Integrative molecular profiling of routine clinical prostate cancer specimens. Ann Oncol 2015;26:1110-8. [Crossref] [PubMed]
- Dragomir M, Calin GA. Circular RNAs in Cancer - Lessons Learned From microRNAs. Front Oncol 2018;8:179. [Crossref] [PubMed]
- Li H, Xu JD, Fang XH, et al. Circular RNA circRNA_000203 aggravates cardiac hypertrophy via suppressing miR-26b-5p and miR-140-3p binding to Gata4. Cardiovasc Res 2020;116:1323-34. [Crossref] [PubMed]
- Chen LL. The expanding regulatory mechanisms and cellular functions of circular RNAs. Nat Rev Mol Cell Biol 2020;21:475-90. [Crossref] [PubMed]
- Yu YZ, Lv DJ, Wang C, et al. Hsa_circ_0003258 promotes prostate cancer metastasis by complexing with IGF2BP3 and sponging miR-653-5p. Mol Cancer 2022;21:12. [Crossref] [PubMed]
- Wei Z, Zhang C, Song Y, et al. CircUBE3A(2,3,4,5) promotes adenylate-uridylate-rich binding factor 1 nuclear translocation to suppress prostate cancer metastasis. Cancer Lett 2024;588:216743. [Crossref] [PubMed]
- Zhang C, Fu J, Zhou Y. A Review in Research Progress Concerning m6A Methylation and Immunoregulation. Front Immunol 2019;10:922. [Crossref] [PubMed]
- Alarcón CR, Lee H, Goodarzi H, et al. N6-methyladenosine marks primary microRNAs for processing. Nature 2015;519:482-5. [Crossref] [PubMed]
- Lan Q, Liu PY, Bell JL, et al. The Emerging Roles of RNA m(6)A Methylation and Demethylation as Critical Regulators of Tumorigenesis, Drug Sensitivity, and Resistance. Cancer Res 2021;81:3431-40. [Crossref] [PubMed]
- He PC, He C. m(6) A RNA methylation: from mechanisms to therapeutic potential. EMBO J 2021;40:e105977. [Crossref] [PubMed]
- Yu J, Chen M, Huang H, et al. Dynamic m6A modification regulates local translation of mRNA in axons. Nucleic Acids Res 2018;46:1412-23. [Crossref] [PubMed]
- Zhao Y, Shi Y, Shen H, et al. m(6)A-binding proteins: the emerging crucial performers in epigenetics. J Hematol Oncol 2020;13:35. [Crossref] [PubMed]
- An Y, Duan H. The role of m6A RNA methylation in cancer metabolism. Mol Cancer 2022;21:14. [Crossref] [PubMed]
- Li J, Xie H, Ying Y, et al. YTHDF2 mediates the mRNA degradation of the tumor suppressors to induce AKT phosphorylation in N6-methyladenosine-dependent way in prostate cancer. Mol Cancer 2020;19:152. [Crossref] [PubMed]
- Perugini J, Di Mercurio E, Tossetta G, et al. Biological Effects of Ciliary Neurotrophic Factor on hMADS Adipocytes. Front Endocrinol (Lausanne) 2019;10:768. [Crossref] [PubMed]
- Tossetta G, Fantone S, Busilacchi EM, et al. Modulation of matrix metalloproteases by ciliary neurotrophic factor in human placental development. Cell Tissue Res 2022;390:113-29. [Crossref] [PubMed]
- Tossetta G, Fantone S, Marzioni D, et al. Role of Natural and Synthetic Compounds in Modulating NRF2/KEAP1 Signaling Pathway in Prostate Cancer. Cancers (Basel) 2023;15:3037. [Crossref] [PubMed]
- Xie L, Ji X, Zhang Q, et al. Curcumin combined with photodynamic therapy, promising therapies for the treatment of cancer. Biomed Pharmacother 2022;146:112567. [Crossref] [PubMed]
- Liu C, Rokavec M, Huang Z, et al. Curcumin activates a ROS/KEAP1/NRF2/miR-34a/b/c cascade to suppress colorectal cancer metastasis. Cell Death Differ 2023;30:1771-85. [Crossref] [PubMed]
- Si L, Zhang L, Xing S, et al. Curcumin as a therapeutic agent in cancer therapy: Focusing on its modulatory effects on circular RNAs. Phytother Res 2023;37:3121-34. [Crossref] [PubMed]
- Xu X, Zhang X, Zhang Y, et al. Curcumin suppresses the malignancy of non-small cell lung cancer by modulating the circ-PRKCA/miR-384/ITGB1 pathway. Biomed Pharmacother 2021;138:111439. [Crossref] [PubMed]
- Rutz J, Benchellal A, Kassabra W, et al. Growth, Proliferation and Metastasis of Prostate Cancer Cells Is Blocked by Low-Dose Curcumin in Combination with Light Irradiation. Int J Mol Sci 2021;22:9966. [Crossref] [PubMed]
- Du A, Li S, Zhou Y, et al. M6A-mediated upregulation of circMDK promotes tumorigenesis and acts as a nanotherapeutic target in hepatocellular carcinoma. Mol Cancer 2022;21:109. [Crossref] [PubMed]
- Lo Piccolo L, Bonaccorso R, Onorati MC. Nuclear and Cytoplasmic Soluble Proteins Extraction from a Small Quantity of Drosophila’s Whole Larvae and Tissues. Int J Mol Sci 2015;16:12360-7. [Crossref] [PubMed]
- Meyer KD, Jaffrey SR. The dynamic epitranscriptome: N6-methyladenosine and gene expression control. Nat Rev Mol Cell Biol 2014;15:313-26. [Crossref] [PubMed]
- Akgül B, Akçaöz-Alasar A, Sağlam B. RNA m(6)A methylation at the juxtaposition of apoptosis and RNA therapeutics. Trends Cell Biol 2024;34:801-4. [Crossref] [PubMed]
- Li T, Hu PS, Zuo Z, et al. METTL3 facilitates tumor progression via an m(6)A-IGF2BP2-dependent mechanism in colorectal carcinoma. Mol Cancer 2019;18:112. [Crossref] [PubMed]
- Han J, Wang JZ, Yang X, et al. METTL3 promote tumor proliferation of bladder cancer by accelerating pri-miR221/222 maturation in m6A-dependent manner. Mol Cancer 2019;18:110. [Crossref] [PubMed]
- Xiong J, He J, Zhu J, et al. Lactylation-driven METTL3-mediated RNA m(6)A modification promotes immunosuppression of tumor-infiltrating myeloid cells. Mol Cell 2022;82:1660-1677.e10. [Crossref] [PubMed]
- Zhang C, Huang S, Zhuang H, et al. YTHDF2 promotes the liver cancer stem cell phenotype and cancer metastasis by regulating OCT4 expression via m6A RNA methylation. Oncogene 2020;39:4507-18. [Crossref] [PubMed]
- Chen YG, Chen R, Ahmad S, et al. N6-Methyladenosine Modification Controls Circular RNA Immunity. Mol Cell 2019;76:96-109.e9. [Crossref] [PubMed]
- Fabris L, Ceder Y, Chinnaiyan AM, et al. The Potential of MicroRNAs as Prostate Cancer Biomarkers. Eur Urol 2016;70:312-22. [Crossref] [PubMed]
- Di Carlo E, Sorrentino C. The multifaceted role of the stroma in the healthy prostate and prostate cancer. J Transl Med 2024;22:825. [Crossref] [PubMed]
- Traish AM, Morgentaler A. Epidermal growth factor receptor expression escapes androgen regulation in prostate cancer: a potential molecular switch for tumour growth. Br J Cancer 2009;101:1949-56. [Crossref] [PubMed]
- Haffner MC, Zwart W, Roudier MP, et al. Genomic and phenotypic heterogeneity in prostate cancer. Nat Rev Urol 2021;18:79-92. [Crossref] [PubMed]
- Lv DJ, Song XL, Huang B, et al. HMGB1 Promotes Prostate Cancer Development and Metastasis by Interacting with Brahma-Related Gene 1 and Activating the Akt Signaling Pathway. Theranostics 2019;9:5166-82. [Crossref] [PubMed]
- Wang W, Li M, Wang L, et al. Curcumin in cancer therapy: Exploring molecular mechanisms and overcoming clinical challenges. Cancer Lett 2023;570:216332. [Crossref] [PubMed]
- Liu X, Qi M, Li X, et al. Curcumin: a natural organic component that plays a multi-faceted role in ovarian cancer. J Ovarian Res 2023;16:47. [Crossref] [PubMed]
- Mirzaei A, Jahanshahi F, Khatami F, et al. Human prostate cancer cell epithelial-to-mesenchymal transition as a novel target of arsenic trioxide and curcumin therapeutic approach. Tissue Cell 2022;76:101805. [Crossref] [PubMed]
- Du Y, Long Q, Zhang L, et al. Curcumin inhibits cancer-associated fibroblast-driven prostate cancer invasion through MAOA/mTOR/HIF-1α signaling. Int J Oncol 2015;47:2064-72. [Crossref] [PubMed]
- Termini D, Den Hartogh DJ, Jaglanian A, et al. Curcumin against Prostate Cancer: Current Evidence. Biomolecules 2020;10:1536. [Crossref] [PubMed]
- Wang X, Ma R, Zhang X, et al. Crosstalk between N6-methyladenosine modification and circular RNAs: current understanding and future directions. Mol Cancer 2021;20:121. [Crossref] [PubMed]
- Wang Y, Liu J, Ma J, et al. Exosomal circRNAs: biogenesis, effect and application in human diseases. Mol Cancer 2019;18:116. [Crossref] [PubMed]
- Liu X, Tong Y, Xia D, et al. Circular RNAs in prostate cancer: Biogenesis,biological functions, and clinical significance. Mol Ther Nucleic Acids 2021;26:1130-47. [Crossref] [PubMed]
- Chen S, Huang V, Xu X, et al. Widespread and Functional RNA Circularization in Localized Prostate Cancer. Cell 2019;176:831-843.e22. [Crossref] [PubMed]
- Chen W, Cen S, Zhou X, et al. Circular RNA CircNOLC1, Upregulated by NF-KappaB, Promotes the Progression of Prostate Cancer via miR-647/PAQR4 Axis. Front Cell Dev Biol 2020;8:624764. [Crossref] [PubMed]
- Tao LJ, Pan XY, Wang JW, et al. Circular RNA circANKS1B acts as a sponge for miR-152-3p and promotes prostate cancer progression by upregulating TGF-α expression. Prostate 2021;81:271-8. [Crossref] [PubMed]
- Wang X, Lu Z, Gomez A, et al. N6-methyladenosine-dependent regulation of messenger RNA stability. Nature 2014;505:117-20. [Crossref] [PubMed]
- Xiao W, Adhikari S, Dahal U, et al. Nuclear m(6)A Reader YTHDC1 Regulates mRNA Splicing. Mol Cell 2016;61:507-19. [Crossref] [PubMed]
- Wang X, Zhao BS, Roundtree IA, et al. N(6)-methyladenosine Modulates Messenger RNA Translation Efficiency. Cell 2015;161:1388-99. [Crossref] [PubMed]
- Yue Y, Liu J, Cui X, et al. VIRMA mediates preferential m(6)A mRNA methylation in 3’UTR and near stop codon and associates with alternative polyadenylation. Cell Discov 2018;4:10. [Crossref] [PubMed]
- Luo L, Li P, Xie Q, et al. n6-methyladenosine-modified circular RNA family with sequence similarity 126, member A affects cholesterol synthesis and malignant progression of prostate cancer cells by targeting microRNA-505-3p to mediate calnexin. J Cancer 2024;15:966-80. [Crossref] [PubMed]
- Zhong C, Long Z, Yang T, et al. M6A-modified circRBM33 promotes prostate cancer progression via PDHA1-mediated mitochondrial respiration regulation and presents a potential target for ARSI therapy. Int J Biol Sci 2023;19:1543-63. [Crossref] [PubMed]