Dexamethasone: a cheap and convenient way to alleviate subsequent stenosis after thermal injury on ureter
Highlight box
Key findings
• Dexamethasone (DEX) reduces the severity of renal fibrosis and inflammation after ureteral thermal injury (UTI) and exerts a significant renal protective effect.
What is known and what is new?
• Due to the development of electrosurgical instruments, thermal injury to the ureter has become one of the common causes of iatrogenic ureteral injury. Currently, animal modeling of UTI often involves larger animals, and exploration of therapeutic drugs is almost non-existent.
• This manuscript successfully created an animal model of UTI in mice for the first time using the Fast-pack pro-obturation pack handpiece instrument, and preliminarily confirmed the renal protective effect of DEX on UTI.
What is the implication, and what should change now?
• Future research should focus on exploring the therapeutic mechanism of DEX in treating UTI.
Introduction
Ureteral injuries are mostly caused by iatrogenic injuries, among which ureteral thermal injuries (UTIs) are the most common due to the widespread use of electrosurgical instruments. UTI results in the structural dysfunction of the ureter, reducing peristalsis at the injury site (1,2), initiating scar healing in the muscularis layer of the ureteral wall, and a reduction of the ureteral lumen, ultimately leading to ureteral strictures (3). The incidence of ureteral stricture varies among surgical procedures. For example, the postoperative stricture rate in urolithiasis treatment ranges from 0.3% to 4.9% (4), whereas the postoperative incidence of ureteral stricture in kidney transplant surgery is approximately 2% to 10% (5). Ureteral strictures often cause obstruction of the urine outflow channel, increased pressure in the renal pelvis and calyceal, secondary renal pelvis and calyceae dilatation and severe ipsilateral hydronephrosis, renal anatomical structure disorders, impaired normal urinary and excretory functions, and ultimately result in loss of renal function in some patients. Treatment of ureteral strictures remains a challenge for urologists. The timely recognition and management of UTIs are associated with desired outcomes. Currently, treatment for ureteral stricture caused by UTI includes the timely placement of a double J stent, balloon dilatation, pyeloplasty, ureteroureterostomy, transureterostomy, or psoas hitch/Boari flap repair when there is damage to the upper, middle, or lower segment of the ureter (6-8). However, many patients still respond poorly to surgical treatment. Moreover, the choice of surgical timing, the recurrence of stenosis in patients with scar constitution after surgical treatment, and the inability of patients to tolerate surgery and relieve obstruction over time owing to their limited basic conditions expose certain limitations of the surgical effect. Hence, it is of great significance to study how to prevent the ureter from further progressing further to scar stricture in the early stages of thermal injury, protect renal function from sustained damage, reduce the economic burden of medical expenses for patients, and save more social and medical resources.
At present, the basic animal exploration of UTI is relatively rare. Due to the thin wall and small diameter of ureteral tube, it is difficult to construct a suitable UTI animal model. In previous studies, UTI models were often constructed in large animals such as pigs or rabbits (7,9). Fast-pack pro-obturation pack handpiece instrument (Eighteeth, Changzhou, China) can be instantly heated, rising from room temperature to the target temperature within 0.2 seconds. At the same time, it can ensure that only the working tip is heated and can continuously maintain the working tip at the target temperature. After the heating is done, its tip rapidly cools down to room temperature in just 2 seconds. Furthermore, this instrument can also perform real-time temperature measurement through its tip’s temperature sensor. Based on this, we used this instrument to simulate UTI process in mouse models. In addition, studies on the immediate drug treatment after UTI to prevent the long-term ureteral strictures are currently lacking. Dexamethasone (DEX), the most common class of corticosteroids, mainly inhibits the aggregation of inflammatory cells at injured sites and inhibits phagocytosis and lysosomal enzyme or pro-inflammatory cytokine release, thus reducing inflammation and playing an anti-inflammatory role (10). DEX has been widely used to treat various diseases, including joint disorders (11), autoimmune diseases, allergies, tendinopathy (12), eye diseases, tumors, and, more recently, coronavirus disease 2019 (COVID-19) patients (13). However, the efficacy and causal mechanisms of DEX in UTI have not yet been elucidated. There are insufficient data with which available to discern the benefits and adverse effects of DEX in patients with UTI.
We hypothesized that the process by which UTI stimulates an inflammatory response, resulting in the excessive accumulation of extracellular matrix, which in turn induces ureteral stricture, can be treated using DEX. This hypothesis was tested using a UTI model in mice. We present this article in accordance with the ARRIVE reporting checklist (available at https://tau.amegroups.com/article/view/10.21037/tau-24-343/rc).
Methods
Animal model
Thirty specific-pathogen-free male C57BL/6J mice, aged 8 weeks and weighting 20–22 g, were chosen for this experiment. They were obtained from Hunan SLAC Laboratory Animal Co., Ltd. (Hunan, China). The mice were all kept in an environment with a temperature of 25 ℃, relative humidity of 60%, and a daily light-dark cycle of 12 hours, while having free access to standard food and water. After 1 week of acclimatization to the feeding environment, a total of 30 mice were randomly divided into the following three groups (n=10 per group): (I) sham operation group (control group), (II) ureter thermal injury group (UTI group, Figure 1), and (III) ureter thermal injury + DEX group (UTI + DEX group). Specifically, the mice were fasted for at least 1 h before the experiments and then anesthetized with an intraperitoneal injection of 1% pentobarbital sodium. Subsequently, the mice were positioned in the lateral decubitus position to open the posterior abdominal cavity. In the sham operation group, the left ureter was carefully separated without causing any injury, and then the abdominal incision was subsequently closed. The ureter thermal injury group was subjected to the same surgery, albeit with the left ureter injured using a fast-pack pro obturation pack handpiece instrument. The fast-pack pro-obturation pack handpiece instrument was used to maintain a temperature at 90 ℃ at the tip, followed by heat-damaging the ureter for approximately 1s. The ureter thermal injury + DEX group was intraperitoneally injected with DEX (2.5 mg/kg) daily for two weeks. The surgical procedures were conducted in a sterile and comfortable environment. Due to unfortunate incidents such as anesthesia accidents, surgical interventions, or intraperitoneal injections resulting in the death of some mice during the experiment, seven mice from each group were retained for subsequent statistical analysis. The mice were euthanized using carbon dioxide after a two-week period, and the ureter and kidney on the ligated side were harvested for additional experiments. Experiments were performed under a project license (No. 2022100948) granted by the Ethics Committee of Xiangya Hospital, Central South University, in compliance with institutional guidelines for the care and use of animals. A protocol was prepared before the study without registration.
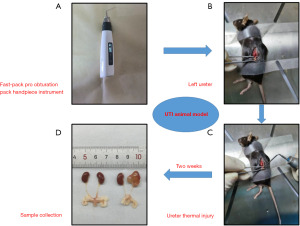
Assessment of serum blood-urea-nitrogen (BUN), creatinine (Cr), and uric acid (UA) levels
Blood samples were obtained from veins in the eyeball of the mice. Samples were stored at 4 ℃ overnight. Serum was collected by centrifugation at 3,000 rpm at 4 ℃ for 15 min. The levels of serum BUN, Cr, and UA levels were measured using detection kits (Servicebio, Wuhan, China), according to the manufacturer’s instructions. Rayto automatic biochemical analyzer were used to derive the results.
RNA extraction and quantitative real-time polymerase chain reaction (qRT-PCR)
Total RNA from mouse kidney tissues was prepared by using Trizol reagent (Takara, Japan), and the PrimeScript RT reagent Kit (Takara, Japan) was then used for reverse transcription PCR according to the manufacturer’s instructions. The real-time PCR system (Applied Biosystems, USA) was utilized for qRT-PCR with the SYBR Green PCR reagent (Takara). The relative messenger RNA (mRNA) expression levels of interleukin (IL)-1β, IL-6, IL-8, and tumor necrosis factor (TNF)-α were normalized using the GAPDH as the reference, and analyzed using the 2−ΔΔCt method. The sequences of the primer used for qRT-PCR are listed in Table S1.
Western blotting
To extract total protein, kidney tissues were rinsed three times with ice-cold PBS. Proteins were extracted from mouse kidney samples using RIPA lysis buffer (NCM Biotech, China) with the addition of 1% PMSF (NCM Biotech), And the protein concentration was determined using the BCA Protein Assay Kit (Beyotime Biotechnology, China). Briefly, 30 µg of protein was separated by 10% sodium dodecyl sulfate polyacrylamide gel electrophoresis (SDS-PAGE) (EpiZyme, China) and was transferred to polyvinylidene fluoride (PVDF) membranes (Merck Millipore, Germany). The membranes were blocked using protein free quick blocking solution (EpiZyme, Lot:034C1300) for 10 minutes at room temperature and incubated overnight with primary antibody overnight at 4 ℃. The details of the primary antibodies are included anti-glyceraldehyde 3-phosphate dehydrogenase (anti-GAPDH; 1:4,000; ab8245; Abcam; UK), anti-IL-1β (1:1,000; AF5103; Affinity, Jiangsu, China), anti-α-SMA (1:3,000; ab124964;) Abcam anti-collagen 1 collagen 1 (COL1) (1:1,000; 14695-1-AP; Proteintech; China), anti-fibronectin (FN) (1:1,000; 66042-1-Ig; Proteintech). After this, the membranes were then washed three times with TBST for 10 min each and exposed to the secondary antibody at room temperature for 1 h by using the goat anti-rabbit or goat anti-mouse IgG (1:5,000; Proteintech). Finally, an enhanced chemiluminescence (ECL) detection kit (NCM Biotech) was used to detect the protein bands. GAPDH was used as an internal reference. Furthermore, the band gray value was measured by Quantity One software (Bio-Rad, Berkeley, CA, USA). The relative densities of the protein bands were calculated using ImageJ software.
Hematoxylin-eosin (HE) staining, Masson staining and Sirius red staining
The mice kidney and ureter tissues were treated with 4% paraformaldehyde for 48 h, and then embedded in paraffin to prepare sections that were 4 μm thick. To estimate the severity of fibrosis, the sections were then stained with a HE staining kit (Solarbio, China), Masson’s trichrome staining kit (Solarbio) and Sirius Red staining kit according to the manufacturer’s instructions. Images were randomly obtained using a Leica light microscopy at a magnification of 20×.
Immunohistochemistry (IHC)
The mice kidney specimens were washed three times with PBS, and fixed with 4% paraformaldehyde for 48 h, and paraffin-embedded. Then, 4 μm thick sections were prepared. The sections were successively deparaffinized and hydrated, followed by antigen retrieval at 95 ℃ for 15 min and immersion in 10% hydrogen peroxide for 10 min. Next, the sections were blocked with 10% normal goat serum at room temperature for 30 min and incubated with primary antibodies using anti-IL-1β (1:500, AF5103; Affinity), anti-α-SMA (1:1,000; ab124964; Abcam), anti-collagen 1 (1:300; 14695-1-AP; Proteintech), anti-fibronectin (1:200; 66042-1-Ig; Proteintech) overnight at 4 ℃. The sections underwent three rounds of washing with PBS for 5 minutes each, followed by a 50-minute incubation at room temperature with the biotinylated secondary antibody (OriGene, USA). Lastly, the sections were reacted with DAB chromogen (OriGene) and counterstained with hematoxylin (Solarbio). Images were captured using a brightfield microscope (Leica, Germany).
Statistical analysis
Data were presented as the mean ± standard deviation (SD). One-way analysis of variance (ANOVA) was used to determine the differences among the three groups. A two tailed P value <0.05 was considered statistically significant. All statistical analyses were performed using the GraphPad Prism 8 software (GraphPad Software, La Jolla, CA, USA).
Results
Evaluation of experimental UTI model
Two weeks after UTI, we did not find a statistically significant difference in the body weight of three groups of mice (Figure S1A). And there were minimal changes in kidney size across all three groups; however, significant renal pelvis dilation was still evident in the UTI group, with DEX treatment effectively alleviating this symptom (Figure S1B). We then analyzed the histopathological changes in the ureter after thermal injury using HE staining. The results showed that the histological structure of the ureter was normal in the control group, while the ureter integrity was compromised and the ureteral lumen was significantly reduced in the UTI group; some epithelial cells were deficient and disarranged, the ureteral myometrium was interrupted and inflammatory cells were infiltrated (Figure 2). The treatment of UTI mice with DEX significantly prevented ureteral histopathological changes and inflammatory cell infiltration (Figure 2), which may be related to the anti-inflammatory protective effect of DEX.
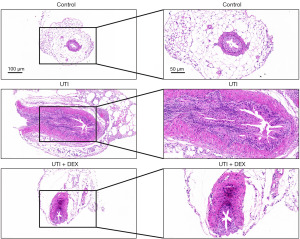
Renal function changes in experimental UTI following DEX intervention
To investigate whether renal function in mice deteriorated after UTI and the effect of DEX treatment on renal function, we examined the serum levels of BUN, Cr, and UA. Our data found that the serum BUN, Cr, and UA levels were significantly increased in the UTI and UTI + DEX groups compared with sham-operated controls (Figure 3). Compared to the UTI group, the serum BUN, Cr, and UA expression levels in the UTI + DEX group decreased for the treatment of DEX, but there was no statistical significance between the two groups (Figure 3). DEX prevented increases in BUN, Cr, and UA levels in the ureter following thermal injury to a certain extent, highlighting its potential renal protective effects against UTI.
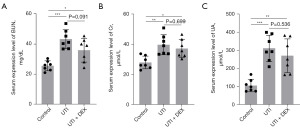
DEX ameliorates renal histopathological changes following UTI
According to the results of HE staining, the UTI group displayed profound glomerulosclerosis combined with tubular necrosis and dilatation compared with the sham-operated controls. UTI mice treated with DEX showed significantly fewer histopathological changes, as evidenced by the lower degree of renal structural damage and inflammatory cell infiltration, combined with a greater degree of tubular regeneration and necrotic tubules in regeneration (Figure 4A). Masson’s trichrome staining revealed that the renal tissue of the UTI group was darker, indicating the deposition of collagen. DEX treatment reduced the collagen fiber accumulation after thermal injury (Figure 4B). Furthermore, Sirius red staining was used to evaluate collagen deposition, showing results similar to the Masson staining analysis. The degree of fibrosis was found to be significantly increased in the kidney tissues of UTI mice, whereas DEX remarkably attenuated the renal fibrosis in the DEX + UTI group (Figure 4C).
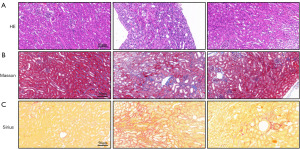
DEX partially ameliorates changes in renal inflammation markers following UTI
We examined the effect of DEX on the expression of renal inflammatory markers in mice with UTI. The qRT-PCR revealed that the mRNA expression levels of renal inflammation markers, such as IL-1β, IL-6, IL-8, and TNF-α, were significantly increased on day 14 post-UTI compared to those of sham-operated controls kidneys, whereas these inflammatory cytokines expression alternations were significantly inhibited by DEX (Figure 5A-5D). In addition, IL-1β was further evaluated by WB and IHC method. The results showed that UTI markedly increased the protein level of IL-1β, while the administration of DEX partially ameliorated the expression of IL-1β of UTI kidney (Figure 5E,5F). No significant difference was observed in the IL-6, IL-8, and TNF-α protein level among the groups. This suggests that DEX treatment alone regulated the expression of IL-6, IL-8, and TNF-α at the mRNA level.
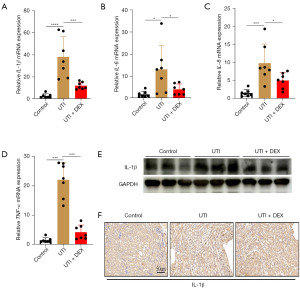
DEX treatment attenuates the expression of renal fibrosis-related markers after UTI
To determine whether DEX is involved in renal fibrosis induced by UTI, we investigated the expression of α-SMA, COL1, and FN, which are considered to be fibrosis related markers. WB analysis demonstrated that UTI induced significantly higher α-SMA, COL1, and FN protein levels in kidney tissues compared to the control, which were markedly inhibited by DEX (Figure 6A). Meanwhile, Immunostaining of fibrosis-related markers also showed positive staining in the interstitial areas due to UTI compared to the control. By contrast, the DEX + UTI group had significantly lower α-SMA, COL1, and FN protein levels compared to the UTI group (Figure 6B). These results suggest that DEX treatment alleviates renal fibrosis in the UTI mice.
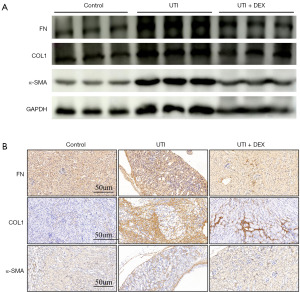
Discussion
In recent years, ureteral injury has been recognized as a serious and complex syndrome with numerous underlying etiologies. At present, owing to the current prevalence of laparoscopic surgery, ultrasonic or electric knives are widely used. Ureteral iatrogenic thermal injury is one of the most common causes of ureteral strictures and renal fibrosis (7). At present, the conventional treatments of ureteral stricture usually involve dilatation of the lumen (14-16), reconstructive anastomosis (16), or the placement of grafts, such as ileal ureter, appendix ureter, lingual mucosal graft and buccal mucosa (17-20). Many patients continue to have poor treatment results and may need additional surgery to relieve recurrent strictures, urine leakage and inflammatory response (21,22). Moreover, the exact molecular mechanisms underlying UTI and the most appropriate drug treatments are yet to be well defined. Hence, in addition to a rapid diagnosis, timely drug interventions to prevent renal failure are really necessary for the patients.
The ureteral injury caused by thermal damage is considered different from other injuries such as compression, ligation, transection. The localized elevation of temperature may cause damage to the ureter, leading to the denaturation of proteins or enzymes and tissue necrosis, resulting in more extensive damage than at the initial site (23). Currently, there is very little exploration of drugs for treating thermal injuries to the ureter. We think that one of the major reasons is the difficulty in creating animal models of UTI, which often require larger animals such as pigs, monkeys, and dogs. There are no suitable methods for constructing animal models of UTI in small animals like mice. Soria et al. used 24 female pigs to establish a unilateral iatrogenic perforation in a proximal ureter model and they demonstrated that heparin coated stents were ineffective in avoiding stent-associated bacteriuria (24). Another study used dogs to simulate an external or intraoperative radiation-induced ureteral damage model during radiotherapy (25). Ho et al. used New Zealand rabbits to reproduce thermal injury resulting in rabbit ureteral stricture by using electrocoagulation (7). However, owing to its animal size, high cost and feeding problems, it is not considered the best choice for animal models of UTI. In our study, we proposed a new method for constructing a UTI model with a high success rate, ease of feeding, and low cost (26,27). For the first time, a fast-pack pro-obturation pack handpiece was used to simulate thermal damage to the ureter using electrical surgical instruments. After two weeks of ureter wound healing, we found that the pathological changes in the thermal injury ureter tissues were more severe than those in the control group, which included urothelium loss, smooth muscle loss, and increased periurethral soft tissues thickness. Moreover, although most indicators of renal function (Cr, BUN, and UA) were within normal ranges two weeks after UTI, the serum of Cr, BUN and UA levels increased significantly. Furthermore, in our study, our results suggested that the inflammatory cytokines and fibrosis-related markers were significantly increased in the UTI kidney tissues. These results indicate that our instrument successfully stimulated ureteral injury in mice, resulting in renal inflammatory cytokine infiltration and renal fibrosis.
Ongoing research has focused on finding new and effective therapies for the prevention and treatment of UTI and their progression to ureteral strictures, including improving the operating skills of the surgeon, fluorescent ureteral catheter, and new pharmacological interventions (28-30). DEX is one of the most widely used glucocorticoids and has powerful anti-inflammatory and immunosuppressive effects (31). A randomized controlled study found that a single dose of DEX was effective and safe for reducing postoperative nausea and vomiting in patients with papillary thyroid carcinoma (32). DEX impedes Notch signaling in acute myeloid leukemia mesenchymal stromal cells and improves the survival rate of leukemic mice (33). Similar to Xue et al., we observed a significant reduction in the inflammatory response to short-term DEX treatment in animals with myocardial injury compared to control animals (33,34). Our qRT-PCR results of the inflammatory factor levels in the kidney tissues revealed that the expression levels of IL-1β, IL-6, IL-8 and TNF-α were significantly decreased in the UTI+ DEX group compared to those in the UTI group. However, no significant difference was observed in the expression of IL-6, IL-8 and TNF-α except IL-1β at the protein level between the UTI+ DEX and the UTI groups. Furthermore, the specific potential regulatory mechanism remains unclear. Thus, our data suggest that DEX significantly reduces the infiltration of inflammatory responses to a certain extent.
In addition to its anti-inflammatory effects, studies have also shown that DEX has an anti-fibrotic and renoprotective effects. One result demonstrated that HK-2 cells co-cultured with DEX can alleviate the epithelial-mesenchymal transition process and that the NF-κB pathway may play an important role in this process (35). Moreover, macrophage-derived microvesicles efficiently delivered DEX to the kidneys of inflamed mice and showed a superior capacity to inhibit renal fibrosis without obvious adverse reactions to glucocorticoids (36). Notably, our study also supports the beneficial effects of DEX in attenuating renal function deterioration. Meanwhile, the expression of the profibrotic markers was significantly lower after DEX treatment in the kidney tissues of the UTI+DEX group than in the UTI group.
Our study has a limitation in term of the potential mechanism of action of DEX in the UTI model. More studies should be carried out to confirm the exact injury mechanism of UTI and to explore the potential signaling pathways of DEX for improving the outcomes of UTI. Another limitation of our study is the lack of experimental results for heat-stimulated ureteral associated cell lines. This is because when the ureter is exposed to heat damage, it can lead to partial or complete damage of the ureteral cell layer. Therefore, further studies are warranted to determine the effect of DEX on thermal ureteral epithelial cells or ureteral smooth muscle cells, which may provide promising insights into potential therapeutic advances.
In conclusion, the results of our study clearly demonstrate that DEX significantly ameliorates heating-induced ureteral injury, resulting in improved renal inflammation and fibrosis. Taken together, our findings suggest that the timely application of DEX represents a viable option for the treatment of heating-induced ureteral injury.
Conclusions
Our study successfully created an animal model of UTI in mice for the first time using the Fast-pack pro-obturation pack handpiece instrument, and preliminarily confirmed the renal protective effect of DEX on UTI. These findings suggest that DEX may be a promising treatment option for preventing or reducing renal complications associated with UTIs.
Acknowledgments
Funding: The study was supported by
Footnote
Reporting Checklist: The authors have completed the ARRIVE reporting checklist. Available at https://tau.amegroups.com/article/view/10.21037/tau-24-343/rc
Data Sharing Statement: Available at https://tau.amegroups.com/article/view/10.21037/tau-24-343/dss
Peer Review File: Available at https://tau.amegroups.com/article/view/10.21037/tau-24-343/prf
Conflicts of Interest: All authors have completed the ICMJE uniform disclosure form (available at https://tau.amegroups.com/article/view/10.21037/tau-24-343/coif). W.X. reports funding from the Natural Science Foundation of Hunan province Youth Fund (No. 2023JJ40999) and National Youth Natural Science Foundation (No. 82400813). B.L. reports funding from the Natural Science Foundation of Hunan province Youth Fund (No. 2022JJ40796), the Postdoctoral International Exchange Program of China Postdoc Council (No. YJ20210203), National Youth Natural Science Foundation (No. 82200863), and Natural Science Foundation of Changsha (No. kq2202370). The other authors have no conflicts of interest to declare.
Ethical Statement: The authors are accountable for all aspects of the work in ensuring that questions related to the accuracy or integrity of any part of the work are appropriately investigated and resolved. Experiments were performed under a project license (No. 2022100948) granted by the Ethics Committee of Xiangya Hospital, Central South University, in compliance with institutional guidelines for the care and use of animals.
Open Access Statement: This is an Open Access article distributed in accordance with the Creative Commons Attribution-NonCommercial-NoDerivs 4.0 International License (CC BY-NC-ND 4.0), which permits the non-commercial replication and distribution of the article with the strict proviso that no changes or edits are made and the original work is properly cited (including links to both the formal publication through the relevant DOI and the license). See: https://creativecommons.org/licenses/by-nc-nd/4.0/.
References
- Ueshima E, Fujimori M, Kodama H, et al. Macrophage-secreted TGF-β(1) contributes to fibroblast activation and ureteral stricture after ablation injury. Am J Physiol Renal Physiol 2019;317:F52-64. [Crossref] [PubMed]
- Lange D, Bidnur S, Hoag N, et al. Ureteral stent-associated complications--where we are and where we are going. Nat Rev Urol 2015;12:17-25. [Crossref] [PubMed]
- Jiang Z, Wang J, Meng W, et al. Inhibition of Ureteral Stricture by Pirfenidone-Loaded Nanoparticle-Coated Ureteral Stents with Slow-Release Pirfenidone. Int J Nanomedicine 2022;17:6579-91. [Crossref] [PubMed]
- Moretto S, Saita A, Scoffone CM, et al. Ureteral stricture rate after endoscopic treatments for urolithiasis and related risk factors: systematic review and meta-analysis. World J Urol 2024;42:234. [Crossref] [PubMed]
- Caamiña L, Pietropaolo A, Prudhomme T, et al. Endourological Management of Ureteral Stricture in Patients with Renal Transplant: A Systematic Review of Literature. J Endourol 2024;38:290-300. [Crossref] [PubMed]
- Fu WJ, Wang ZX, Li G, et al. Comparison of a biodegradable ureteral stent versus the traditional double-J stent for the treatment of ureteral injury: an experimental study. Biomed Mater 2012;7:065002. [Crossref] [PubMed]
- Ho DR, Su SH, Chang PJ, et al. Biodegradable Stent with mTOR Inhibitor-Eluting Reduces Progression of Ureteral Stricture. Int J Mol Sci 2021;22:5664. [Crossref] [PubMed]
- Chatroux LR, Einarsson JI. Keep your attention closer to the ureters: Ureterolysis in deep endometriosis surgery. Best Pract Res Clin Obstet Gynaecol 2024;95:102494. [Crossref] [PubMed]
- Sorokin I, Canvasser N, Johnson B, et al. Irreversible Electroporation for Renal Ablation Does Not Cause Significant Injury to Adjacent Ureter or Bowel in a Porcine Model. J Endourol 2021;35:873-7. [Crossref] [PubMed]
- Madamsetty VS, Mohammadinejad R, Uzieliene I, et al. Dexamethasone: Insights into Pharmacological Aspects, Therapeutic Mechanisms, and Delivery Systems. ACS Biomater Sci Eng 2022;8:1763-90. [Crossref] [PubMed]
- Simón-Vázquez R, Tsapis N, Lorscheider M, et al. Improving dexamethasone drug loading and efficacy in treating arthritis through a lipophilic prodrug entrapped into PLGA-PEG nanoparticles. Drug Deliv Transl Res 2022;12:1270-84. [Crossref] [PubMed]
- Dietrich-Zagonel F, Aspenberg P, Eliasson P. Dexamethasone Enhances Achilles Tendon Healing in an Animal Injury Model, and the Effects Are Dependent on Dose, Administration Time, and Mechanical Loading Stimulation. Am J Sports Med 2022;50:1306-16. [Crossref] [PubMed]
- Potere N, Candeloro M, Porreca E, et al. Direct oral anticoagulant plasma levels in hospitalized COVID-19 patients treated with dexamethasone. J Thromb Thrombolysis 2022;53:346-51. [Crossref] [PubMed]
- Kuntz NJ, Neisius A, Tsivian M, et al. Balloon Dilation of the Ureter: A Contemporary Review of Outcomes and Complications. J Urol 2015;194:413-7. [Crossref] [PubMed]
- Yanagisawa T, Mori K, Quhal F, et al. Iatrogenic ureteric injury during abdominal or pelvic surgery: a meta-analysis. BJU Int 2023;131:540-52. [Crossref] [PubMed]
- Jensen AS, Rudnicki M. Iatrogenic bladder and ureteral injuries following gynecological and obstetric surgery. Arch Gynecol Obstet 2023;307:511-8. [Crossref] [PubMed]
- Ordorica R, Wiegand LR, Webster JC, et al. Ureteral replacement and onlay repair with reconfigured intestinal segments. J Urol 2014;191:1301-6. [Crossref] [PubMed]
- Patil N, Javali T. Application of the "Yang-Monti principle" in children with iatrogenic ureteral injuries. J Pediatr Urol 2021;17:543.e1-7. [Crossref] [PubMed]
- Engelmann SU, Yang Y, Pickl C, et al. Ureteroplasty with buccal mucosa graft without omental wrap: an effective method to treat ureteral strictures. World J Urol 2024;42:116. [Crossref] [PubMed]
- Wang X, Meng C, Li D, et al. Minimally invasive ureteroplasty with lingual mucosal graft for complex ureteral stricture: analysis of surgical and patient-reported outcomes. Int Braz J Urol 2024;50:46-57. [Crossref] [PubMed]
- Yang KK, Asghar AM, Lee RA, et al. Robot-Assisted Laparoscopic Distal Ureteroureterostomy for Distal Benign Ureteral Strictures with Long-Term Follow-Up. J Endourol 2022;36:203-8. [Crossref] [PubMed]
- Ambani SN, Skupin P, Malaeb BS, et al. Does Early Ureteroneocystostomy After Iatrogenic Ureteral Injury Jeopardize Outcome? Urology 2020;136:245-50. [Crossref] [PubMed]
- Limbachiya D, Tiwari R, Kumari R. Iatrogenic Thermal Energy-Induced Distal Ureteric Injury and Its Management by Laparoscopy Ureteroureterostomy. JSLS 2023;27:e2023.00030.
- Soria F, de La Cruz JE, Budia A, et al. Iatrogenic Ureteral Injury Treatment with Biodegradable Antireflux Heparin-Coated Ureteral Stent-Animal Model Comparative Study. J Endourol 2021;35:1244-9. [Crossref] [PubMed]
- Gillette SL, Gillette EL, Powers BE, et al. Ureteral injury following experimental intraoperative radiation. Int J Radiat Oncol Biol Phys 1989;17:791-8. [Crossref] [PubMed]
- Cho JH, Ryu HM, Oh EJ, et al. Alpha1-Antitrypsin Attenuates Renal Fibrosis by Inhibiting TGF-β1-Induced Epithelial Mesenchymal Transition. PLoS One 2016;11:e0162186. [Crossref] [PubMed]
- Su H, Liu H, Yang K, et al. Construction of a novel rabbit model of ureteral calculi implanted with flowable resin. BMC Urol 2022;22:105. [Crossref] [PubMed]
- Ryu S, Okamoto A, Nakashima K, et al. Ureteral navigation using a fluorescent ureteral catheter during laparoscopic colorectal surgery. Surg Endosc 2021;35:4882-9. [Crossref] [PubMed]
- Al-Taher M, van den Bos J, Schols RM, et al. Fluorescence Ureteral Visualization in Human Laparoscopic Colorectal Surgery Using Methylene Blue. J Laparoendosc Adv Surg Tech A 2016;26:870-5. [Crossref] [PubMed]
- Kim JK, Choi CI, Lee SH, et al. Silodosin for Prevention of Ureteral Injuries Resulting from Insertion of a Ureteral Access Sheath: A Randomized Controlled Trial. Eur Urol Focus 2022;8:572-9. [Crossref] [PubMed]
- Giles AJ, Hutchinson MND, Sonnemann HM, et al. Dexamethasone-induced immunosuppression: mechanisms and implications for immunotherapy. J Immunother Cancer 2018;6:51. [Crossref] [PubMed]
- Chen W, Li G, Jiang K, et al. Dexamethasone for Postoperative Nausea and Vomiting in Papillary Thyroid Carcinoma Patients: A Randomized Clinical Trial. J Am Coll Surg 2022;235:454-67. [Crossref] [PubMed]
- Ahmed HMM, Nimmagadda SC, Al-Matary YS, et al. Dexamethasone-mediated inhibition of Notch signalling blocks the interaction of leukaemia and mesenchymal stromal cells. Br J Haematol 2022;196:995-1006. [Crossref] [PubMed]
- Xue Q, Patterson AJ, Xiao D, et al. Glucocorticoid modulates angiotensin II receptor expression patterns and protects the heart from ischemia and reperfusion injury. PLoS One 2014;9:e106827. [Crossref] [PubMed]
- Li Q, Lv LL, Wu M, et al. Dexamethasone prevents monocyte-induced tubular epithelial-mesenchymal transition in HK-2 cells. J Cell Biochem 2013;114:632-8. [Crossref] [PubMed]
- Tang TT, Lv LL, Wang B, et al. Employing Macrophage-Derived Microvesicle for Kidney-Targeted Delivery of Dexamethasone: An Efficient Therapeutic Strategy against Renal Inflammation and Fibrosis. Theranostics 2019;9:4740-55. [Crossref] [PubMed]