Multiparametric magnetic resonance imaging of the prostate—a basic tutorial
IntroductionOther Section
- Introduction
- Hardware
- Sequences
- Patient preparation
- Clinical applications
- Conclusions
- Acknowledgements
- Footnote
- References
Multiparametric magnetic resonance imaging (mpMRI) of the prostate is revolutionizing the diagnosis of prostate cancer by providing high quality images with excellent tissue contrast. Historically, prostate MRI was available in only a few academic sites due to its high dependence on technical expertise and radiologist experience, but in recent years significant advances in hardware and software have greatly simplified image acquisition, leading to a steady improvement in the quality and diagnostic performance of MRI scans. Furthermore, the Prostate Imaging-Reporting and Data System (PI-RADS) version 2 has been recently introduced, establishing the minimum technical parameters that should be utilized, standardizing terminology, and providing guidelines for interpretation and reporting of imaging findings. These advances have allowed the clinical applications to broaden from loco-regional staging of prostate cancer to tumor detection, localization, risk stratification, and image-guided biopsy and treatment. The purpose of this article is to introduce the basic concepts of mpMRI of the prostate and its clinical applications, which will be covered in detail in subsequent chapters of this issue.
HardwareOther Section
- Introduction
- Hardware
- Sequences
- Patient preparation
- Clinical applications
- Conclusions
- Acknowledgements
- Footnote
- References
MRI is dependent on strong magnetic fields and the electromagnetic properties of hydrogen to generate the signal that is used to create images. The signal is very weak, though, and the main advantage of a 3 Tesla (3T) versus a 1.5 Tesla (1.5T) scanner is the increased signal-to-noise ratio. The increased signal strength at 3T allows for faster acquisition of images with higher spatial and temporal resolution. 3T scanners are also able to acquire other high demand sequences that may provide additional diagnostic information such as refined pharmacokinetic data (1). Though these sequences may be obtained on 1.5T scanners, the decreased signal to noise ratio, and lower spatial and temporal resolution provide suboptimal image quality. A growing number of studies are showing improved image quality resulting in better detection and characterization of prostate cancer with higher field strength (2). While there are many advantages when compared to 1.5T scanners, higher strength magnets are also more sensitive to inhomogeneous magnetic fields; and this can exacerbate artifacts cause by metallic hardware, such as hip prostheses, or air and stool in the rectum.
All prostate MRI exams use an external phased array coil placed over the pelvis to excite the protons and receive their signal to generate the images; however some institutions elect to use an additional endorectal coil. These coils are positioned directly opposed to the prostate, further increasing the signal and resolution. The use of endorectal coils is debatable for both 1.5T and 3T scanners, but generally considered a requirement for 1.5T systems.
Recent studies have shown that the use of an endorectal coil does not increase tumor detection, but the ability to obtain higher resolution images may improve local staging. While the endorectal coil improves image quality, it comes with some disadvantages including patient discomfort, gland deformation and enema preparation. Ongoing hardware and software improvements may lead to adequate and accurate imaging without it.
SequencesOther Section
- Introduction
- Hardware
- Sequences
- Patient preparation
- Clinical applications
- Conclusions
- Acknowledgements
- Footnote
- References
Applying magnetic gradients and radiofrequency pulses generates MR images. By changing scanning parameters one obtains different pulse sequences, or sequences for short. Each sequence provides different, but complementary information which is crucial for diagnosis. A mpMRI exam of the prostate consists of several sequences including T1-weighted, T2-weighted, dynamic contrast-enhanced, and diffusion-weighted MRI (Figure 1). Occasionally, MR spectroscopic imaging is also obtained. Each of these sequences can be thought of distinct components that together comprise a complete MR examination. A basic knowledge of tissue signal characteristics with each sequence is key for understanding prostate MRI. A finding seen on any single sequence should always be correlated with the other sequences for accurate characterization.
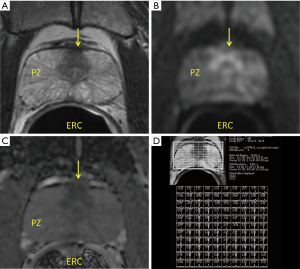
T2-weighted MR imaging
T2-weighted images (T2WI) are the mainstay of prostate MRI. High resolution T2WI are acquired in three different imaging planes (axial, coronal, and sagittal) with a small field-of-view focused on the prostate. These images provide the best opportunity to evaluate the prostatic zonal anatomy and to determine the presence of extra-prostatic extension. Findings of extraprostatic extension (EPE) include asymmetry of neurovascular bundles, obliteration of rectoprostatic angle, bulging of the prostatic contour, and capsular irregularity with tumor in the rectoprostatic fat (Figure 2).
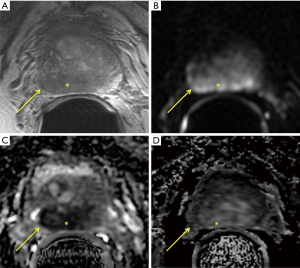
A recent meta-analysis by de Rooij et al. showed 53% sensitivity, 91% specificity for detection of EPE (3). The modest sensitivity has caused some to argue against the use of MRI, but the accuracy and sensitivity have been shown to improve when patients are scanned on 3T systems and images reviewed by experienced readers (3,4).
In the peripheral zone, typical prostate cancers appear as round or ill-defined dark (low T2 signal) lesions on a bright (high T2 signal) background of glandular tissue. Unfortunately low T2 signal in the peripheral zone is nonspecific; prostatitis, post-biopsy hemorrhage, glandular atrophy, and post-treatment changes can have a similar appearance. Accordingly, T2WI alone is not adequate for the diagnosis and localization of prostate cancer; in one study, Rosenkrantz et al. demonstrated a diagnostic accuracy of 60% (5). This underscores the importance of correlation with clinical history as well as imaging characteristics on the remaining sequences (6).
The transition zone contains variable amounts of glandular (high T2 signal), and stromal (low T2 signal) tissue. Since prostate cancer usually demonstrate low T2 signal, benign stromal tissue can mimic or obscure malignant lesions. Transition zone tumors are difficult to detect, yet T2WI is the preferred imaging sequence for evaluation of such lesions because there is significant overlap of imaging findings of benign prostatic hyperplasia and prostate cancer on the other sequences (7). Hoeks et al. recently compared the accuracy of T2WI alone with mpMRI and did not find a difference between the two (68% vs. 66%) (8). The diagnostic accuracy of T2WI for the detection of transition zone tumors ranges from 62% to 81% (9-11).
T1-weighted MR imaging
T1 weighted images (T1WI) are not utilized for the direct assessment of prostate cancer, as the zonal anatomy and tumors are very poorly visualized. However, these images are usually acquired using a large field of view of the pelvis, allowing for the assessment for regional lymph nodes and osseous structures. While an abdomen and pelvis CT scan and bone scintigraphy are often obtained in high-risk cases, this sequence provides an opportunity to detect unsuspected metastases.
T1WI are also valuable for detection of post-biopsy hemorrhage. Hemorrhage may mimic tumor on T2WI because it has low T2 signal, but it is easy recognized on T1WI (Figure 3). Furthermore, hemorrhage may also limit the accuracy of other sequences. There is no consensus as to the time interval between prostate biopsy and mpMRI, but the American College of Radiology and European Society of Urogenital Radiology suggest at least 6 weeks.
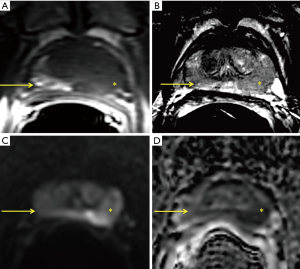
Diffusion-weighted MR imaging (DWI)
DWI generates image contrast based on differences in the rate of diffusion of water molecules in soft tissues relative to free solution. With an increase in grade of prostate cancer, there is an increase in cellularity, progressive loss of ductal architecture, and decrease the cytoplasm-to-nucleus ratio, all of which reduce the ability of water to diffuse. In other words, prostate cancer demonstrates restricted diffusion (of water molecules) and the higher the grade of cancer, the more significant is the restriction (Figure 2). DWI is comprised of two sets of images for analysis, the high b-value images and an apparent diffusion coefficient (ADC) map. A b-value is a parameter of DWI that measures the strength of the diffusion weighting. Several studies have shown improved tumor detection using high b-values in the range of 1,400–2,000 (12-15). While the interpretation of DWI is subjective, the restriction of water molecules can be quantified. This is done generating ADC maps and measuring ADC values (mm2/s). Structures that demonstrate reduced diffusion will appear bright on DWI and dark on the ADC map (low ADC values) (Figure 2). Several studies have shown an inverse correlation between ADC values and Gleason score (16-18). In addition, DWI and ADC maps are very useful for detection of prostate cancer, particularly in the peripheral zone, with sensitivities ranging from 65% to 84%, and specificities of 77% to 87% (9,19).
Dynamic contrast-enhanced (DCE) MR imaging
DCE MRI is performed by obtaining T1-weighted images with suppression of the signal of fat before and after the administration of intravenous gadolium based contrast agents. Post contrast images of the entire gland are acquired in rapid succession over several minutes, allowing for the study of the kinetics of contrast enhancement in the prostatic tissue. An entire set of images is typically acquired every 5–10 seconds for at least 2 minutes (Figure 2).
Malignant cells release factors that promote neovascularization and increased capillary permeability. These factors are believed to cause prostate cancer to demonstrate rapid enhancement and faster washout of contrast relative to normal tissue. While many tumors demonstrate these classic features, kinetics are variable and DCE alone cannot definitively diagnose or exclude malignancy. This determination must be made in conjunction with the morphologic and functional features seen on the accompanying sequences. If abnormal enhancement is seen, other sequences must be closely examined to confirm the presence or absence of a suspicious lesion.
While PI-RADS version 2 still recommends the acquisition of DCE, its use remains a matter of debate. While multiple studies have shown limited additional benefit beyond T2-weighted and diffusion-weighted (5,8,20), others have suggested that DCE is helpful for the detection of small lesions and to predict treatment response. There are three different methods for interpretation of DCE images: subjectively using visual inspection, semi-objectively using calculation of various kinetic parameters, enhancement curves and colorized parametric maps superimposed on anatomic images, or objectively using pharmacokinetic models that measure concentration of gadolinium within the tissues (21).
MR spectroscopic imaging (MRSI)
Proton MRSI depicts the metabolic profile of tissues. In the case of prostate cancer, three metabolites are of main interest: citrate, choline, and creatine. Citrate is found in abundance in normal prostatic tissue, but it is decreased when the gland is replaced by cancer. Choline is a phospholipid membrane component and it is increased in cancer because of high cell membrane turnover associated with neoplastic proliferation. Creatine is also a normal peak, but it remains unchanged in the presence of cancer and serves as internal reference. Relative concentrations of these metabolites are quantified using choline + creatinine-to-citrate and choline-to-citrate ratios. Use of MRSI remains limited to a few institutions, as image acquisition is difficult, extensive post-processing is required, and interpretation is time consuming and challenging. Although studies from experienced institution have found promising results (22,23), other recent research failed to show additional benefit, perhaps because of the challenges mentioned above (24,25).
Patient preparationOther Section
- Introduction
- Hardware
- Sequences
- Patient preparation
- Clinical applications
- Conclusions
- Acknowledgements
- Footnote
- References
The high magnetic field MRI scanners (1.5T and 3T) used in clinical practice generally have bore sizes of approximately 60 cm. The confined space and prolonged image acquisition can cause anxiety even when patients do not suffer from claustrophobia. It is therefore important to alert patients to this possibility and thoroughly explain the procedure. If necessary, high quality images may still be obtained with the aid of anxiolytic medications. Yet, these are usually not available in radiology departments, and must be prescribed by the referring physicians in advance. In addition to the standard screening procedures that all patients undergoing MRI must partake in (Figure 4), there are certain aspects of prostate MRI that require additional consideration. There are no formal guidelines for patient preparation and procedures vary from practice to practice. Some centers utilize antispasmodic agents such as butylscopolamine prior to image acquisition in order to decrease peristalsis and motion related artifacts, though use of these agents is controversial, as studies have failed to demonstrate improved image quality (26). Though not required, patients being imaged with an endorectal coil are advised to use a saline laxative enema within 3 hours of the examination in order to facilitate coil placement as well as reduce artifacts created by air and stool (27) (Figure 5).
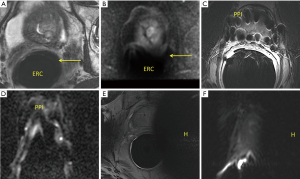
Patients are generally scanned in the supine position as this is better tolerated and minimizes respiratory motion. One of the main indications for scanning a patient in the prone position is the presence of a large quantity of rectal gas in cases in which an endorectal coil is not being used. This change in positioning can promote redistribution of bowel gas, decreasing the probability of artifact, thereby producing higher quality images.
Clinical applicationsOther Section
- Introduction
- Hardware
- Sequences
- Patient preparation
- Clinical applications
- Conclusions
- Acknowledgements
- Footnote
- References
The most widely accepted uses of mpMRI of the prostate include biopsy guidance, local staging, post-treatment assessment, and as an adjunct tool for active surveillance (AS).
Biopsy
For many years, the standard for prostate biopsy was the sextant technique of sampling 6 separates sites, which over time has expanded up to 30 sites. The limitations of such systematic, non-targeted techniques are now well recognized, but in short they are associated with over diagnosis of low-grade disease and under diagnosis of clinically significant disease, with discrepant Gleason scores between biopsy and surgical specimens (28). The superior tissue contrast and improved visualization of prostate cancer using mpMRI has led to the implementation of targeted biopsy. Targeted biopsy techniques include cognitive fusion biopsy, i.e., visual co-registration of MRI and TRUS, MRI/TRUS fusion biopsy, where MRI data is overlaid to real-time TRUS images, and in-bore MRI guided biopsy, when biopsies are performed with the patient inside the MRI scanner. Cognitive fusion has been shown to improve disease characterization when compared to systematic biopsy (29), and both MRI/TRUS fusion and in-bore techniques are better than the cognitive and systematic approaches (30,31). These newer MRI-guided procedures reduce sampling error and detect more high-risk and fewer low-risk cancers when compared to systematic biopsy (31,32). It is important to understand that though these techniques are an improvement over the prior standard of care, up to 17% of clinically significant cancers are still missed when compared to prostatectomy specimens (33,34). For this reason, the current recommendation is to perform systematic biopsy in addition to targeted biopsy (35). In addition to directing biopsy, improved tumor localization and characterization can also guide focal therapy such as ablation (cryotherapy, laser, photodynamic), electroporation, and high intensity focused ultrasound. Targeted biopsy methods will be discussed in further detail later in this issue.
Local staging
mpMRI of the prostate is currently the best option for local staging. The limitations of serum PSA and digital rectal examination for staging are well recognized. And while the accuracy of transrectal ultrasound (TRUS) for the detection of EPE can be similar to that of T2WI when performed by an experienced operator (36), it typically does not provide detailed enough evaluation for accurate staging.
A recent meta-analysis showed high specificity for the detection of EPE, seminal vesicle invasion (SVI), and overall staging of T3 disease when using T2WI with DWI or DCE to range from 88–96% (3). mpMRI may also be helpful for local lymph node staging and evaluation of pelvic osseous metastases, but given the limited field-of-view, other cross sectional imaging modalities, such as computed tomography (CT) and nuclear medicine techniques, are better suited for these tasks .
Post-treatment evaluation
A rising PSA following definitive therapy, i.e., biochemical failure, provides accurate evidence of recurrent prostate cancer. The challenge is determining whether this increase in PSA is a result of isolated local recurrence or metastatic disease.
mpMRI has high sensitivity and specificity for detection of local recurrence ranging from 85–97% and 90–100%, respectively (37). Different sequences may be of particular value in different situations. For example a recent study showed the increased sensitivity of DWI for detection of local recurrence following radiation therapy (23), while other studies have shown that DCE is the most sensitive for diagnosis of local recurrence following prostatectomy (38,39). As for patients who are treatment-naïve, imaging findings are utilized to guide biopsies in this group of men. While the detection of local recurrence does not exclude the presence of metastatic disease, a negative mpMRI will increase its probability. Other imaging modalities, such as CT, nuclear scintigraphy, and positron emission tomography are usually used to evaluate for distant metastases.
AS
One of the main challenges of AS is accurate selection of patients with prostate cancer that will never become clinically manifest and exclusion of men whose cancers should be treated with definitive therapy. Several studies have shown that up to about 40% of patients under AS receive definitive treatment within 3–5 years, typically because re-biopsy diagnoses disease upgrading (40-42). Disease upgrade, however, may be due to inaccurate characterization at the time of diagnosis or true progression of disease. Given the high quality anatomic and functional data provided by mpMRI, some have suggested mpMRI as a potential adjunct to AS, first by more accurately identifying candidates for surveillance, and also by monitoring men classified as low risk patients (33,43,44). For example, a study by Thompson et al. showed mpMRI to have high sensitivity and negative predictive value for clinically significant prostate cancer (45). In addition, many AS patients opt for definitive therapy to avoid repeat routine biopsies, which in some protocols are performed yearly. mpMRI may also have a role avoiding or postponing these per protocol biopsies.
ConclusionsOther Section
- Introduction
- Hardware
- Sequences
- Patient preparation
- Clinical applications
- Conclusions
- Acknowledgements
- Footnote
- References
Multiparametric MRI of the prostate is an exam consisting of several components including T2-weighted imaging, T1-weighted imaging, diffusion-weighted imaging, and dynamic contrast enhanced imaging. It provides high quality anatomic and functional images that improve the diagnosis of prostate cancer, assisting with risk-stratification and treatment selection. The concepts presented in this article are important for providing a foundation of knowledge that will be expanded upon by subsequent articles in this issue.
AcknowledgementsOther Section
- Introduction
- Hardware
- Sequences
- Patient preparation
- Clinical applications
- Conclusions
- Acknowledgements
- Footnote
- References
None.
FootnoteOther Section
- Introduction
- Hardware
- Sequences
- Patient preparation
- Clinical applications
- Conclusions
- Acknowledgements
- Footnote
- References
Conflicts of Interest: 3D Biopsy, Scientific Advisory Board; and Verily Life Sciences, research grant (to AC Westphalen). MC Cabarrus has no conflicts of interest to declare.
ReferencesOther Section
- Introduction
- Hardware
- Sequences
- Patient preparation
- Clinical applications
- Conclusions
- Acknowledgements
- Footnote
- References
- Fütterer JJ, Scheenen TW, Huisman HJ, et al. Initial experience of 3 tesla endorectal coil magnetic resonance imaging and 1H-spectroscopic imaging of the prostate. Invest Radiol 2004;39:671-80. [Crossref] [PubMed]
- Verma S, Turkbey B, Muradyan N, et al. Overview of dynamic contrast-enhanced MRI in prostate cancer diagnosis and management. AJR Am J Roentgenol 2012;198:1277-88. [Crossref] [PubMed]
- de Rooij M, Hamoen EH, Witjes JA, et al. Accuracy of Magnetic Resonance Imaging for Local Staging of Prostate Cancer: A Diagnostic Meta-analysis. Eur Urol 2016;70:233-45. [Crossref] [PubMed]
- Garcia-Reyes K, Passoni NM, Palmeri ML, et al. Detection of prostate cancer with multiparametric MRI (mpMRI): effect of dedicated reader education on accuracy and confidence of index and anterior cancer diagnosis. Abdom Imaging 2015;40:134-42. [Crossref] [PubMed]
- Rosenkrantz AB, Kim S, Campbell N, et al. Transition zone prostate cancer: revisiting the role of multiparametric MRI at 3 T. AJR Am J Roentgenol 2015;204:W266-72. [Crossref] [PubMed]
- Langer DL, van der Kwast TH, Evans AJ, et al. Prostate cancer detection with multi-parametric MRI: logistic regression analysis of quantitative T2, diffusion-weighted imaging, and dynamic contrast-enhanced MRI. J Magn Reson Imaging 2009;30:327-34. [Crossref] [PubMed]
- Weinreb JC, Barentsz JO, Choyke PL, et al. PI-RADS Prostate Imaging - Reporting and Data System: 2015, Version 2. Eur Urol 2016;69:16-40. [Crossref] [PubMed]
- Hoeks CM, Hambrock T, Yakar D, et al. Transition zone prostate cancer: detection and localization with 3-T multiparametric MR imaging. Radiology 2013;266:207-17. [Crossref] [PubMed]
- Delongchamps NB, Rouanne M, Flam T, et al. Multiparametric magnetic resonance imaging for the detection and localization of prostate cancer: combination of T2-weighted, dynamic contrast-enhanced and diffusion-weighted imaging. BJU Int 2011;107:1411-8. [Crossref] [PubMed]
- Haider MA, van der Kwast TH, Tanguay J, et al. Combined T2-weighted and diffusion-weighted MRI for localization of prostate cancer. AJR Am J Roentgenol 2007;189:323-8. [Crossref] [PubMed]
- Yoshizako T, Wada A, Hayashi T, et al. Usefulness of diffusion-weighted imaging and dynamic contrast-enhanced magnetic resonance imaging in the diagnosis of prostate transition-zone cancer. Acta Radiol 2008;49:1207-13. [Crossref] [PubMed]
- Katahira K, Takahara T, Kwee TC, et al. Ultra-high-b-value diffusion-weighted MR imaging for the detection of prostate cancer: evaluation in 201 cases with histopathological correlation. Eur Radiol 2011;21:188-96. [Crossref] [PubMed]
- Wang X, Qian Y, Liu B, et al. High-b-value diffusion-weighted MRI for the detection of prostate cancer at 3 T. Clin Radiol 2014;69:1165-70. [Crossref] [PubMed]
- Rosenkrantz AB, Chandarana H, Hindman N, et al. Computed diffusion-weighted imaging of the prostate at 3 T: impact on image quality and tumour detection. Eur Radiol 2013;23:3170-7. [Crossref] [PubMed]
- Ueno Y, Takahashi S, Ohno Y, et al. Computed diffusion-weighted MRI for prostate cancer detection: the influence of the combinations of b-values. Br J Radiol 2015;88:20140738. [Crossref] [PubMed]
- Hambrock T, Vos PC, Hulsbergen-van de Kaa CA, et al. Prostate cancer: computer-aided diagnosis with multiparametric 3-T MR imaging--effect on observer performance. Radiology 2013;266:521-30. [Crossref] [PubMed]
- Park SY, Oh YT, Jung DC, et al. Diffusion-weighted imaging predicts upgrading of Gleason score in biopsy-proven low grade prostate cancers. BJU Int 2017;119:57-66. [Crossref] [PubMed]
- Nagarajan R, Margolis D, Raman S, et al. Correlation of Gleason scores with diffusion-weighted imaging findings of prostate cancer. Adv Urol 2012;2012:374805.
- Wu LM, Xu JR, Ye YQ, et al. The clinical value of diffusion-weighted imaging in combination with T2-weighted imaging in diagnosing prostate carcinoma: a systematic review and meta-analysis. AJR Am J Roentgenol 2012;199:103-10. [Crossref] [PubMed]
- Rais-Bahrami S, Siddiqui MM, Vourganti S, et al. Diagnostic value of biparametric magnetic resonance imaging (MRI) as an adjunct to prostate-specific antigen (PSA)-based detection of prostate cancer in men without prior biopsies. BJU Int 2015;115:381-8. [Crossref] [PubMed]
- Gupta RT, Kauffman CR, Polascik TJ, et al. The state of prostate MRI in 2013. Oncology (Williston Park) 2013;27:262-70. [PubMed]
- Zakian KL, Hatfield W, Aras O, et al. Prostate MRSI predicts outcome in radical prostatectomy patients. Magn Reson Imaging 2016;34:674-81. [Crossref] [PubMed]
- Westphalen AC, Reed GD, Vinh PP, et al. Multiparametric 3T endorectal mri after external beam radiation therapy for prostate cancer. J Magn Reson Imaging 2012;36:430-7. [Crossref] [PubMed]
- Weinreb JC, Blume JD, Coakley FV, et al. Prostate cancer: sextant localization at MR imaging and MR spectroscopic imaging before prostatectomy--results of ACRIN prospective multi-institutional clinicopathologic study. Radiology 2009;251:122-33. [Crossref] [PubMed]
- Casciani E, Polettini E, Bertini L, et al. Contribution of the MR spectroscopic imaging in the diagnosis of prostate cancer in the peripheral zone. Abdom Imaging 2007;32:796-802. [Crossref] [PubMed]
- Venkatanarasimha N, Jenkins SJ, Yang N, et al. Impact of butylscopolamine on image quality of magnetic resonance cholangiopancreatography. Eur J Radiol 2013;82:583-8. [Crossref] [PubMed]
- Starobinets O, Korn N, Iqbal S, et al. Practical aspects of prostate MRI: hardware and software considerations, protocols, and patient preparation. Abdom Radiol (NY) 2016;41:817-30. [Crossref] [PubMed]
- Köksal IT, Ozcan F, Kadioglu TC, et al. Discrepancy between Gleason scores of biopsy and radical prostatectomy specimens. Eur Urol 2000;37:670-4. [Crossref] [PubMed]
- Puech P, Rouvière O, Renard-Penna R, et al. Prostate cancer diagnosis: multiparametric MR-targeted biopsy with cognitive and transrectal US-MR fusion guidance versus systematic biopsy--prospective multicenter study. Radiology 2013;268:461-9. [Crossref] [PubMed]
- Oberlin DT, Casalino DD, Miller FH, et al. Diagnostic Value of Guided Biopsies: Fusion and Cognitive-registration Magnetic Resonance Imaging Versus Conventional Ultrasound Biopsy of the Prostate. Urology 2016;92:75-9. [Crossref] [PubMed]
- Acar Ö, Esen T, Çolakoğlu B, et al. Multiparametric MRI guidance in first-time prostate biopsies: what is the real benefit? Diagn Interv Radiol 2015;21:271-6. [Crossref] [PubMed]
- Siddiqui MM, Rais-Bahrami S, Turkbey B, et al. Comparison of MR/ultrasound fusion-guided biopsy with ultrasound-guided biopsy for the diagnosis of prostate cancer. JAMA 2015;313:390-7. [Crossref] [PubMed]
- Tran GN, Leapman MS, Nguyen HG, et al. Magnetic Resonance Imaging-Ultrasound Fusion Biopsy During Prostate Cancer Active Surveillance. Eur Urol 2016. [Epub ahead of print]. [Crossref] [PubMed]
- Muthigi A, George AK, Sidana A, et al. Missing the Mark: Prostate Cancer Upgrading by Systematic Biopsy over Magnetic Resonance Imaging/Transrectal Ultrasound Fusion Biopsy. J Urol 2017;197:327-34. [Crossref] [PubMed]
- Radtke JP, Kuru TH, Boxler S, et al. Comparative analysis of transperineal template saturation prostate biopsy versus magnetic resonance imaging targeted biopsy with magnetic resonance imaging-ultrasound fusion guidance. J Urol 2015;193:87-94. [Crossref] [PubMed]
- Jung A, Coakley F, Shinohara K, et al. Local Staging of Prostate Cancer: Comparative Accuracy of Contemporary Endorectal MRI and Transrectal Ultrasound. AJR Am J Roentgenol 2011;196:A31-35.
- Radtke JP, Teber D, Hohenfellner M, et al. The current and future role of magnetic resonance imaging in prostate cancer detection and management. Transl Androl Urol 2015;4:326-41. [PubMed]
- Panebianco V, Barchetti F, Sciarra A, et al. Prostate cancer recurrence after radical prostatectomy: the role of 3-T diffusion imaging in multi-parametric magnetic resonance imaging. Eur Radiol 2013;23:1745-52. [Crossref] [PubMed]
- Sciarra A, Barentsz J, Bjartell A, et al. Advances in magnetic resonance imaging: how they are changing the management of prostate cancer. Eur Urol 2011;59:962-77. [Crossref] [PubMed]
- Dall'Era MA, Konety BR, Cowan JE, et al. Active surveillance for the management of prostate cancer in a contemporary cohort. Cancer 2008;112:2664-70. [Crossref] [PubMed]
- Cooperberg MR, Cowan JE, Hilton JF, et al. Outcomes of active surveillance for men with intermediate-risk prostate cancer. J Clin Oncol 2011;29:228-34. [Crossref] [PubMed]
- Eggener SE, Mueller A, Berglund RK, et al. A multi-institutional evaluation of active surveillance for low risk prostate cancer. J Urol 2009;181:1635-41; discussion 1641. [Crossref] [PubMed]
- Flavell RR, Westphalen AC, Liang C, et al. Abnormal findings on multiparametric prostate magnetic resonance imaging predict subsequent biopsy upgrade in patients with low risk prostate cancer managed with active surveillance. Abdom Imaging 2014;39:1027-35. [Crossref] [PubMed]
- Stamatakis L, Siddiqui MM, Nix JW, et al. Accuracy of multiparametric magnetic resonance imaging in confirming eligibility for active surveillance for men with prostate cancer. Cancer 2013;119:3359-66. [Crossref] [PubMed]
- Thompson JE, Moses D, Shnier R, et al. Multiparametric magnetic resonance imaging guided diagnostic biopsy detects significant prostate cancer and could reduce unnecessary biopsies and over detection: a prospective study. J Urol 2014;192:67-74. [Crossref] [PubMed]