Is early-life obesity associated with kidney cancer in adulthood?—insights from genetic studies
Highlight box
Key findings
• This study identified a genetic association between early obesity and the risk of kidney cancer in adulthood using Mendelian randomization analysis.
What is known and what is new?
• Adult obesity is strongly associated with a variety of cancers, including kidney cancer. Some observational studies suggest a potential association between early-life obesity and kidney cancer in adulthood.
• This study provides new evidence linking genetic-based early-life obesity with kidney cancer in adulthood.
What is the implication, and what should change now?
• In the context of the global obesity epidemic, maintaining a healthy weight from an early age is important to reduce the burden of kidney cancer.
Introduction
The latest cancer statistics from the World Health Organization reveals over 430,000 new cases of kidney cancer (KIC) and more than 150,000 deaths globally (1). KIC, histopathologically classified into two main categories—renal cell carcinoma (RCC) originating from renal tubular epithelial cells and renal pelvic carcinoma from urothelial cells—comprises 90% and 10% of cases respectively (2). RCC presents insidiously, challenging early diagnosis due to the absence of specific clinical symptoms. Frequently detected incidentally during physical examinations, approximately 17% of patients exhibit distant metastases upon initial diagnosis (3).
Epidemiological studies indicate that risk factors for KIC include occupational exposure, smoking, and hypertension, among others (4). Obesity represents another well-established risk factor. The International Agency for Research on Cancer asserts sufficient evidence linking increased body mass index (BMI) with higher RCC risk, particularly in the context of the current obesity epidemic (5). In high-income countries, overweight and obesity account for 8% of cancer cases, with expectations of further increasing burdens (6). However, RCC primarily peaks in the elderly, with a median age at diagnosis of approximately 75 years (7). Given the prolonged latency period observed in many cancers from initial carcinogenic exposure to malignancy detection, early-life risk factors may potentially impact the future progression of KIC.
Mendelian randomization (MR) is an innovative epidemiological approach grounded in genome-wide association study (GWAS) data, employing single nucleotide polymorphisms (SNPs) as instrumental variables (IVs) to uncover causal relationships. MR effectively addresses confounding factors and reverse causality, which cannot be fully eliminated in observational studies (8). In this study, we collected GWAS summary data of birth weight, childhood BMI, adolescent BMI, and adult BMI, performing MR analysis to investigate the effect of BMI throughout growth stages on adult KIC. We present this article in accordance with the STROBE-MR reporting checklist (available at https://tau.amegroups.com/article/view/10.21037/tau-24-521/rc).
Methods
Study design
The overall flow of this study is illustrated in Figure 1. The study was conducted in accordance with the Declaration of Helsinki (as revised in 2013). The data utilized in this study were sourced from publicly accessible databases, obviating the need for institutional ethics review board approval. IVs for MR analysis must meet specific criteria: (I) IVs must be strongly correlated with the exposure factor (correlation); (II) IVs should exhibit no correlation with confounding factors (independence); (III) IVs must not be directly associated with outcome variables, but rather solely through the exposure factors (exclusion restriction).
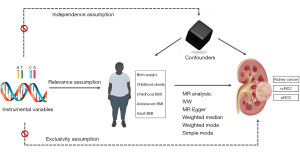
Exposure data
GWAS summary data for birth weight were extracted from the study conducted by Warrington et al., encompassing 298,142 individuals of European descent (9). GWAS summary data for childhood BMI were acquired from the study conducted by Vogelezang et al., involving 39,620 European samples aged between 2 and 10 years (10). GWAS data for adolescent BMI were derived from published studies encompassing 29,880 European individuals aged 16 to 25 years (11). As for adult BMI GWAS summary data, we utilized the Locke et al. study, which included 339,224 European samples aged between 40 and 75 years (12).
Outcome data
GWAS summary data on KIC were extracted from a comprehensive meta-analysis recently published by Purdue et al. (13). This study included data from seven published studies, a newly integrated study, and three large biobanks (UK Biobank, FinnGen, and Biobank Japan), comprising a total of 29,020 cases of KIC and 835,670 controls. Specifically, for clear cell RCC (ccRCC), there were 16,321 patients and 743,479 controls; for papillary RCC (pRCC), 2,193 patients and 740,816 controls were included. Given the European origin of the exposure variables, we specifically focused on GWAS summary data for KIC, ccRCC, and pRCC in European populations.
Selection of IVs
To identify suitable IVs for this study, the following criteria were applied: (I) SNPs associated with exposures exceeding the GWAS significance threshold of P<5×10−8, and assessment of the F-statistic between IVs and exposure factors to gauge correlation strength, calculated as F=beta2/se2. IVs with F-statistics less than 10 were deemed weak and excluded from subsequent analyses; (II) a clump distance of 10,000 kb and r2<0.001 were set to exclude the effects of linkage disequilibrium; (III) a minimum allele frequency threshold of 0.01 was enforced. Lastly, LDtrait, an online tool for exploring phenotypic associations, was utilized for meticulous SNP analysis, particularly in relation to KIC and its risk factors such as smoking and hypertension (14).
When conducting reverse MR analysis, the criteria for selecting IVs were similar to those used in forward MR analysis.
Statistical analysis
This study used multiple methods to assess the causal association between BMI at various stages and KIC and its subtypes, including inverse variance weighted (IVW), MR Egger regression, weighted median (WM), weighted mode, and simple mode. Our primary focus was on the IVW method due to its ability to provide unbiased causal estimates in the absence of horizontal pleiotropy (15). MR results were reported as odds ratios (ORs) with their corresponding 95% confidence intervals (95% CIs). MR Egger and WM analyses served as supplementary methods to IVW. Reliable results were indicated when the IVW method yielded a P value <0.05 and the OR direction from MR Egger matched that of IVW. If the exposure factor only when an IV, the Wald ratio model was used as the main analysis method.
Sensitivity analysis
A series of sensitivity analyses were used to evaluate the robustness of the causal relationship between early obesity indicators and the risk of KIC. We employed the Cochran Q statistic to evaluate heterogeneity, and utilized a random-effects model to assess significant heterogeneity. The MR-Egger intercept test was used to assess the presence of pleiotropy; significance at P<0.05 indicated pleiotropy. Additionally, we conducted a leave-one-out analysis to evaluate the robustness of our findings.
All analyses were conducted using R version 4.3.1 and relevant packages. Specifically, statistical analysis and plotting utilized packages including TwoSampleMR (version 0.5.7) and forestploter (version 1.1.0).
Results
The selected IVs
Following the removal of linkage disequilibrium and LDtrait scans, we identified a total of 236 SNPs associated with body size, characterized by F statistics ranging from 28.44 to 370.54, confirming the absence of weak IVs. For specific details of the selected IVs, refer to (available online: https://cdn.amegroups.cn/static/public/tau-24-521-1.xlsx).
Causal association between body size traits and KIC and subtypes
Body size traits at various life stages were used as exposure variables, and MR analysis was employed to investigate their potential causal relationship with KIC. The IVW method indicated that childhood obesity (OR =1.08, 95% CI: 1.04–1.14, P<0.001), childhood BMI (OR =1.23, 95% CI: 1.07–1.42, P=0.003), adolescent BMI (OR =1.22, 95% CI: 1.07–1.40, P=0.003), and adult BMI (OR =1.75, 95% CI: 1.41–2.17, P<0.001) were associated with an increased risk of KIC. Consistency in OR direction across different methods was observed (Figure 2).
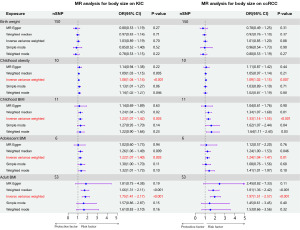
We further examined the causal effects of body size traits on ccRCC and pRCC. Similarly, childhood obesity (OR =1.09, 95% CI: 1.02–1.15, P=0.007), childhood BMI (OR =1.33, 95% CI: 1.14–1.55, P<0.001), adolescent BMI (OR =1.24, 95% CI: 1.04–1.47, P=0.01), and adult BMI (OR =1.97, 95% CI: 1.51–2.57, P<0.001) were associated with an increased risk of ccRCC. Consistent OR directions were observed across all four methods (Figure 2). However, no causal association was found between these traits and pRCC (Table S1).
Sensitivity analysis
The stability of the MR analysis results was evaluated through assessments of heterogeneity, horizontal pleiotropy, and leave-one-out sensitivity. Cochran’s Q test revealed no significant heterogeneity between childhood obesity, childhood BMI, adolescent BMI, and KIC or ccRCC (P>0.05). Similarly, horizontal pleiotropy analysis yielded non-significant findings (P>0.05). Nevertheless, heterogeneity was observed between adult BMI and KIC or ccRCC in adults (P<0.05) (Table 1). The random-effects IVW model was employed to examine the causal relationship between adult BMI and KIC or ccRCC, yielding a P value of <0.001, suggesting minimal impact of heterogeneity on the MR analysis results. Furthermore, a pleiotropic effect analysis for adult BMI showed non-significant results (Table 1).
Table 1
Exposure | Outcome | Heterogeneity | Pleiotropy | |||||
---|---|---|---|---|---|---|---|---|
Method | Cochran’s Q | P value | Egger_intercept | SE | P value | |||
Childhood obesity | Kidney cancer | MR Egger | 6.3491 | 0.61 | – | – | – | |
IVW | 6.6173 | 0.68 | 0.0092 | 0.0178 | 0.62 | |||
Childhood BMI | Kidney cancer | MR Egger | 13.5588 | 0.14 | – | – | – | |
IVW | 13.7191 | 0.19 | 0.0057 | 0.0174 | 0.75 | |||
Adolescent BMI | Kidney cancer | MR Egger | 3.3974 | 0.49 | – | – | – | |
IVW | 3.8701 | 0.57 | 0.0131 | 0.0190 | 0.53 | |||
Adult BMI | Kidney cancer | MR Egger | 122.4899 | <0.001 | – | – | – | |
IVW | 122.5030 | <0.001 | 0.0008 | 0.0107 | 0.94 | |||
Childhood obesity | ccRCC | MR Egger | 6.7327 | 0.57 | – | – | – | |
IVW | 6.7592 | 0.66 | 0.0037 | 0.0227 | 0.87 | |||
Childhood BMI | ccRCC | MR Egger | 7.3264 | 0.60 | – | – | – | |
IVW | 8.2460 | 0.60 | 0.0175 | 0.0182 | 0.36 | |||
Adolescent BMI | ccRCC | MR Egger | 3.2263 | 0.52 | – | – | – | |
IVW | 3.3187 | 0.65 | 0.0074 | 0.0245 | 0.78 | |||
Adult BMI | ccRCC | MR Egger | 114.0979 | <0.001 | – | – | – | |
IVW | 114.4679 | <0.001 | 0.0054 | 0.0133 | 0.69 |
BMI, body mass index; ccRCC, clear cell renal cell carcinoma; IVW, inverse variance weighted; MR, Mendelian randomization; SE, standard error.
Further leave-one-out analyses were conducted to evaluate the individual impact of each SNP on the overall outcome. None of the individual SNPs significantly influenced the MR estimate (Figure 3).
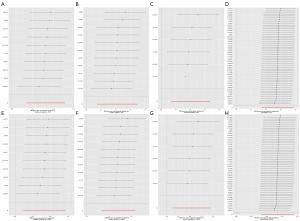
Reverse MR analysis
Reverse MR analysis was conducted to investigate whether KIC or ccRCC had an effect on childhood obesity, childhood BMI, and adult BMI. The results revealed no evidence of associations among them (Table S2). Due to unavailable raw GWAS summary data for adolescent BMI, the Steiger direction test was performed in the forward MR analysis. The results indicated no causal effect of KIC or ccRCC on adolescent BMI (Table S3).
Discussion
Obesity represents a significant global public health concern, which is closely linked to the occurrence and development of cardiovascular diseases and cancer. In this study, we investigated the influence of BMI at various stages on KIC and its subtypes using MR analysis. Our results revealed that genetically predicted childhood obesity, childhood BMI, and adolescent BMI were associated with an elevated risk of KIC and ccRCC in adulthood.
The majority of existing evidence linking obesity to KIC risk is derived from retrospective analyses or observational studies primarily involving adult BMI. Limited research has investigated the impact of body size during early growth stages on KIC. Increasing evidence suggests that elevated body fat during childhood, adolescence, and young adulthood may contribute to increased cancer risks, including thyroid cancer (16), esophageal and gastric cardia cancer (17,18), liver cancer (19), pancreatic cancer (20), colorectal cancer (21,22), endometrial cancer (23,24), and ovarian cancer (25), although it appears to be inversely associated with breast cancer risk (26).
Our findings are consistent with some previous observational studies indicating a positive association between increased BMI in children and adolescents and the risk of KIC (27-30). For instance, Jensen et al. conducted a review involving health records of over 300,000 children aged 7–13 years, documenting weight and height data, and observed a positive association between childhood BMI and RCC risk independent of gender or age (OR =1.14, 95% CI: 1.06–1.23) (27). Bjørge et al. investigated the impact of BMI on KIC risk in a cohort of over 200,000 adolescents aged 14 to 19 years, revealing a significant increase in RCC risk among those with BMI above the 85th percentile (28). Similarly, Leiba et al. studied a cohort of over 1 million male adolescents aged 16 to 19 years, demonstrating a substantially elevated risk of KIC in individuals with a BMI exceeding 27.5 kg/m2 compared to those of normal weight (OR =2.43, 95% CI: 1.54–3.83, P<0.001) (29). Landberg et al. investigated the relationship between BMI and subsequent occurrence of KIC in a cohort of over 230,000 Swedish men aged 18–19 years, finding that one-unit increase in BMI during adolescence was associated with a 6% higher risk of RCC (95% CI: 1.01–1.10) (30). Furthermore, our analysis revealed a higher risk of KIC and ccRCC with increasing BMI in adulthood (31,32).
Recently, evidence has emerged suggesting that birth weight plays a crucial role in future cancer development (33). Several studies have reported a positive association between birth weight and RCC risk [hazard ratio (HR) =1.12, 95% CI: 1.05–1.20 per 500 grams] (27). Bergström et al. founded an elevated risk of KIC among male children with birth weights exceeding 3,500 grams (adjusted OR =1.3, 95% CI: 1.0–1.8) (34). However, our study did not find a causal relationship between birth weight and the risk of KIC.
Elevated BMI is commonly associated with adipose tissue accumulation. Adipose tissue-derived cytokines like interleukin-6 and tumor necrosis factor-α can induce inflammatory responses that contribute to the occurrence and progression of KIC (35,36). Chronic inflammation not only impacts kidney tissue directly but also disrupts the immune system, thereby increasing the risk of KIC. Obesity leads to the release of hormones and growth factors from adipose tissue, such as increased insulin and insulin-like growth factor 1, which promote cell proliferation and resistance to apoptosis, thereby facilitating cancer cell formation and proliferation (37). Furthermore, lipid metabolites and the overproduction of hormones and cytokines like adiponectin and leptin from adipose tissue may directly or indirectly contribute to the occurrence and progression of KIC (38).
In recent years, the impact of obesity on KIC treatment has also received increasing attention. Compared with normal weight population, obesity is associated with higher incidence of perioperative complications. Studies have shown that obese patients are associated with longer operative time, ischemic time, and greater blood loss (39-42). Moreover, obese patients have more perirenal adherent fat, which further contributes to longer operative time and increased blood loss (43,44). The increased abdominal fat tissue can restrict the movement of surgical instruments, which has sparked interest among researchers regarding the choice of surgical approaches for obese populations. For obese patients, the minimally invasive approach has a lower complication rate and length of stay compared with the open approach (45). In comparison to traditional radical nephrectomy, laparoscopic and robot-assisted partial nephrectomy have similar perioperative complication rates with radical nephrectomy, but have more advantages in long-term renal function preservation (46). These findings indicate the potential benefit of minimally invasive partial nephrectomy for obese patients. In terms of prognosis, evidence suggests that high BMI is associated with better tumor outcomes (such as overall survival, cancer specific survival, recurrence free survival), which contradicts the idea that obesity increases the risk of KIC, a phenomenon known as the “obesity paradox” (41,47). Some possible explanations include the higher levels of immune infiltration and increased hypoxia in the peritumoral fat of obese patients, which may trigger a stronger immune response to immunotherapy (48). A recent study found that obesity leads to the expression of programmed death-1 (PD-1) in tumor-associated macrophages, thereby impairing tumor immune surveillance (49). This finding provides a novel insight into how obesity increases cancer risk and enhances response to immunotherapy. However, other studies suggest that obesity may reduce the effectiveness of anti-PD-1 immunotherapy in KIC patients, potentially due to elevated levels of inflammatory cytokines such as interleukin-1 beta (IL-1β) (50).
Reviewing our study, there are several important strengths. First, we employed MR analysis for the first time to investigate the causal impact of birth weight and BMI of childhood, adolescence, and adulthood on KIC and its subtypes, underscoring the significance of early-life obesity in later KIC risk. Second, the comprehensive GWAS summary data for KIC and its subtypes, and the large sample size further enhance the confidence in our current findings. Similarly, the GWAS data for BMI are the most recent available. Nonetheless, there are limitations in our study that should not be ignored. Firstly, due to limitations in exposure and outcome data, replication analyses were not conducted. Second, although we tried to eliminate the influence of potential factors with LDtrait, cancer is the result of multiple factors and multiple steps, and the potential effects of pleiotropy and residual confounding cannot be fully eliminated. Lastly, caution is advised when generalizing our findings, as all data were derived from a European cohort.
Conclusions
In conclusion, our study has established a causal link between increased BMI or obesity during early life and the occurrence of KIC in adulthood. These findings suggest the important role of early-life obesity in the risk of KIC, emphasizing that maintaining a healthy weight in early life has a potentially positive impact on the prevention of KIC in adulthood.
Acknowledgments
We thank the Early Growth Genetics (EGG) Consortium and all investigators for making these GWAS summary statistics.
Footnote
Reporting Checklist: The authors have completed the STROBE-MR reporting checklist. Available at https://tau.amegroups.com/article/view/10.21037/tau-24-521/rc
Peer Review File: Available at https://tau.amegroups.com/article/view/10.21037/tau-24-521/prf
Funding: None.
Conflicts of Interest: All authors have completed the ICMJE uniform disclosure form (available at https://tau.amegroups.com/article/view/10.21037/tau-24-521/coif). The authors have no conflicts of interest to declare.
Ethical Statement: The authors are accountable for all aspects of the work in ensuring that questions related to the accuracy or integrity of any part of the work are appropriately investigated and resolved. The study was conducted in accordance with the Declaration of Helsinki (as revised in 2013).
Open Access Statement: This is an Open Access article distributed in accordance with the Creative Commons Attribution-NonCommercial-NoDerivs 4.0 International License (CC BY-NC-ND 4.0), which permits the non-commercial replication and distribution of the article with the strict proviso that no changes or edits are made and the original work is properly cited (including links to both the formal publication through the relevant DOI and the license). See: https://creativecommons.org/licenses/by-nc-nd/4.0/.
References
- Bray F, Laversanne M, Sung H, et al. Global cancer statistics 2022: GLOBOCAN estimates of incidence and mortality worldwide for 36 cancers in 185 countries. CA Cancer J Clin 2024;74:229-63. [Crossref] [PubMed]
- Chow WH, Dong LM, Devesa SS. Epidemiology and risk factors for kidney cancer. Nat Rev Urol 2010;7:245-57. [Crossref] [PubMed]
- Capitanio U, Montorsi F. Renal cancer. Lancet 2016;387:894-906. [Crossref] [PubMed]
- Bukavina L, Bensalah K, Bray F, et al. Epidemiology of Renal Cell Carcinoma: 2022 Update. Eur Urol 2022;82:529-42. [Crossref] [PubMed]
- Lauby-Secretan B, Scoccianti C, Loomis D, et al. Body Fatness and Cancer--Viewpoint of the IARC Working Group. N Engl J Med 2016;375:794-8. [Crossref] [PubMed]
- Sung H, Siegel RL, Torre LA, et al. Global patterns in excess body weight and the associated cancer burden. CA Cancer J Clin 2019;69:88-112. [Crossref] [PubMed]
- Znaor A, Lortet-Tieulent J, Laversanne M, et al. International variations and trends in renal cell carcinoma incidence and mortality. Eur Urol 2015;67:519-30. [Crossref] [PubMed]
- Smith GD, Ebrahim S. 'Mendelian randomization': can genetic epidemiology contribute to understanding environmental determinants of disease? Int J Epidemiol 2003;32:1-22. [Crossref] [PubMed]
- Warrington NM, Beaumont RN, Horikoshi M, et al. Maternal and fetal genetic effects on birth weight and their relevance to cardio-metabolic risk factors. Nat Genet 2019;51:804-14. [Crossref] [PubMed]
- Vogelezang S, Bradfield JP, Ahluwalia TS, et al. Novel loci for childhood body mass index and shared heritability with adult cardiometabolic traits. PLoS Genet 2020;16:e1008718. [Crossref] [PubMed]
- Graff M, Ngwa JS, Workalemahu T, et al. Genome-wide analysis of BMI in adolescents and young adults reveals additional insight into the effects of genetic loci over the life course. Hum Mol Genet 2013;22:3597-607. [Crossref] [PubMed]
- Locke AE, Kahali B, Berndt SI, et al. Genetic studies of body mass index yield new insights for obesity biology. Nature 2015;518:197-206. [Crossref] [PubMed]
- Purdue MP, Dutta D, Machiela MJ, et al. Multi-ancestry genome-wide association study of kidney cancer identifies 63 susceptibility regions. Nat Genet 2024;56:809-18. [Crossref] [PubMed]
- Lin SH, Brown DW, Machiela MJ. LDtrait: An Online Tool for Identifying Published Phenotype Associations in Linkage Disequilibrium. Cancer Res 2020;80:3443-6. [Crossref] [PubMed]
- Hemani G, Bowden J, Davey Smith G. Evaluating the potential role of pleiotropy in Mendelian randomization studies. Hum Mol Genet 2018;27:R195-208. [Crossref] [PubMed]
- Aarestrup J, Kitahara CM, Baker JL. Birthweight and risk of thyroid cancer and its histological types: A large cohort study. Cancer Epidemiol 2019;62:101564. [Crossref] [PubMed]
- Cook MB, Freedman ND, Gamborg M, et al. Childhood body mass index in relation to future risk of oesophageal adenocarcinoma. Br J Cancer 2015;112:601-7. [Crossref] [PubMed]
- Petrick JL, Jensen BW, Sørensen TIA, et al. Overweight Patterns Between Childhood and Early Adulthood and Esophageal and Gastric Cardia Adenocarcinoma Risk. Obesity (Silver Spring) 2019;27:1520-6. [Crossref] [PubMed]
- Berentzen TL, Gamborg M, Holst C, et al. Body mass index in childhood and adult risk of primary liver cancer. J Hepatol 2014;60:325-30. [Crossref] [PubMed]
- Nogueira L, Stolzenberg-Solomon R, Gamborg M, et al. Childhood body mass index and risk of adult pancreatic cancer. Curr Dev Nutr 2017;1:e001362. [Crossref] [PubMed]
- Jensen BW, Gamborg M, Gögenur I, et al. Childhood body mass index and height in relation to site-specific risks of colorectal cancers in adult life. Eur J Epidemiol 2017;32:1097-106. [Crossref] [PubMed]
- Jensen BW, Bjerregaard LG, Ängquist L, et al. Change in weight status from childhood to early adulthood and late adulthood risk of colon cancer in men: a population-based cohort study. Int J Obes (Lond) 2018;42:1797-803. [Crossref] [PubMed]
- Aarestrup J, Gamborg M, Ulrich LG, et al. Childhood body mass index and height and risk of histologic subtypes of endometrial cancer. Int J Obes (Lond) 2016;40:1096-102. [Crossref] [PubMed]
- Aarestrup J, Gamborg M, Tilling K, et al. Childhood body mass index growth trajectories and endometrial cancer risk. Int J Cancer 2017;140:310-5. [Crossref] [PubMed]
- Aarestrup J, Trabert B, Ulrich LG, et al. Childhood Overweight, Tallness, and Growth Increase Risks of Ovarian Cancer. Cancer Epidemiol Biomarkers Prev 2019;28:183-8. [Crossref] [PubMed]
- Warner ET, Hu R, Collins LC, et al. Height and Body Size in Childhood, Adolescence, and Young Adulthood and Breast Cancer Risk According to Molecular Subtype in the Nurses' Health Studies. Cancer Prev Res (Phila) 2016;9:732-8. [Crossref] [PubMed]
- Jensen BW, Meyle KD, Madsen K, et al. Early life body size in relation to risk of renal cell carcinoma in adulthood: a Danish observational cohort study. Eur J Epidemiol 2020;35:251-8. [Crossref] [PubMed]
- Bjørge T, Tretli S, Engeland A. Relation of height and body mass index to renal cell carcinoma in two million Norwegian men and women. Am J Epidemiol 2004;160:1168-76. [Crossref] [PubMed]
- Leiba A, Kark JD, Afek A, et al. Adolescent obesity and paternal country of origin predict renal cell carcinoma: a cohort study of 1.1 million 16 to 19-year-old males. J Urol 2013;189:25-9. [Crossref] [PubMed]
- Landberg A, Fält A, Montgomery S, et al. Overweight and obesity during adolescence increases the risk of renal cell carcinoma. Int J Cancer 2019;145:1232-7. [Crossref] [PubMed]
- Adams KF, Leitzmann MF, Albanes D, et al. Body size and renal cell cancer incidence in a large US cohort study. Am J Epidemiol 2008;168:268-77. [Crossref] [PubMed]
- Deng Z, Hajihosseini M, Moore JX, et al. Lifetime Body Weight Trajectories and Risk of Renal Cell Cancer: A Large U.S. Prospective Cohort Study. Cancer Epidemiol Biomarkers Prev 2023;32:1651-9. [Crossref] [PubMed]
- Chen C, Chen X, Wu D, et al. Association of birth weight with cancer risk: a dose-response meta-analysis and Mendelian randomization study. J Cancer Res Clin Oncol 2023;149:3925-35. [Crossref] [PubMed]
- Bergström A, Lindblad P, Wolk A. Birth weight and risk of renal cell cancer. Kidney Int 2001;59:1110-3. [Crossref] [PubMed]
- Hursting SD, Dunlap SM. Obesity, metabolic dysregulation, and cancer: a growing concern and an inflammatory (and microenvironmental) issue. Ann N Y Acad Sci 2012;1271:82-7. [Crossref] [PubMed]
- Venkatesh N, Martini A, McQuade JL, et al. Obesity and renal cell carcinoma: Biological mechanisms and perspectives. Semin Cancer Biol 2023;94:21-33. [Crossref] [PubMed]
- Avgerinos KI, Spyrou N, Mantzoros CS, et al. Obesity and cancer risk: Emerging biological mechanisms and perspectives. Metabolism 2019;92:121-35. [Crossref] [PubMed]
- O'Rourke RW. Obesity and cancer: at the crossroads of cellular metabolism and proliferation. Surg Obes Relat Dis 2014;10:1208-19. [Crossref] [PubMed]
- Asif W, Paster IC, Pulling KR, et al. Differential effects of obesity on perioperative outcomes in renal cell carcinoma patients based on race and ethnicity and neighborhood-level socioeconomic status. Transl Androl Urol 2024;13:548-59. [Crossref] [PubMed]
- Ohsugi H, Ikeda J, Takayasu K, et al. Trifecta outcomes of robotic partial nephrectomy in obese patients: A comparison of body mass index <25, 25 to <30, and ≥30. Int J Urol 2024;31:1108-13. [Crossref] [PubMed]
- Ong CSH, Law TYX, Mok A, et al. The impact of body mass index on oncological and surgical outcomes of patients undergoing nephrectomy: a systematic review and meta-analysis. BJU Int 2023;132:608-18. [Crossref] [PubMed]
- Chen XB, Du QL, Zhu PY. Body mass index influence on short-term perioperative results in robotic-assisted laparoscopic partial nephrectomy: a comprehensive systematic review and meta-analysis. J Robot Surg 2024;18:169. [Crossref] [PubMed]
- Fernandez-Pello S, Verma N, Kuusk T, et al. Perioperative impact of body mass index on upper urinary tract and renal robot-assisted surgery: a single high-volume centre experience. J Robot Surg 2022;16:611-9. [Crossref] [PubMed]
- Khene ZE, Dosin G, Peyronnet B, et al. Adherent perinephric fat affects perioperative outcomes after partial nephrectomy: a systematic review and meta-analysis. Int J Clin Oncol 2021;26:636-46. [Crossref] [PubMed]
- Beauval JB, Khene ZE, Roumiguié M, et al. Open versus robotic partial nephrectomy in obese patients: a multi-institutional propensity score-matched analysis (UroCCR 43-Robese study). World J Urol 2024;42:213. [Crossref] [PubMed]
- Lambertini L, Mari A, Sandulli A, et al. Minimally invasive transperitoneal partial versus radical nephrectomy in obese patients: perioperative and long-term functional outcomes from a large perspective contemporary series (RECORd2 project). Minerva Urol Nephrol 2024;76:185-94. [PubMed]
- Kim LH, Doan P, He Y, et al. A Systematic Review and Meta-Analysis of the Significance of Body Mass Index on Kidney Cancer Outcomes. J Urol 2021;205:346-55. [Crossref] [PubMed]
- Sanchez A, Furberg H, Kuo F, et al. Transcriptomic signatures related to the obesity paradox in patients with clear cell renal cell carcinoma: a cohort study. Lancet Oncol 2020;21:283-93. [PubMed]
- Bader JE, Wolf MM, Lupica-Tondo GL, et al. Obesity induces PD-1 on macrophages to suppress anti-tumour immunity. Nature 2024;630:968-75. [PubMed]
- Boi SK, Orlandella RM, Gibson JT, et al. Obesity diminishes response to PD-1-based immunotherapies in renal cancer. J Immunother Cancer 2020;8:e000725. [PubMed]