Butyrate improves testicular spermatogenic dysfunction induced by a high-fat diet
Highlight box
Key findings
• Butyrate supplementation alleviates high-fat diet (HFD)-induced spermatogenic dysfunction in mice by restoring testicular metabolic homeostasis, particularly via normalization of steroid and bile acid synthesis pathways, thereby enhancing sperm quality.
What is known and what is new?
• Obesity impairs male fertility via gut microbiota dysbiosis and metabolic disruptions, while butyrate, a gut-derived short-chain fatty acid, modulates metabolic pathways and has potential reproductive benefits.
• Butyrate affected steroid and bile acid synthesis in the testes, restored HFD-induced spermatogenic dysfunction, and established a link between gut microbiota-derived metabolites, metabolic pathways, and male fertility outcomes.
What is the implication, and what should change now?
• Butyrate improves male fertility in obesity by affecting steroid and bile acid synthesis, suggesting novel targeted metabolic interventions.
Introduction
Over the past few decades, obesity and its associated health problems have increased with the availability of high-calorie, low-nutrient foods and changes in lifestyle (1,2). The global male obesity rate has risen sharply by 28–36% (3). Long-term high-fat diet (HFD) leads to obesity, weight gain, and metabolic disorders such as glucose and lipid metabolism disorders. Many studies have shown that obesity is closely related to low male fertility and decreased sperm quality (4,5). High-energy diets and obesity directly or indirectly affect sperm quality through multiple pathways, including inflammatory response, oxidative stress, disruption of gut microbiota, disruption and remodeling of sperm DNA, and other mechanisms (6). These mechanisms cumulatively cause irreversible damage to sperm. Improving lifestyle is the preferred solution, but it has little effect on some people.
Obesity is often accompanied by dysbiosis of the gut microbiota (DSGM), which not only leads to systemic metabolic disorders but may also affect male fertility through a complex series of mechanisms (7). A number of microbiome-based interventions have been developed to promote health, including prebiotic and synbiotics supplements, FMT, and health-related dietary regimens (8-11). However, while these interventions have shown some health benefits, their effectiveness in altering the microbiome to promote health and generate personalized beneficial responses is often limited. It depends on an individual’s microbiome composition and the difficulty in standardizing fecal microbiota transplantation, which limits clinical application. It is of great significance to clarify the role of intestinal microbial metabolites caused by obesity in male infertility and to intervene in male infertility caused by obesity from the perspective of metabolic products.
Short-chain fatty acids in the gut and the composition of gut microbiota both play key roles in maintaining intestinal homeostasis in testicular injury (12). Short-chain fatty acids, including butyrate, propionate, and acetate, are metabolites produced by intestinal microbiota fermenting dietary fiber in the intestine (13). Animal model studies have confirmed that butyrate can recover obesity and its complications induced by HFD through various mechanisms, such as inhibiting lipid formation, promoting lipid degradation, and regulating mitochondrial function (14-16). Research on the effects of butyric acid on male reproduction is more often used as an intermediate metabolite for intestinal flora transplantation. Rumen bacteria can produce butyrate, which may promote spermatogenesis (17-19). At present, butyrate has become an important small-molecule compound in our personalized dietary supplement. However, its role in the treatment of male infertility is still unclear. This study used the HFD animal model to clarify the effect of dietary supplementation with butyrate and metabolomics on improving semen quality. This study aims to provide experimental evidence for the treatment of abnormal spermatogenesis caused by obesity with butyrate. We present this article in accordance with the ARRIVE reporting checklist (available at https://tau.amegroups.com/article/view/10.21037/tau-2024-660/rc).
Methods
Animals
C57BL/6J male mice, 8 weeks old, were purchased from the Experimental Animal Center of Xuzhou Medical University. Mice were kept in a 22 ℃ constant temperature air-conditioned room, with free access to food and water, and a light/dark cycle of 12 hours. After adapting to laboratory conditions for 1 week, mice were randomly divided into three groups (n=5 per group). In the ND group mice were fed with a normal diet (13.1% fat, 28.7% protein, and 58.2% carbohydrate by weight). In the HFD group mice were fed with HFD (55.2% fat, 14.7% protein, and 30.1% carbohydrate by weight), the 55.2% fat HFD was selected to robustly induce metabolic and inflammatory perturbations, consistent with established models of HFD-induced NAFLD and reproductive dysfunction (20,21). In the HFDB group mice were fed with an HFD supplemented with sodium butyrate (5% butyrate by weight) (22-24). All the groups were given intervention for 12 weeks. The 12-week duration aligns with established models of HFD-induced metabolic and reproductive dysfunction, where aging-related fertility decline minimally impacts outcomes within this timeframe (25-27). All animal care and experiments were carried out following guidelines by the ethics committee of Xuzhou Medical University. A protocol was prepared before the study without registration. This study was approved by the ethics committee of Xuzhou Medical University (No. 202302T006).
Detection of sperm-related indicators in mice
Mice were sacrificed by cervical dislocation. Make an incision in the abdominal cavity, and separate and remove the bilateral epididymides. Place the epididymides in a petri dish containing 3 mL of physiological saline. Use the hematoxylin and eosin (H&E) stain for histopathological observation. Use ophthalmic scissors to mince the epididymides. Gently pipette the suspension 5–6 times with a pipette. Let it stand for 3–5 minutes. Filter out tissue debris using four layers of lens paper to obtain the sperm suspension. The sperm count and motility data of mice were completed using a computer-assisted sperm analysis (CASA). Sperm morphology was assessed via Diff-Quik staining (head, midpiece, or tail defects). Testosterone levels in mouse serum were quantified using a commercially available enzyme-linked immunosorbent assay (ELISA) kit (Beijing North Biotechnology Co., Ltd., China). The cell supernatant was collected, and diluted 50 times, and the testosterone concentration in the supernatant was detected according to the instructions provided with the testosterone detection kit.
Metabolomic analysis
To further determine the impact of an HFD and butyrate on testicular function, we examined the testicular metabolome of mice fed normal diets (ND), HFD, and HFD supplemented with butyrate. A total of 2,588 metabolites were annotated. To extract metabolites from mouse testes, dissected testes tissues are homogenized and lysed in a 20:80 water-methanol solution. After centrifugation to remove insoluble debris, the supernatant containing soluble metabolites is collected and subjected to vacuum concentration using a centrifugal evaporator. Untargeted metabolomics analysis was performed at BGI Shenzhen, China, on a platform consisting of an independent ultra-high performance liquid chromatography-tandem mass spectrometry (UPLC-MS/MS) instrument. In short, each group collected testicular tissue for metabolite extraction. Mass spectrometry data acquisition was performed using a mass spectrometer Xevo G2-XS QTOF (Waters, UK). The raw data from the mass spectrometry instrument was imported into the commercial software Progenesis QI (version 2.2) for peak extraction, obtaining information such as mass-to-charge ratio, retention time, and ion area related to metabolites. Data preprocessing is completed using metaX software (28). To explore the metabolic pathways through which butyrate improves the function of mouse testes, we first used PLS-DA to establish a relationship model between the expression levels of metabolites and the differences between samples. Using the multivariate analysis PLS-DA model, combined with the univariate analysis of differential fold change and P value values, to screen differentially expressed metabolites with VIP >1, P value <0.05, fold change >1.2, or fold change <0.83. The results of differential metabolite analysis for the three sample groups are presented in https://cdn.amegroups.cn/static/public/tau-2024-660-1.xlsx. Pathway enrichment analysis of differential metabolites was completed using MetaboAnalyst 6.0 (29).
Statistical analysis
The data of sperm-related indicators in mice were analyzed using a two-tailed non-paired t-test for difference analysis, with P<0.05 considered to indicate significant differences. Normality and variance equality were assessed using Shapiro-Wilk and Levene’s tests, respectively. Venn diagram was completed using jvenn tool (30). The differential metabolite heatmap was completed using the pheatmap R package. The statistical charts were completed using the ggplot2 R package (31).
Results
Effects of HFD and butyrate on spermatogenesis and development of testicular cells in mice
After 12 weeks of HFD feeding, the body weight of mice in the HFD group significantly increased compared to the ND group, and the addition of butyrate to the HFD (high-fat diet supplemented with butyrate, HFDB) reversed the obesity caused by the HFD (P<0.001, Figure 1A). We further evaluated mature sperm motility and total sperm production, which were isolated from the cauda epididymis. The in vitro analysis of sperm in the epididymis revealed that the concentration of sperm in mice on an HFD was significantly lower than that in mice on a standard diet and that the addition of butyrate to the HFD restored sperm concentration to normal levels (Figure 1B). The total motility was slightly reduced, and the addition of butyrate to an HFD slightly improved the total sperm motility to a certain extent (Figure 1C). This study also found that the sperm tail of mice in the HFD group showed extensive curling, folding, and other malformed sperm, and the head malformation rate also showed a slight increase. We categorized sperm deformities into head and tail abnormalities. The addition of butyrate to the HFD group significantly reduced the malformation rate of both the head and tail of sperm (Figure 1D,1E). In addition, the testosterone levels in the testes of mice significantly decreased in the HFD group, and testosterone levels increased after adding butyrate to the diet (Figure 1F). No substantial differences were observed in the pathology of testis tissue (Figure 1G). These results indicated that an HFD significantly reduced the sperm count in mice, increased the rate of sperm deformity, and decreased sperm motility. However, butyrate was able to improve it to a normal state.
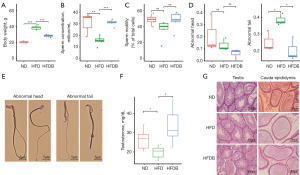
Effects of HFD and butyrate on the testicular metabolome of mice
To further determine the impact of an HFD and butyrate on testicular function, we examined the testicular metabolome of mice fed ND, HFD, and HFD supplemented with butyrate. A total of 2,588 metabolites were annotated. Compared with mice fed ND, the testes of mice fed HFD exhibited 249 differential metabolites, including 173 upregulated and 76 downregulated metabolites (Figure 2A). In comparison to mice fed HFD, the testes of HFDB mice showed 78 differential metabolites, with 37 upregulated and 41 downregulated metabolites (Figure 2B). Between HFDB and ND, a total of 210 differential metabolites were obtained, with 161 upregulated and 50 downregulated (Figure 2C). It was noteworthy that these differential metabolites in two sets (HFD versus ND and HFD versus HFDB) were enriched in primary bile acid biosynthesis, steroid biosynthesis, and taurine and hypotaurine metabolism metabolic pathways at the same time. In addition, these differential metabolites were enriched in the biosynthesis of unsaturated fatty acids, fructose, and mannose metabolism, glycolysis and inositol phosphate metabolism pathways (HFDB versus ND) (Figure 2D).
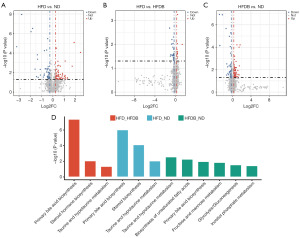
Butyrate improves primary bile acid biosynthesis and steroid biosynthesis metabolic pathways in the testes of mice fed with HFD
The partial least squares discriminant analysis (PLS-DA) showed that the testicular tissues of mice fed ND and those fed HFD exhibited significantly different metabolic states, while some metabolic characteristics in the testicular tissues of mice fed with butyrate tended to the intermediate state between HFD and ND. This suggested that butyrate might improve sperm quality in mice by improving the metabolic status of the testes (Figure 3A).
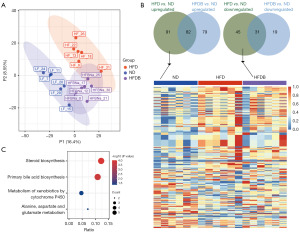
Our study found that compared to ND, 91 metabolites that were upregulated in the testes of HFD mice were elevated to normal levels in the testes of HFDB mice, and 45 metabolites that were downregulated in the testes of mice fed an HFD were elevated to normal levels in the testes of HFDB mice (Figure 3B). These metabolites and butyrate were key metabolites that regulate sperm quality in mice. Using MetaboAnalyst to perform functional enrichment analysis on key differentially expressed metabolites, the results showed that these metabolites were significantly enriched in KEGG pathways related to sperm malformation, including steroid biosynthesis, primary bile acid biosynthesis, metabolism of xenobiotics by cytochrome P450, and alanine, aspartate and glutamate metabolism (Figure 3C). Based on the enrichment results of the HFD vs. HFDB group, we believed that steroid biosynthesis and primary bile acid biosynthesis were important pathways for improving sperm malformation in mice.
Discussion
HFD is a well-established disruptor of metabolic and reproductive health (32). For instance, HFD-induced gut dysbiosis elevates FXR-antagonistic bile acids (e.g., DCA), which may impair testicular FXR signaling and promote inflammation (33,34). This study explored the role of butyrate in recovering spermatogenesis dysfunction caused by obesity by inducing an obese model of male mice with an HFD. It observed that the body weight of HFD group mice increased significantly, while the body weight of HFDB group mice was effectively controlled after adding butyrate. This finding is consistent with previous studies on the anti-obesity potential of butyrate, suggesting that butyrate may prevent or alleviate obesity by regulating energy metabolism (22,35). There was a significant decrease in total sperm motility and a significant increase in sperm deformity, including deformities in the head and tail. These results further confirmed the negative impact of obesity on sperm quality, which is consistent with existing literature reports (36).
Butyrate ameliorates HFD-induced metabolic disorders in animal models. It enhances insulin sensitivity, reduces adiposity, and suppresses inflammation by activating G protein-coupled receptors (e.g., GPR41/43) and inhibiting histone deacetylases (37,38). Butyrate also improves intestinal barrier integrity, mitigating endotoxemia and systemic metabolic inflammation (39). The intervention of butyrate significantly improved this situation. In the HFDB group, the total sperm motility was restored and the malformation rate of the tail was also significantly reduced. The results of metabolomics showed that HFD significantly changed the metabolic status of the testes of mice, involving multiple metabolic pathways. Compared with ND mice, multiple metabolites in the testes of HFD mice were significantly up- or down-regulated. However, after the addition of butyrate, some metabolic characteristics tended towards those of ND mice, especially in the steroid biosynthesis and primary bile acid biosynthesis pathways, which were significantly improved. These metabolic pathways are closely related to sperm malformation and sperm quality.
Steroid biosynthesis is an important pathway for androgen production, and androgens play a key role in spermatogenesis (40). After undergoing HFD in mice, a total of 249 metabolites showed significant changes in the testes, which were significantly enriched in the steroid biosynthesis pathway. The testosterone levels also indicated that after HFD, the testosterone levels in the testes of mice were significantly downregulated. Therefore, we speculated that HFDs might interfere with the steroid biosynthesis pathway, leading to a decrease in androgen levels and subsequently affecting spermatogenesis. Experiments on various animals have shown a close relationship between butyrate and steroid synthesis (41,42). After supplementing butyrate in HFD mice, significant changes were observed in 78 metabolites, including 22(R)-hydroxycholesterol, 20alpha hydroxycholesterol and cholesterol sulfate. These metabolites were significantly enriched in the steroid hormone biosynthesis pathway. The intervention of butyrate may improve sperm quality by restoring the steroid biosynthesis pathway and increasing androgen levels. In addition, the improvement of the primary bile acid biosynthesis pathway may also have a positive effect on sperm production. Zhang et al. used a sheep model of metabolic syndrome induced by a high-energy diet to analyze changes in gut microbiota and vitamin A metabolism. This study found that abnormal metabolism of vitamin A was transmitted to the testes through the bloodstream, further exacerbating sperm production disorders. The absorption of fat-soluble vitamins is affected by the decrease in bile acid levels, and the abnormal metabolism of vitamin A is transferred to testicular cells through circulating blood, resulting in impaired spermatogenesis (43). However, the exact mechanisms need further research.
Although this study provided experimental evidence for the treatment of male infertility with butyrate, there were still some limitations. For example, we only conducted experiments on mouse models, and it remains to be further verified whether these results could be reproduced in humans. A previous study has shown that supplementing with butyrate can improve the reproductive ability of normal animals (44). The effect of butyrate on ND males is also an interesting study and further exploration is needed in future research. In addition, the specific molecular mechanism of butyrate improving sperm quality has not been fully elucidated, and further molecular and cellular biology research is needed to reveal it.
Conclusions
This study shows that obesity induced by an HFD impairs male fertility, reducing sperm count, motility, and increasing malformation rates in mice. Butyrate supplementation recovered these effects, restoring sperm quality. Metabolomic analysis revealed that HFD disrupted testicular metabolic pathways, including steroid and bile acid biosynthesis, while butyrate normalized these changes. These findings highlight butyrate’s potential to mitigate obesity-induced spermatogenic dysfunction by restoring metabolic balance.
Acknowledgments
None.
Footnote
Reporting Checklist: The authors have completed the ARRIVE reporting checklist. Available at https://tau.amegroups.com/article/view/10.21037/tau-2024-660/rc
Data Sharing Statement: Available at https://tau.amegroups.com/article/view/10.21037/tau-2024-660/dss
Peer Review File: Available at https://tau.amegroups.com/article/view/10.21037/tau-2024-660/prf
Funding: This study was supported by
Conflicts of Interest: All authors have completed the ICMJE uniform disclosure form (available at https://tau.amegroups.com/article/view/10.21037/tau-2024-660/coif). The authors have no conflicts of interest to declare.
Ethical Statement: The authors are accountable for all aspects of the work in ensuring that questions related to the accuracy or integrity of any part of the work are appropriately investigated and resolved. All animal care and experiments were carried out following guidelines by the ethics committee of Xuzhou Medical University. This study was approved by ethics committee of Xuzhou Medical University (No. 202302T006).
Open Access Statement: This is an Open Access article distributed in accordance with the Creative Commons Attribution-NonCommercial-NoDerivs 4.0 International License (CC BY-NC-ND 4.0), which permits the non-commercial replication and distribution of the article with the strict proviso that no changes or edits are made and the original work is properly cited (including links to both the formal publication through the relevant DOI and the license). See: https://creativecommons.org/licenses/by-nc-nd/4.0/.
References
- Coronel J, Pinos I, Amengual J. β-carotene in Obesity Research: Technical Considerations and Current Status of the Field. Nutrients 2019;11:842. [Crossref] [PubMed]
- Wadden TA, Tronieri JS, Butryn ML. Lifestyle modification approaches for the treatment of obesity in adults. Am Psychol 2020;75:235-51. [Crossref] [PubMed]
- Ng M, Fleming T, Robinson M, et al. Global, regional, and national prevalence of overweight and obesity in children and adults during 1980-2013: a systematic analysis for the Global Burden of Disease Study 2013. Lancet 2014;384:766-81. [Crossref] [PubMed]
- El-Sawy SA, Amin YA, El-Naggar SA, et al. Artemisia annua L. (Sweet wormwood) leaf extract attenuates high-fat diet-induced testicular dysfunctions and improves spermatogenesis in obese rats. J Ethnopharmacol 2023;313:116528. [Crossref] [PubMed]
- Jia YF, Feng Q, Ge ZY, et al. Obesity impairs male fertility through long-term effects on spermatogenesis. BMC Urol 2018;18:42. [Crossref] [PubMed]
- Pearce KL, Hill A, Tremellen KP. Obesity related metabolic endotoxemia is associated with oxidative stress and impaired sperm DNA integrity. Basic Clin Androl 2019;29:6. [Crossref] [PubMed]
- Chen T, Zhang B, He G, et al. Gut-Derived Exosomes Mediate the Microbiota Dysbiosis-Induced Spermatogenesis Impairment by Targeting Meioc in Mice. Adv Sci (Weinh) 2024;11:e2310110. [Crossref] [PubMed]
- Ribera C, Sánchez-Ortí JV, Clarke G, et al. Probiotic, prebiotic, synbiotic and fermented food supplementation in psychiatric disorders: A systematic review of clinical trials. Neurosci Biobehav Rev 2024;158:105561. [Crossref] [PubMed]
- Sergeev IN, Aljutaily T, Walton G, et al. Effects of Synbiotic Supplement on Human Gut Microbiota, Body Composition and Weight Loss in Obesity. Nutrients 2020;12:222. [Crossref] [PubMed]
- Porcari S, Benech N, Valles-Colomer M, et al. Key determinants of success in fecal microbiota transplantation: From microbiome to clinic. Cell Host Microbe 2023;31:712-33. [Crossref] [PubMed]
- Patterson RE, Sears DD. Metabolic Effects of Intermittent Fasting. Annu Rev Nutr 2017;37:371-93. [Crossref] [PubMed]
- Hao Y, Feng Y, Yan X, et al. Gut microbiota-testis axis: FMT improves systemic and testicular micro-environment to increase semen quality in type 1 diabetes. Mol Med 2022;28:45. [Crossref] [PubMed]
- Dalile B, Van Oudenhove L, Vervliet B, et al. The role of short-chain fatty acids in microbiota-gut-brain communication. Nat Rev Gastroenterol Hepatol 2019;16:461-78. [Crossref] [PubMed]
- Hong J, Jia Y, Pan S, et al. Butyrate alleviates high fat diet-induced obesity through activation of adiponectin-mediated pathway and stimulation of mitochondrial function in the skeletal muscle of mice. Oncotarget 2016;7:56071-82. [Crossref] [PubMed]
- Arnoldussen IAC, Wiesmann M, Pelgrim CE, et al. Butyrate restores HFD-induced adaptations in brain function and metabolism in mid-adult obese mice. Int J Obes (Lond) 2017;41:935-44. [Crossref] [PubMed]
- Jia Y, Hong J, Li H, et al. Butyrate stimulates adipose lipolysis and mitochondrial oxidative phosphorylation through histone hyperacetylation-associated β3 -adrenergic receptor activation in high-fat diet-induced obese mice. Exp Physiol 2017;102:273-81. [Crossref] [PubMed]
- Zhang P, Feng Y, Li L, et al. Improvement in sperm quality and spermatogenesis following faecal microbiota transplantation from alginate oligosaccharide dosed mice. Gut 2021;70:222-5. [Crossref] [PubMed]
- Zhang C, Xiong B, Chen L, et al. Rescue of male fertility following faecal microbiota transplantation from alginate oligosaccharide-dosed mice. Gut 2021;70:2213-5. [Crossref] [PubMed]
- Hao Y, Feng Y, Yan X, et al. Gut Microbiota-Testis Axis: FMT Mitigates High-Fat Diet-Diminished Male Fertility via Improving Systemic and Testicular Metabolome. Microbiol Spectr 2022;10:e0002822. [Crossref] [PubMed]
- Mattace Raso G, Simeoli R, Russo R, et al. Effects of sodium butyrate and its synthetic amide derivative on liver inflammation and glucose tolerance in an animal model of steatosis induced by high fat diet. PLoS One 2013;8:e68626. [Crossref] [PubMed]
- Zhou D, Pan Q, Xin FZ, et al. Sodium butyrate attenuates high-fat diet-induced steatohepatitis in mice by improving gut microbiota and gastrointestinal barrier. World J Gastroenterol 2017;23:60-75. [Crossref] [PubMed]
- Gao Z, Yin J, Zhang J, et al. Butyrate improves insulin sensitivity and increases energy expenditure in mice. Diabetes 2009;58:1509-17. [Crossref] [PubMed]
- Beisner J, Filipe Rosa L, Kaden-Volynets V, et al. Prebiotic Inulin and Sodium Butyrate Attenuate Obesity-Induced Intestinal Barrier Dysfunction by Induction of Antimicrobial Peptides. Front Immunol 2021;12:678360. [Crossref] [PubMed]
- Kaden-Volynets V, Günther C, Zimmermann J, et al. Deletion of the Casp8 gene in mice results in ileocolitis, gut barrier dysfunction, and malassimilation, which can be partially attenuated by inulin or sodium butyrate. Am J Physiol Gastrointest Liver Physiol 2019;317:G493-507. [Crossref] [PubMed]
- Podrini C, Cambridge EL, Lelliott CJ, et al. High-fat feeding rapidly induces obesity and lipid derangements in C57BL/6N mice. Mamm Genome 2013;24:240-51. [Crossref] [PubMed]
- Ravaut G, Carneiro A, Mounier C. Exploring the impacts of ketogenic diet on reversible hepatic steatosis: initial analysis in male mice. Front Nutr 2024;11:1290540. [Crossref] [PubMed]
- Szabó R, Börzsei D, Hoffmann A, et al. The Interplay of Lifestyle and Adipokines in the Non-Obese Stroke-Prone Spontaneously Hypertensive Rats. Antioxidants (Basel) 2023;12:1450. [Crossref] [PubMed]
- Wen B, Mei Z, Zeng C, et al. metaX: a flexible and comprehensive software for processing metabolomics data. BMC Bioinformatics 2017;18:183. [Crossref] [PubMed]
- Pang Z, Lu Y, Zhou G, et al. MetaboAnalyst 6.0: towards a unified platform for metabolomics data processing, analysis and interpretation. Nucleic Acids Res 2024;52:W398. [Crossref] [PubMed]
- Bardou P, Mariette J, Escudié F, et al. jvenn: an interactive Venn diagram viewer. BMC Bioinformatics 2014;15:293. [Crossref] [PubMed]
- Ginestet C. ggplot2: Elegant Graphics for Data Analysis. J R Stat Soc Ser A Stat Soc 2011;174:245-6. [Crossref]
- Ding N, Zhang X, Zhang XD, et al. Impairment of spermatogenesis and sperm motility by the high-fat diet-induced dysbiosis of gut microbes. Gut 2020;69:1608-19. [Crossref] [PubMed]
- Shi L, Jin L, Huang W. Bile Acids, Intestinal Barrier Dysfunction, and Related Diseases. Cells 2023;12:1888. [Crossref] [PubMed]
- Luo Z, Zhou W, Xie T, et al. The role of botanical triterpenoids and steroids in bile acid metabolism, transport, and signaling: Pharmacological and toxicological implications. Acta Pharm Sin B 2024;14:3385-415. [Crossref] [PubMed]
- Chen Y, Xu C, Huang R, et al. Butyrate from pectin fermentation inhibits intestinal cholesterol absorption and attenuates atherosclerosis in apolipoprotein E-deficient mice. J Nutr Biochem 2018;56:175-82. [Crossref] [PubMed]
- Li Y, Lin Y, Ou C, et al. Association between body mass index and semen quality: a systematic review and meta-analysis. Int J Obes (Lond) 2024;48:1383-401. [Crossref] [PubMed]
- Abildinova GZ, Benberin VV, Vochshenkova TA, et al. Global trends and collaborative networks in gut microbiota-insulin resistance research: a comprehensive bibliometric analysis (2000-2024). Front Med (Lausanne) 2024;11:1452227. [Crossref] [PubMed]
- Lin Z, Sun L. Research advances in the therapy of metabolic syndrome. Front Pharmacol 2024;15:1364881. [Crossref] [PubMed]
- Maiuolo J, Bulotta RM, Ruga S, et al. The Postbiotic Properties of Butyrate in the Modulation of the Gut Microbiota: The Potential of Its Combination with Polyphenols and Dietary Fibers. Int J Mol Sci 2024;25:6971. [Crossref] [PubMed]
- Naamneh Elzenaty R, du Toit T, Flück CE. Basics of androgen synthesis and action. Best Pract Res Clin Endocrinol Metab 2022;36:101665. [Crossref] [PubMed]
- Liu Q, Ma R, Li S, et al. Dietary Supplementation of Auricularia auricula-judae Polysaccharides Alleviate Nutritional Obesity in Mice via Regulating Inflammatory Response and Lipid Metabolism. Foods 2022;11:942. [Crossref] [PubMed]
- Li X, Sha Y, Li S, et al. Dietary resveratrol improves immunity and antioxidant defense in ewes by regulating the rumen microbiome and metabolome across different reproductive stages. Front Immunol 2024;15:1462805. [Crossref] [PubMed]
- Zhang T, Sun P, Geng Q, et al. Disrupted spermatogenesis in a metabolic syndrome model: the role of vitamin A metabolism in the gut-testis axis. Gut 2022;71:78-87. [Crossref] [PubMed]
- Wang J, Zhang H, Bai S, et al. Dietary tributyrin improves reproductive performance, antioxidant capacity, and ovary function of broiler breeders. Poult Sci 2021;100:101429. [Crossref] [PubMed]