Prognostic impact of early versus delayed loop diuretic administration in sepsis: a propensity score-matched analysis using the MIMIC-IV database
Highlight box
Key findings
• This study found that early use of diuretics in septic patients impairs renal function and increases mortality.
What is known and what is new?
• Fluid overload can impair organ function, and reverse fluid resuscitation is crucial.
• The optimal timing for reverse fluid resuscitation remains unclear, and the safety and efficacy of loop diuretics are controversial.
What is the implication, and what should change now?
• During the early phase of fluid management in septic patients, careful assessment of fluid status is essential, and the use of diuretics should be approached with caution.
IntroductionOther Section
Aggressive fluid resuscitation has become a cornerstone of sepsis management (1). Sepsis induces hypovolemia through mechanisms such as increased vascular permeability and reduced vascular tone. Early fluid resuscitation enhances cardiac output, optimizes tissue perfusion, and has been associated with better clinical outcomes in patients with sepsis (2). Fluid therapy is generally categorized into four overlapping phases: resuscitation, optimization, stabilization, and evacuation (ROSE) (3). However, the first three phases often result in substantial fluid accumulation, and increasing evidence indicates that fluid overload may impair multiple organ systems (4) and is associated with increased mortality (5,6). Therefore, the process of deresuscitation requires careful consideration to avoid further complications.
Loop diuretics are frequently used during the deresuscitation phase; however, the optimal timing for initiating this process remains uncertain (7). The safety and efficacy of loop diuretics in this context remain subjects of ongoing debate (8). Premature initiation of deresuscitation may lead to insufficient resuscitation, resulting in compromised perfusion, tissue hypoxia, organ dysfunction, and potentially increased mortality (9). Thus, determining the optimal timing for deresuscitation is critical. Loop diuretics are widely utilized in intensive care unit (ICU) settings to promote diuresis, reduce fluid retention, and alleviate pulmonary edema (10,11). However, the evidence regarding their impact on mortality and the occurrence of severe adverse events in fluid-overloaded patients remains inconclusive (8).
The objective of this study was to examine the effect of the timing of loop diuretic administration on the prognosis of patients with sepsis. We present this article in accordance with the STROBE reporting checklist (available at https://tau.amegroups.com/article/view/10.21037/tau-24-620/rc).
MethodsOther Section
Database overview
This retrospective, observational study utilized data from the Medical Information Mart for Intensive Care IV (MIMIC-IV) database, which contains comprehensive clinical information on patients admitted to Beth Israel Deaconess Medical Center (BIDMC) between 2008 and 2019. The database was selected as the primary data source due to its public availability and free access. Patient identities are anonymized through random coding to ensure privacy, eliminating the need for ethical approval or informed consent. Data access and collection were performed by Y.W., the author, who was granted access following completion of the necessary training and certification process. The study was conducted in accordance with the Declaration of Helsinki (as revised in 2013).
Inclusion and exclusion criteria
Inclusion criteria: Patients who met the Sepsis-3.0 definition were included in this study. Specifically, individuals suspected of having an infection and a Sequential Organ Failure Assessment (SOFA) score greater than two (12) were identified from the MIMIC-IV database for inclusion. Exclusion criteria: For patients with multiple ICU admissions, only data from the first ICU admission were considered. Patients were excluded if they did not receive loop diuretics, received loop diuretics were first administered between 48 and 96 hours after sepsis diagnosis, or if they died within 24 hours of sepsis diagnosis.
Data extraction
Data extracted from the MIMIC-IV database included demographic characteristics (gender, weight, and age), disease severity (as indicated by the SOFA score), laboratory results (such as platelet count, white blood cell count, C-reactive protein, lactate, blood sodium, creatinine, and urea nitrogen), comorbidities [including hypertension and chronic obstructive pulmonary disease (COPD)], use of vasoactive medications, 48-hour fluid intake, 48-hour urine output, 48-hour fluid balance, and blood purification treatments like continuous renal replacement therapy (CRRT), mechanical ventilation treatment, diuretic dosage, and mean arterial pressure (MAP).
Relevant definitions
Early loop diuretic use was defined as the initiation of any loop diuretic, including torasemide, bumetanide, or furosemide, within 48 hours of sepsis diagnosis. Late loop diuretic use was defined as the initiation of these diuretics 96 hours or more after sepsis diagnosis. The criteria for AKI were based on the Kidney Disease: Improving Global Outcomes (KDIGO) guidelines, which included one or more of the following: a creatinine increase to more than 1.5 times the baseline level during the ICU stay, urine output less than 0.5 mg/(kg·h) for over six hours, or a creatinine increase of more than 26.5 µmol/L within 48 hours.
Outcomes
The primary outcome of this study was 28-day mortality. Secondary outcomes included the in-hospital mortality rate and the incidence of acute kidney injury (AKI).
Propensity score matching (PSM)
PSM was used to minimize the influence of confounding factors, such as disease severity, comorbidities, laboratory results, and fluid intake, on the study outcomes. Propensity scores were generated through a multivariate logistic regression model and used to match patients who received loop diuretics. A 1:1 nearest neighbor matching algorithm with a caliper width of 0.02 was applied. Matching was performed based on variables such as gender, age, weight, initial SOFA score at ICU admission, peak SOFA score, 48-hour fluid intake, cumulative 48-hour norepinephrine and dobutamine, creatinine levels, white blood cell count, blood sodium, platelet count, and history of conditions such as hypertension and COPD. The quality of the matching process was assessed using Kernel density estimation, resulting in 1,882 matched pairs for subsequent analysis.
Handling missing data
The MIMIC-IV database, given its large volume of data, inevitably contains missing values. To minimize potential bias, missing values for continuous variables with less than 5% missing data were imputed using the mean or median of the respective variable. For variables with more than 20% missing data, no imputation was conducted.
Statistical analysis
The histogram command in STATA was used to visualize continuous variables and assess their normality. Continuous variables are presented as either mean ± standard deviation or median (interquartile range), with group comparisons conducted using either the t-test or the Mann-Whitney U test, depending on the data distribution. Categorical variables are expressed as percentages, and differences between groups were conducted using the Chi-squared test. The 28-day survival rates between the early and late groups were compared using Kaplan-Meier curves, with the log-rank test applied for statistical assessment. Further comparisons were made after adjusting for potential confounding factors. The relationship between loop diuretic use and 28-day mortality was assessed using Cox proportional hazards regression. The process for selecting confounding factors involved: initially conducting univariate Cox regression analysis to include variables with P values less than 0.05 in the multivariate Cox regression model, followed by a stepwise backward selection procedure to finalize the model. The proportional hazards assumption for the final model was assessed to calculate the adjusted hazard ratio (HR). PSM was applied to balance confounding variables between groups, reducing the potential for bias. A two-tailed test was used, with statistical significance defined as a P value less than 0.05 (P<0.05). All statistical analyses were conducted using Stata 16.
ResultsOther Section
Baseline characteristics of included patients
The baseline characteristics of the 8,518 patients included in this study are summarized in Table 1. Patients in the late group generally presented with more critical conditions compared to those in the early group. The late group had a higher initial SOFA score upon ICU admission {6 [4–9] vs. 5 [3–7], P<0.001} and a higher peak SOFA score during their ICU stay {8 [5–10] vs. 6 [4–8], P<0.001} compared to the early group, with these differences being statistically significant. During the first 48 hours following sepsis diagnosis, the late group received a greater volume of fluid {6,655 [4,055–9,984] vs. 4,331 [2,696–6,277], P<0.001}. Although the early group initiated diuretic therapy sooner to intervene in fluid balance, there was no statistically significant difference in urine output between the two groups (P=0.12). Consequently, the late group had a larger positive fluid balance over the 48-hour period {3,251 (605–6,668) vs. 965 (−501 to 2,742), P<0.001}. In terms of respiratory function, 75% of patients in the early group required invasive mechanical ventilation, which is significant lower than that in the late group. Regarding circulatory function, patients in the early diuretic group had a significantly lower overall MAP, and no change in MAP was observed before and after diuretic administration. As for the 28-day mortality rate, the early group had a significantly higher rate of 18.5%, compared to only 16.8% in the late group (P=0.03). The proportion of patients developing AKI and the proportion of patients in AKI stage 3 were similar between the two groups (P>0.05).
Table 1
Demographics | ≤48 h (n=4,485) | ≥96 h (n=4,033) | All patients (n=8,518) | P |
---|---|---|---|---|
Age (years) | 68.8±14.2 | 66.8±15.8 | 67.8±15.0 | <0.001 |
Male, n (%) | 2,728 (60.8) | 2,319 (57.5) | 5,047 (59.2) | 0.002 |
Weight (kg) | 83.6±22.4 | 84.3±25.3 | 83.9±23.8 | 0.19 |
Comorbidities, n (%) | ||||
Hypertension | 2,174 (48.4) | 1,730 (42.8) | 3,904 (45.8) | <0.001 |
COPD | 248 (5.5) | 247 (6.0) | 495 (5.8) | 0.24 |
Laboratory tests | ||||
Maximum WBC (×109/L) | 16.7±7.3 | 19.2±9.0 | 17.9±8.3 | <0.001 |
Min Plt (×109/L) | 139.4±76.9 | 136.2±82.2 | 137.9±79.5 | 0.06 |
Maximum CRP (mmol/L) | 99.1±78.0 | 111.5±82.0 | 107.9±81.0 | 0.08 |
Maximum Bun (mg/dL) | 37.9±26.9 | 48.2±30.8 | 42.8±29.3 | <0.001 |
Maximum Crea (mg/dL) | 1.8±1.7 | 2.0±1.7 | 1.9±1.7 | <0.001 |
Maximum Na (mmol/L) | 142.1±4.7 | 145.9±5.8 | 143.9±5.6 | <0.001 |
Maximum Lac (mmol/L) | 3.1±2.5 | 3.3±2.7 | 3.2±2.6 | <0.001 |
Fluid balance | ||||
48 h fluid intake (mL) | 4,331 [2,696–6,277] | 6,655 [4,055–9,984] | 5,160 [3,176–8,144] | <0.001 |
48 h urine output (mL) | 3,040 [1,902–4,600] | 2,960 [1,875–4,435] | 3,005 [1,885–4,520] | 0.12 |
48 h fluid balance (mL) | 965 [–501 to 2,742] | 3,251 [605–6,668] | 1,755 [–145 to 4,673] | <0.001 |
Vasopressors | ||||
Number of patients using vasopressors, n (%) | 1,223 (27.2) | 1,650 (40.9) | 2,873 (33.7) | <0.001 |
Norepinephrine (mg) in 48 h | 3.6±13.7 | 7.5±19.2 | 5.4±16.7 | <0.001 |
Dopamine (mg) in 48 h | 13.3±128.2 | 12.9±120.6 | 13.2±124.7 | 0.87 |
Dobutamine (mg) in 48 h | 24.3±227.4 | 21.9±202.7 | 23.2±216.1 | 0.60 |
Epinephrine (mg) in 48 h | 0.33±4.5 | 0.32±5.0 | 0.33±4.8 | 0.94 |
MAP | ||||
Mean MAP before diuretic use (mmHg) | 76.3±9.5 | 79.5±11.6 | 77.8±10.6 | <0.001 |
Mean MAP after diuretic use (mmHg) | 75.9±10.4 | 79.0±11.6 | 77.4±11.1 | <0.001 |
Max MAP before diuretic use (mmHg) | 98 [89–110] | 104 [92–118] | 101 [90–114] | <0.001 |
Max MAP after diuretic use (mmHg) | 97 [86–112] | 104 [92–118] | 101 [89–115] | <0.001 |
Min MAP before diuretic use (mmHg) | 57.3±14.3 | 59.6±13.9 | 58.4±14.2 | <0.001 |
Min MAP after diuretic use (mmHg) | 57.3±14.4 | 58.6±14.8 | 57.9±14.6 | <0.001 |
Special treatment, n (%) | ||||
CRRT within 48 h | 127 (2.8) | 83 (2.0) | 210 (2.4) | 0.02 |
Invasive mechanical ventilation | 3,365 (75.0) | 3,454 (85.6) | 6,819 (80.0) | <0.001 |
Severity of illness | ||||
Maximum SOFA | 6 [4–8] | 8 [5–10] | 7 [5–9] | <0.001 |
Initial SOFA | 5 [3–7] | 6 [4–9] | 5 [3–8] | <0.001 |
Clinical outcomes, n (%) | ||||
28-day mortality rate | 832 (18.5) | 679 (16.8) | 1,511 (17.7) | 0.03 |
In-hospital mortality rate | 676 (15.0) | 640 (15.8) | 1,316 (15.4) | 0.31 |
AKI rate | 1,085 (24.1) | 1,001 (24.8) | 2,086 (24.4) | 0.50 |
AKI3 rate | 244 (5.4) | 251 (6.2) | 495 (5.8) | 0.12 |
For continuous variables, data that are normally distributed are expressed as mean ± standard deviation, while non-normally distributed data are presented as the median [interquartile range]. AKI, acute kidney injury; Bun, blood urea nitrogen; COPD, chronic obstructive pulmonary disease; Crea, creatinine; CRP, C-reactive protein; CRRT, continuous renal replacement therapy; Lac, lactate; MAP, mean arterial pressure; Plt, platelet count; SOFA, Sequential Organ Failure Assessment; WBC, white blood cell count.
Kaplan-Meier survival curve
Kaplan-Meier survival curves were generated to assess the effect of diuretic administration timing on 28-day cumulative survival rates. The analysis indicated that the early group had a significantly lower survival rate compared to the late group (Figure 1). Furthermore, after adjusting for multiple confounding factors, the Kaplan-Meier curve revealed no significant change in the outcome, indicating that the observed survival difference persisted between the two groups (Figure 2). Additionally, Kaplan-Meier curves were used to evaluate the impact of diuretic dosage on the 28-day cumulative survival rate. The results indicate that as diuretic dosage increased, survival rates gradually improved (Figure 3).
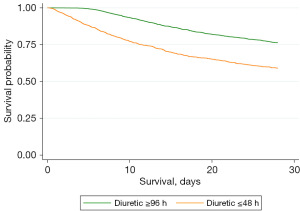
Cox regression analysis
The influence of various factors on the 28-day mortality rate was assessed using a univariate Cox proportional hazards model. Statistically significant variables (P<0.05) included early diuretic use, age, gender, weight, initial SOFA score, peak SOFA score, peak blood sodium, peak creatinine, peak white blood cell count, minimum platelet count, high blood pressure, COPD, 48-hour urine output, 48-hour fluid balance, cumulative 48-hour norepinephrine, and cumulative 48-hour total dobutamine. These variables were incorporated into a multivariate regression model. A stepwise backward selection method was applied to refine the model assessing 28-day mortality. The results, as detailed in Table 2, indicated that the early group had a significantly higher risk of 28-day mortality compared to the late group (HR =2.590, 95% CI: 2.325–2.884, P<0.001). After adjusting for the proportional hazards assumption using a correction factor, the HR was recalculated as exp[3.55–1.20 × ln(t)].
Table 2
Variable | Univariate model | Multivariate model | |||||
---|---|---|---|---|---|---|---|
HR | 95% CI | P | HR | 95% CI | P | ||
Diuretics | 2.354 | 2.123–2.611 | <0.001 | 2.590 | 2.325–2.884 | <0.001 | |
Age | 1.021 | 1.018–1.025 | <0.001 | 1.020 | 1.016–1.024 | <0.001 | |
Sex | 0.818 | 0.740–0.906 | <0.001 | – | – | – | |
Weight | 0.992 | 0.990–0.994 | <0.001 | 0.994 | 0.991–0.996 | <0.001 | |
Initial SOFA | 1.097 | 1.083–1.111 | <0.001 | – | – | – | |
Max SOFA | 1.123 | 1.111–1.136 | <0.001 | 1.188 | 1.169–1.207 | <0.001 | |
Maximum Na | 0.994 | 0.985–1.003 | 0.23 | – | – | – | |
Maximum Crea | 1.094 | 1.071–1.117 | <0.001 | 0.948 | 0.920–0.976 | <0.001 | |
Maximum WBC (×109/L) | 1.017 | 1.012–1.022 | <0.001 | – | – | – | |
Min Plt (×109/L) | 0.998 | 0.998–0.999 | <0.001 | 1.002 | 1.001–1.002 | <0.001 | |
Hypertension | 0.808 | 0.728–0.897 | <0.001 | – | – | – | |
COPD | 1.504 | 1.265–1.789 | <0.001 | 1.311 | 1.101–1.560 | 0.002 | |
48 h urine output | 0.999 | 0.999–0.999 | <0.001 | 0.999 | 0.999–0.999 | <0.001 | |
48 h fluid balance | 1.000 | 1.000–1.000 | <0.001 | 0.999 | 0.999–0.999 | <0.001 | |
48 h norepinephrine | 1.000 | 1.000–1.011 | <0.001 | 1.008 | 1.006–1.011 | <0.001 | |
48 h dopamine | 1.000 | 1.000–1.000 | <0.001 | 1.000 | 1.000–1.000 | 0.004 |
CI, confidence interval; COPD, chronic obstructive pulmonary disease; Crea, creatinine; HR, hazard ratio; Plt, platelet count; SOFA, Sequential Organ Failure Assessment; WBC, white blood cell count.
Results of PSM analysis
After applying a 1:1 matching algorithm through PSM, 1,882 cases in each group were successfully matched, resulting in a well-balanced sample (Table 3). The quality of the matched sample was assessed by comparing standardized mean differences and variance ratios of the propensity scores between the groups, as well as through a graphical examination of the propensity score distributions (Figure 4). No significant differences were observed between the groups across all 14 covariates, including age, weight, initial SOFA score, peak SOFA score, fluid intake, and use of vasoactive medication. Subsequent analysis indicated that the early group had a significantly higher 28-day mortality rate (27.3% vs. 18.8%), and in-hospital mortality rates (22.8% vs. 17.3%) compared to the late group. Additionally, the proportion of patients in stage 3 AKI was significantly higher in the early group (8.1% vs. 5.7%, P=0.004).
Table 3
Variables | ≤48 h (n=1,882) | ≥96 h (n=1,882) | P |
---|---|---|---|
Male, n (%) | 1,054 (56.0) | 1,065 (56.6) | 0.71 |
Age (years) | 67.6±15.5 | 68.1±15.4 | 0.41 |
Weight (kg) | 83.6±23.8 | 83.6±25.8 | 0.97 |
Initial SOFA | 6 [4–8] | 6 [4–8] | 0.51 |
Max SOFA | 7 [5–10] | 7 [5–10] | 0.96 |
48 h fluid intake (mL) | 5,530 [3,513–8,256] | 5,733 [3,503–8,510] | 0.48 |
Maximum Na (mmol/L) | 144.7±5.3 | 144.5±5.1 | 0.34 |
Maximum Crea (mg/dL) | 2.1±1.9 | 1.9±1.7 | 0.06 |
Maximum WBC (×109/L) | 18.3±8.3 | 18.1±8.4 | 0.41 |
Min Plt (×109/L) | 143.6±88.0 | 140.8±83.5 | 0.31 |
COPD, n (%) | 115 (6.1) | 120 (6.4) | 0.73 |
HBP, n (%) | 802 (42.6) | 815 (43.3) | 0.66 |
Norepinephrine (mg) in 48 h | 6.0±18.0 | 5.6±15.5 | 0.45 |
Dobutamine (mg) in 48 h | 15.4±131.3 | 15.9±146.6 | 0.90 |
Outcomes | |||
28-day mortality rate, n (%) | 514 (27.3) | 353 (18.8) | <0.001 |
In-hospital mortality rate, n (%) | 429 (22.8) | 326 (17.3) | <0.001 |
48 h urine output (mL) | 3,949±2,444 | 3,233±2,066 | <0.001 |
AKI3 stage rate, n (%) | 153 (8.1) | 108 (5.7) | 0.004 |
AKI rate, n (%) | 518 (27.5) | 482 (25.6) | 0.18 |
Matching parameters: gender, age, weight, initial SOFA, peak SOFA, peak blood sodium, peak creatinine, peak white blood cell count, minimum platelet count, COPD, HBP, cumulative 48-hour norepinephrine, cumulative 48-hour dobutamine. For continuous variables, data that are normally distributed are expressed as mean ± standard deviation, while non-normally distributed data are presented as the median [interquartile range]. AKI, acute kidney injury; COPD, chronic obstructive pulmonary disease; Crea, creatinine; HBP, high blood pressure; Plt, platelet count; PSM, propensity score matching; SOFA, Sequential Organ Failure Assessment; WBC, white blood cell count.
DiscussionOther Section
The findings of this study indicate that both 28-day mortality rates were significantly higher in patients who received loop diuretics within 48 hours of sepsis diagnosis (early group) compared to those who initiated diuretic therapy 96 hours or more after diagnosis (late group). After PSM and covariate adjustment, both the 28-day mortality rate and in-hospital mortality rate increased. Furthermore, early application of diuretics may exacerbate kidney function deterioration. While the influence of certain confounding factors cannot be entirely excluded, these findings suggest that early diuretic use in patients with sepsis should be approached with caution. Further clinical studies are necessary to validate these findings.
With the increasing adoption of Rivers’ early goal-directed therapy (EGDT) for fluid resuscitation (2), aggressive fluid resuscitation has become a widely accepted practice in the management of sepsis. However, a growing body of research indicates that EGDT may not enhance outcomes in patients with sepsis (13-15). Moreover, aggressive fluid administration often results in fluid overload, which is associated with various forms of organ dysfunction, including cerebral, pulmonary, cardiac, and intestinal edema, and may contribute to increased mortality (16,17). Consequently, there has been a growing shift towards restrictive fluid management strategies, which have garnered greater attention and support. For instance, a study comparing conservative and liberal fluid management in patients with acute lung injury found that a conservative approach led to enhanced lung function, reduced ventilator duration, and no increase in extrapulmonary organ failure (6). The CLASSIC study further examined the impact of restrictive fluid management versus standard care in patients with septic shock following initial resuscitation. This study found that the restrictive fluid management group received a significantly lower volume of fluids and exhibited a reduced rate of AKI exacerbation (18). Additionally, a meta-analysis of restrictive fluid management strategies suggested that this approach was associated with shorter ICU stays and extended periods without the need for mechanical ventilation (19).
While restrictive fluid management has demonstrated certain potential advantages, it is crucial to recognize that it has not consistently shown a significant impact on enhancing long-term patient outcomes. For instance, a multicenter study conducted in the United States found that among patients with sepsis-induced hypotension, a restrictive fluid resuscitation strategy did not significantly alter the 90-day mortality rate when compared to a liberal fluid resuscitation approach (20). Similarly, another study exploring the effects of restrictive fluid management on long-term outcomes reported no significant differences in survival rates or cognitive function between restrictive and liberal fluid resuscitation strategies (21). These findings are consistent with several other studies (22).
Given the potential benefits of restrictive fluid management, efforts to prevent or reduce fluid overload after initial resuscitation have become increasingly important. However, there is currently no standardized approach for defining fluid overload. Some definitions have relied on changes in patient weight (17), but this metric is often impractical in clinical settings. Additionally, clinicians use various methods and indicators to assess fluid overload (23), leading to variability in assessments. Even among different clinicians assessing the same patient, there may be disagreements about whether fluid overload is present (24). These inconsistencies can significantly influence decisions regarding the timing of diuretic therapy. Premature intervention risks inducing hypovolemia, which can further compromise patient stability, necessitating carefully consideration (3).
Sepsis can be initiated by a wide range of pathogens, leading to dysregulated inflammatory and immune responses that can damage vascular endothelial cells and increase capillary permeability (25). This permeability results in intravascular hypovolemia, extravascular edema, and inadequate tissue perfusion. Although there is no specific treatment for increased capillary permeability, effective anti-infection strategies may help reduce leakage. In this study, two time points were selected for analysis: within 48 hours and after 96 hours following sepsis diagnosis. Considering the time required for antibiotics to reach steady-state therapeutic levels, insufficient drug concentrations or suboptimal therapeutic responses could indicate unresolved capillary permeability, continuing the risk of volume deficits over time. Premature initiation of deresuscitation in this context could exacerbate hypovolemia and further compromise tissue perfusion.
Interestingly, the study found no significant difference in peak lactate levels between the early and late groups, indicating that the outcomes may not be solely attributed to impaired tissue perfusion. However, lactate has several limitations as a marker, including its lack of specificity for hypoperfusion, delayed response to therapy, and limited utility in guiding fluid resuscitation (26). Therefore, the absence of differences in lactate levels should not be interpreted as evidence of consistent tissue perfusion between the groups. Other indicators such as capillary refill duration, peripheral perfusion index, and mottling score may detect impaired tissue perfusion earlier than lactate (27,28), but these parameters were not effectively captured in the database used for this study.
To date, research comparing the timing of deresuscitation remains limited. Findings from a study by Shen et al., which involved critically ill patients requiring vasoactive medication, indicated that patients who received diuretics within 48 hours of ICU admission had a significantly lower mortality rate (29). This contrasts with the findings of the current study, likely due to differences in patient populations. Shen et al.’s study included a significant proportion of patients with cardiac conditions—40–50% with heart disease and 30–40% with acute or chronic heart failure—who may benefit from early diuretic use. Conversely, the current study focused on patients with sepsis, a population that typically requires early fluid resuscitation. Various parameters are available for assessing fluid overload to guide deresuscitation (23). While time is only one factor contributing to fluid accumulation, its role should not be underestimated. Further studies are warranted to examine its impact.
The type of intervention is as critical as its timing in managing fluid overload. Loop diuretics are commonly used, yet their efficacy and safety remain subjects of debate. Mehta et al., in their study on patients with AKI, found a significant association between diuretic use and an increased risk of mortality or failure to recover renal function (30). Conversely, a multicenter study involving patients who were mechanically ventilated reported that diuretic use demonstrated a favorable safety profile for both cardiac and renal outcomes (31). Some research even indicates that diuretic use may reduce mortality in patients with AKI (32). However, other studies have indicated no survival benefit of loop diuretics in patients with AKI (33).
The current study found that early diuretic administration was associated with increased mortality, with no significant differences observed in the incidence of AKI or stage 3 AKI between early and late intervention groups. However, after PSM, there was a significant increase in the proportion of patients in AKI stage 3, suggesting more severe kidney injury. The effects of diuretics on mortality and renal function remain contentious, with factors such as circulatory stability, volume status, baseline renal function, direct effects of diuretics, and timing of renal support measures contributing to the debate (30,31,33).
Within this study, patients in the early diuretic administration group exhibited relatively stable circulatory status. Specifically, fewer patients in this group required vasoactive medications, and they received lower cumulative doses of norepinephrine within the first 48 hours compared to the late group. These observations may support the rationale for clinicians to consider using diuretics without compromising circulatory stability. Although patients in the early group used fewer vasopressors, it is important to note that their corresponding MAP was significantly lower than that of the late group. We also observed that the early group had a higher number of hypertensive patients, suggesting that even if the MAP is within the target range and there is little change in MAP before and after diuretic administration, effective perfusion pressure may already be impaired in patients with a long history of hypertension, which could potentially impact their prognosis. Moreover, the early group also demonstrated a more stable circulatory status and lower fluid intake, potentially reflecting differences in sepsis severity. Notably, the late group had higher overall SOFA scores, indicating a greater requirement for fluid resuscitation. After the early administration of diuretics, there was no significant difference in urine output between the two groups. This may be due to the fact that the early group’s condition was still unstable, leading to suboptimal diuretic response. Considering the intake volume, the overall fluid balance in the early group remained lower than that in the late group. While prior studies have associated lower fluid balance with enhanced outcomes (34,35), the findings of this study did not align with those conclusions. In terms of lactate levels, often used an indicator of tissue perfusion, there were no significant differences between the two groups, indicating that lactate may not be the primary determinant of patient outcomes in this context. Given the limitations of lactate as a biomarker—such as its low specificity for hypoperfusion and delayed responsiveness to therapeutic interventions—the need for alternative indicators of tissue perfusion remains critical.
Shen et al. highlighted that the severity of AKI could confound adverse outcomes, noting that diuretic use in patients with severe AKI may correlate with poorer prognoses (29). In the current study, while no significant difference in the incidence of AKI or stage 3 AKI was observed. After PSM, the proportion of patients with AKI stage 3 in the early group was higher than in the late group (8.1% vs. 5.7%), indicating more severe renal damage in the early group. Diuretics, by enhancing urine output, might influence clinical decisions on initiating blood purification therapies in patients with stage 3 AKI. Research has indicated that delays in starting blood purification have been linked to increased mortality (36).
In this study, the proportion of patients who started CRRT was higher in the early group. This could imply earlier initiation of blood purification in the early intervention group, though the timing of blood purification depends primarily on when AKI is diagnosed. Additionally, the underlying primary condition (sepsis, in this study) may also influence the outcomes, as its pathophysiological characteristics differ from those observed in patients with isolated kidney injury, mechanical ventilation, or shock, as seen in other studies. Therefore, the effects of the same therapeutic intervention, such as diuretics, may vary substantially across different patient populations.
The impact of diuretic dosage should not be overlooked. In this study, we found that increased diuretic dosage was associated with improved patient survival. This finding aligned with earlier evidence suggesting that early diuretic use may increase mortality. A higher diuretic dose may indicate more effective fluid management, particularly for patients with fluid overload, allowing for quicker edema reduction and improved organ function, ultimately leading to better outcomes. Additionally, the timing of diuretic administration is equally important. In the late-stage group, patients may have reached a more stable condition over time, making diuretic use more effective, with fewer side effects and greater clinical benefits.
Limitations
This study has several limitations. The clinical administration of diuretics is influenced by numerous confounding factors, including individual clinician preferences and practices. While efforts were made to account for as many confounders through PSM, this approach is not comprehensive. As a retrospective study, some data were inevitably unavailable.
Although this study examined differences in vasoactive drug dosage and changes in MAP, a significant proportion of patients had hypertension. The target perfusion pressure may vary among different patients, and even within the same patient, different organs may have varying tolerances to the same perfusion pressure. Therefore, the observed MAP values may not fully capture the overall condition of complex patients. Fluid input represents a critical variable in this context. The study incorporated fluid data collected within the first 48 hours following sepsis diagnosis. However, patient care is a continuous process, and patients may have received fluid resuscitation in the emergency department or other units before a definitive diagnosis was established. Quantifying this earlier fluid administration proves challenging, increasing the likelihood that total fluid volume has been underestimated. Additionally, this study did not analyze the specific dosages of diuretics administered, which may have influenced their effects on patient outcomes. Finally, while the results indicate an association between early diuretic use and increased mortality, this finding should be interpreted with caution. The data from this study cannot establish a definitive causal relationship between diuretic use and mortality rates, and further research is needed to validate these observations.
ConclusionsOther Section
Early diuretic administration in patients with sepsis warrants careful consideration, as it may be associated with kidney function impairment and an increased mortality rate. Further research, particularly large-scale randomized controlled trials, is needed to thoroughly assess the impact of diuretic timing on outcomes in patients with sepsis.
AcknowledgmentsOther Section
We would like to acknowledge the hard and dedicated work of all the staff that implemented the intervention and evaluation components of the study.
FootnoteOther Section
Reporting Checklist: The authors have completed the STROBE reporting checklist. Available at https://tau.amegroups.com/article/view/10.21037/tau-24-620/rc
Peer Review File: Available at https://tau.amegroups.com/article/view/10.21037/tau-24-620/prf
Funding: None.
Conflicts of Interest: All authors have completed the ICMJE uniform disclosure form (available at https://tau.amegroups.com/article/view/10.21037/tau-24-620/coif). The authors have no conflicts of interest to declare.
Ethical Statement: The authors are accountable for all aspects of the work in ensuring that questions related to the accuracy or integrity of any part of the work are appropriately investigated and resolved. The study was conducted in accordance with the Declaration of Helsinki (as revised in 2013).
Open Access Statement: This is an Open Access article distributed in accordance with the Creative Commons Attribution-NonCommercial-NoDerivs 4.0 International License (CC BY-NC-ND 4.0), which permits the non-commercial replication and distribution of the article with the strict proviso that no changes or edits are made and the original work is properly cited (including links to both the formal publication through the relevant DOI and the license). See: https://creativecommons.org/licenses/by-nc-nd/4.0/.
ReferencesOther Section
- Evans L, Rhodes A, Alhazzani W, et al. Surviving sepsis campaign: international guidelines for management of sepsis and septic shock 2021. Intensive Care Med 2021;47:1181-247. [Crossref] [PubMed]
- Rivers E, Nguyen B, Havstad S, et al. Early goal-directed therapy in the treatment of severe sepsis and septic shock. N Engl J Med 2001;345:1368-77. [Crossref] [PubMed]
- Malbrain MLNG, Van Regenmortel N, Saugel B, et al. Principles of fluid management and stewardship in septic shock: it is time to consider the four D's and the four phases of fluid therapy. Ann Intensive Care 2018;8:66. [Crossref] [PubMed]
- Hansen B. Fluid Overload. Front Vet Sci 2021;8:668688. [Crossref] [PubMed]
- Boyd JH, Forbes J, Nakada TA, et al. Fluid resuscitation in septic shock: a positive fluid balance and elevated central venous pressure are associated with increased mortality. Crit Care Med 2011;39:259-65. [Crossref] [PubMed]
- National Heart, Lung, and Blood Institute Acute Respiratory Distress Syndrome (ARDS) Clinical Trials Network; Wiedemann HP, Wheeler AP, et al. Comparison of two fluid-management strategies in acute lung injury. N Engl J Med 2006;354:2564-75.
- Messmer AS, Dill T, Müller M, et al. Active fluid de-resuscitation in critically ill patients with septic shock: A systematic review and meta-analysis. Eur J Intern Med 2023;109:89-96. [Crossref] [PubMed]
- Wichmann S, Barbateskovic M, Liang N, et al. Loop diuretics in adult intensive care patients with fluid overload: a systematic review of randomised clinical trials with meta-analysis and trial sequential analysis. Ann Intensive Care 2022;12:52. [Crossref] [PubMed]
- Jones TW, Smith SE, Van Tuyl JS, et al. Sepsis With Preexisting Heart Failure: Management of Confounding Clinical Features. J Intensive Care Med 2021;36:989-1012. [Crossref] [PubMed]
- de Louw EJ, Sun PO, Lee J, et al. Increased incidence of diuretic use in critically ill obese patients. J Crit Care 2015;30:619-23. [Crossref] [PubMed]
- Uchino S, Doig GS, Bellomo R, et al. Diuretics and mortality in acute renal failure. Crit Care Med 2004;32:1669-77. [Crossref] [PubMed]
- Singer M, Deutschman CS, Seymour CW, et al. The Third International Consensus Definitions for Sepsis and Septic Shock (Sepsis-3). JAMA 2016;315:801-10. [Crossref] [PubMed]
- ARISE Investigators. Goal-directed resuscitation for patients with early septic shock. N Engl J Med 2014;371:1496-506. [Crossref] [PubMed]
- ProCESS Investigators. A randomized trial of protocol-based care for early septic shock. N Engl J Med 2014;370:1683-93. [Crossref] [PubMed]
- Mouncey PR, Osborn TM, Power GS, et al. Trial of early, goal-directed resuscitation for septic shock. N Engl J Med 2015;372:1301-11. [Crossref] [PubMed]
- Rhodes A, Evans LE, Alhazzani W, et al. Surviving Sepsis Campaign: International Guidelines for Management of Sepsis and Septic Shock: 2016. Intensive Care Med 2017;43:304-77. [Crossref] [PubMed]
- Messmer AS, Zingg C, Müller M, et al. Fluid Overload and Mortality in Adult Critical Care Patients-A Systematic Review and Meta-Analysis of Observational Studies. Crit Care Med 2020;48:1862-70. [Crossref] [PubMed]
- Hjortrup PB, Haase N, Bundgaard H, et al. Restricting volumes of resuscitation fluid in adults with septic shock after initial management: the CLASSIC randomised, parallel-group, multicentre feasibility trial. Intensive Care Med 2016;42:1695-705. [Crossref] [PubMed]
- Silversides JA, Major E, Ferguson AJ, et al. Conservative fluid management or deresuscitation for patients with sepsis or acute respiratory distress syndrome following the resuscitation phase of critical illness: a systematic review and meta-analysis. Intensive Care Med 2017;43:155-70. [Crossref] [PubMed]
- National Heart, Lung, and Blood Institute Prevention and Early Treatment of Acute Lung Injury Clinical Trials Network; Shapiro NI, Douglas IS, et al. Early Restrictive or Liberal Fluid Management for Sepsis-Induced Hypotension. N Engl J Med 2023;388:499-510.
- Kjær MN, Meyhoff TS, Sivapalan P, et al. Long-term effects of restriction of intravenous fluid in adult ICU patients with septic shock. Intensive Care Med 2023;49:820-30. [Crossref] [PubMed]
- Meyhoff TS, Hjortrup PB, Wetterslev J, et al. Restriction of Intravenous Fluid in ICU Patients with Septic Shock. N Engl J Med 2022;386:2459-70. [Crossref] [PubMed]
- Malbrain MLNG, Martin G, Ostermann M. Everything you need to know about deresuscitation. Intensive Care Med 2022;48:1781-6. [Crossref] [PubMed]
- Boulain T, Cecconi M. Can one size fit all? The fine line between fluid overload and hypovolemia. Intensive Care Med 2015;41:544-6. [Crossref] [PubMed]
- Huang M, Cai S, Su J. The Pathogenesis of Sepsis and Potential Therapeutic Targets. Int J Mol Sci 2019;20:5376. [Crossref] [PubMed]
- Legrand M, van der Horst ICC, De Jong A. Serial lactate measurements to guide resuscitation: more evidence not to? Intensive Care Med 2024;50:728-30. [Crossref] [PubMed]
- Lima AP, Beelen P, Bakker J. Use of a peripheral perfusion index derived from the pulse oximetry signal as a noninvasive indicator of perfusion. Crit Care Med 2002;30:1210-3. [Crossref] [PubMed]
- Ait-Oufella H, Bakker J. Understanding clinical signs of poor tissue perfusion during septic shock. Intensive Care Med 2016;42:2070-2. [Crossref] [PubMed]
- Shen Y, Zhang W, Shen Y. Early diuretic use and mortality in critically ill patients with vasopressor support: a propensity score-matching analysis. Crit Care 2019;23:9. [Crossref] [PubMed]
- Mehta RL, Pascual MT, Soroko S, et al. Diuretics, mortality, and nonrecovery of renal function in acute renal failure. JAMA 2002;288:2547-53. [Crossref] [PubMed]
- Cinotti R, Lascarrou JB, Azais MA, et al. Diuretics decrease fluid balance in patients on invasive mechanical ventilation: the randomized-controlled single blind, IRIHS study. Crit Care 2021;25:98. [Crossref] [PubMed]
- Zhao GJ, Xu C, Ying JC, et al. Association between furosemide administration and outcomes in critically ill patients with acute kidney injury. Crit Care 2020;24:75. [Crossref] [PubMed]
- Sampath S, Moran JL, Graham PL, et al. The efficacy of loop diuretics in acute renal failure: assessment using Bayesian evidence synthesis techniques. Crit Care Med 2007;35:2516-24. [Crossref] [PubMed]
- Shahn Z, Shapiro NI, Tyler PD, et al. Fluid-limiting treatment strategies among sepsis patients in the ICU: a retrospective causal analysis. Crit Care 2020;24:62. [Crossref] [PubMed]
- Finfer S, Myburgh J, Bellomo R. Intravenous fluid therapy in critically ill adults. Nat Rev Nephrol 2018;14:541-57. [Crossref] [PubMed]
- Gaudry S, Hajage D, Martin-Lefevre L, et al. Comparison of two delayed strategies for renal replacement therapy initiation for severe acute kidney injury (AKIKI 2): a multicentre, open-label, randomised, controlled trial. Lancet 2021;397:1293-300. [Crossref] [PubMed]