Future perspectives of prostate cancer therapy
Introduction
Prostate cancer is the second most common cause of cancer-related death in American men. Along with surgery or radiation therapy, hormonal therapy is a main mode of treatment. For men with metastatic disease, chemotherapy provides a significant survival advantage. Therefore, new treatment options are being actively pursued to extend the survival of metastatic cancer patients. In this review, we will focus on current advances in therapies that target cancer cells, outline recent advances in our understanding of the tumor microenvironment and its therapeutic implications for advanced metastatic prostate cancer patients and discuss the current therapeutic modalities, highlight new treatment options and offer future perspectives on prostate cancer therapy. We will discuss therapies that target: (I) cancer cells; (II) stromal fibroblasts; (III) vascular endothelial cells; (IV) immune cells and (V) less well-defined population of cells that contribute to the effectiveness of immunotherapy and cancer vaccines.
Targeting prostate cancer cells
Hormonal agents for prostate cancer therapy
Androgen and androgen receptor (AR) are required for normal prostate development and carcinogenesis. Castration-resistant prostate cancer tissues (CRPC) express AR and remain responsive to low levels of androgens. AR mutation, truncation and/or amplification may confer differential ligand and antagonist affinity and specificity. Thus, even low levels of testosterone could still activate the AR and confer the growth and survival advantages for prostate cancer cells. Several studies have demonstrated that low levels of testosterone are present in prostate cancer cells. Mohler et al. studied testosterone levels in clinical specimens collected from castrated patients who underwent prostatectomy and found that intratumoral testosterone levels were elevated despite an overall reduction in serum testosterone (1). Intracellular androgen in prostate cancer tissues has demonstrated clinical significance as treatment with agents that reduce their levels have impacted overall survival for men with castrate-resistant disease. Abiraterone (Zytiga, Janssen Biotech, Horsham, PA) is a selective 17,20 lyase inhibitor, which inhibits the conversion of 17-α-hydroxyprogesterone to androstenedione. This agent was brought to a randomized phase III clinical trial against placebo in men with castrate-resistant disease who had received prior docetaxel. In this study, treatment with abiraterone was associated with a 35% reduction in death from prostate cancer with an improvement in median survival from 10.9 to 14.8 months (2). The survival benefit was observed across all subgroups analyzed, including number of prior chemotherapeutic regimens (one or two), type of progression (PSA versus radiographic), and patients with visceral metastatic diseases. Orteronel (TAK-700, Millennium Pharmaceuticals, Cambridge, MA) is another compound targeting 17,20 lyase and endogenous testosterone biosynthesis. In a phase I/II study of orteronel, of 43 patients with RECIST-evaluable disease, 6 showed a partial response, 23 had stable disease, and 9 showed progression (3). Phase III clinical trials with Orteronel are currently in progress in chemotherapy naïve and post-docetaxel settings. They will evaluate tumor response rate and survival benefit attributed to Orteronel therapy. Unlike abiraterone, it may be possible to administer orteronel without the use of concominant prednisone given the higher specific inhibition of this agent against CYP17.
MDV3100 (Medivation, Inc., San Francisco, CA) is an orally bioavailable anti-androgen lacking the agonist properties of conventional non-steroidal antiandrogens such as bicalutamide (4). MDV3100 antagonizes AR action by preventing the translocation of the AR from cytoplasmic to nuclear compartment and by inhibiting DNA binding of AR and hence repressed the expression of androgen-regulated genes. In a phase I study of docetaxel-naïve and docetaxel-treated patients, 62% and 51% of patients, respectively, had at least a 50% PSA decline (5). Phase III randomized trials have been completed evaluating MDV3100 in both the pre- and post-docetaxel clinical spaces (1,3,6-10). The results from the completed post-chemotherapy studies (AFFIRM) will be presented in February 2012.
Novel cytotoxic chemotherapeutics to treat prostate cancer
Cabazitaxel (Jevtana, XRP6258, RPR 116258A; Sanofi-Aventis, Paris, France) is a semisynthetic taxane that has been shown to have activity against multidrug-resistant prostate cancer cell lines in vitro (11). This preclinical observation led to a randomized trial in patients with CRPC who failed docetaxel-based chemotherapy. Patients were eligible for study if they had PSA progression, or with soft tissue and/or new lesions on bone scan. In this phase III trial, 720 patients were randomly assigned to receive either cabazitaxel, or mitoxantrone, every 3 weeks. The median survival for patients treated with cabazitaxel was 15.1 months, compared to 12.7 months in those patients treated with mitoxantrone with an overall 30 reduction in death from prostate cancer (12). In order to compare the efficacy of cabazitaxel/prednisone as first-line chemotherapy to the current therapeutic regimen, docetaxel/prednisone, an international randomized study is currently being designed at the mandate of the US Food and Drug Administration (12).
Antiapoptotic agents in prostate cancer
One unique feature of the androgen-independent prostate cancer cells is that the regression of prostate tumors still required an activation of apoptotic machinery. In many cases, AR blocking is capable of inducing apoptosis. Therefore, identifying a cure for prostate cancer requires identification and reversal of the apoptotic avoidance mechanisms, either AR-related or unrelated, responsible for drug resistance and/or newer therapies that bypass the apoptosis-resistance pathways. A number of antisense oligonucleotides targeting several anti-apoptotic genes, including BCL-2, BCL-XL, clusterin, the inhibitors of apoptosis (IAP) family, MDM2, protein kinase C-alpha, c-raf, insulin-like growth factor binding proteins and the AR, are being tested for potential clinical use in prostate cancer. Clusterin is a proapoptotic protein expressed in prosate, kidney, bladder, ovarian, lung, colorectal, and breast cancers. Clusterin expression increases with Gleason score, and is upregulated after androgen blockade (13,14). Clusterin modulates resistance to androgen blockade, radiation therapy, and chemotherapy. OGX-011 (Custirsen) is an investigational antisense compound that downregulates clusterin expression and enhances apoptotic death of prostate cancer cells (15). Increased apoptotic index of prostate cancer cells have been reported subsequent to clusterin inhibition. OGX-011/docetaxel/prednisone has been evaluated in combination with docetaxel/prednisone in men with CRPC (16). Although there was no difference was observed in time to disease progression (7.3 vs. 6.1 months), a superior survival was noted with OGX-011 (23.8 vs. 16.9 months) (7,17). In a randomized trial of Custirsen with docetaxel or mitoxantrone in patients who have progressed through docetaxel chemotherapy, the addtition of Custirsen was well tolerated and appeared to improve pain response. In the population of men who had previous docetaxel, the addition of OGX-011 yielded 23% partial radiographic responses by RECIST criteria and PSA declines in excess of 30% in 55% of the treatment arm (17).
Targeting tumor stromal fibroblastic microenvironments in prostate cancer
Tumor-stroma interactions are crucial for normal prostate development and neoplastic prostate progression. It has been demonstrated that fibromuscular stroma and stromal fibroblasts play a regulatory role in prostate development and prostate carcinogenesis. In these studies, urogenital sinus mesenchyme (UGM) or embryonic/adult stromal fibroblasts were shown to drive the growth of UG epithelium (UGE) and prostate cancer (18-22). Using a tissue recombination technique, it has been demonstrated that while UGM derived from AR-negative testicular feminized mice failed to induce prostate morphogenesis and cytodifferentiation, UGM isolated from AR-positive wild-type mice is competent in conferring growth and differentiation signals to UGE tissues by responding to androgen-regulated growth (23-28) and expression of differentiation-related genes regardless of their AR status. Results of these studies in aggregate suggest that AR signaling from the stroma is critical for the development and differentiation of the normal prostate epithelium (19,21). The inductive role of adult prostate stromal fibroblasts, promoting prostate cancer progression, was first demonstrated by our laboratory using cell recombination models (19,20,23-28). Specifically, the progression of prostate cancer from androgen-dependent to androgen-independent state and the acquisition of bone and soft tissue metastatic phenotypes can be achieved through cellular interactions between prostate cancer cells and organ-specific stromal fibroblasts including prostate or bone stromal cells in mice in vivo or when co-cultured these interactive cells under three-dimensional (3D) conditions (29-34). These findings, taken together, emphasized the important role of the stromal and tumor microenvironment in prostate cancer progression and hence the rationales for co-targeting tumor and stroma (20,22,34,35).
Stromal cells surrounding the cancer cells, including stromal fibroblasts, endothelial cells, and inflammatory cells in the primary and bone cells at the metastatic sites have been shown to exert directive action on prostate cancer cells by modulating reciprocally cancer cell growth, migration, invasion and metastasis. Impairment of reciprocal stromal or bone cell function and their communication with cancer cells could significantly impact the growth and progression of prostate cancer within the tumor microenvironments. Table 1 summarizes several co-targeting strategies of cancer-associated stroma, either in the primary tumor or in bone metastases that have been implemented in the clinic for improving the mortality and morbidity of prostate cancer patients. Future research on the specific mediators and cell signaling pathways regulating the reciprocal cellular communication between cancer cells and their immediate microenvironments and circulating factors in cancer and microenvironment cell milieu could further significantly improve our ability to target the progression of cancer and its lethal metastatic progression. For example, it has been established that immortalized stromal fibroblasts or cancer-associated fibroblasts (CAF) adjacent to tumors are morphologically and functionally distinct from normal stromal fibroblasts adjacent to normal epithelium (18,31). These cells exhibit marked differences in gene expression profiles and have been shown to predict the progression of prostate cancer (66). We demonstrated the reciprocal cellular interaction between prostate cancer and CAF or stromal fibroblasts from different zonal origin (31). Using marginally androgen responsive tumorigenic LNCaP human prostate cancer cells, we demonstrated that co-culture of the cancer cells with microcarrier beads previously seeded with prostate or bone stromal cells of the human prostate gland or human bone, under 3D culture system, led to permanent nonrandom genetic and phenotypic changes in both the cancer and the stroma. LNCaP cells derived from these growth conditions became androgen-independent and gained the ability to metastasize. Stromal fibroblasts that interact with cancer cells, also gained increased levels of brain derived neurotropic factor (BDNF), chemokines (e.g., CCL5 and CXCL5), versican, tenascin, stromal cell derived factor-1 (SDF-1/CXCL12), and transcription factors like HIF-1α. These were validated using clinical tissue or serum samples obtained from prostate cancer patients with bone metastases. Studies from our group and others have demonstrated the role of stromal soluble factors such as VEGF, bFGF, HGF/SF, TGF-β, IGF-1, IL-6 and KGF, interacting with receptors on prostate cancer cells (refs). These studies highlight the bidirectional interactions and co-evolution of tumor–stroma in cancer progression (67). Therapies that target many of the stromal factors have been tested in preclinical models and in clinical trials to eradicate or delay the lethal progression of prostate cancer and other solid tumors to the metastatic phenotype.

Full table
Targeting angiogenesis in prostate cancer
Angiogenesis is essential for the growth and dissemination of prostate cancer cells. The process of blood vessel formation is regulated by complex interactions of vascular growth factors, including VEGF, matrix metalloproteins, and integrins. Inhibition of these proteins that support angiogenesis can block tumor growth as well as inhibit metastasis. Several studies demonstrate that circulating levels of VEGF were increased in patients with CRPC and serve as prognostic markers for patient survival (68). Microvessel density has been found to be increased in patients who have metastatic disease in comparison to those who have clinically localized cancer (36,37). Thus, the tumor vasculature appears to be a rational therapeutic target for men with prostate cancer. Significant work has been undertaken evaluating putative antiangiogenic agents. Early work with thalidomide showed activity as a single agent (38). This work has developed into a series of clinical studies supported by the intramural program of the National Cancer Institute including recent work with a combination of docetaxel, bevacizumab, and thalidomide (39). Bevacizumab, an antibody which blocks the biding of VEGF-A to the VEGF-R, is approved for use in non-small-cell lung and colorectal cancer (69). Other potent anti-angiogenic agents such as sorafenib (Nexavar), sunitinib (Sutent) (70), and aflibercept (VEGF Trap) (40) have shown the potential for benefit in this disease that is still under evaluation.
Targeting tyrosine kinases in prostate cancer
The efficacy of receptor tyrosine kinase inhibitors such as sunitinib or sorafenib has been disappointing in clinical trials for prostate cancer. Unlike other therapies, these agents have been associated with prolonged progression-free survival but no potent anti-tumor effect. A receptor tyrosine kinase inhibitor has a unique clinical phenotype that may potentially translate to therapeutic benefit. Cabozantinib (XL184, Exelixis, San Francisco, CA), is an orally available, multiple tyrosine kinases inhibitor. It inhibits activation of the c-MET protooncogene, as well as VEGFR2. In preclinical animal and cell models, cabozantinib exhibited potent dose-dependent cancer growth inhibition and tumor regression against a variety of solid tumors (41,42). Studies with prostate cancer specimens derived from primary tumors as well as bone, lymph node, and soft tissue metastases reveal that 51% of primary prostate cancer tissues expressed c-MET. In particular, osseous metastates from prostate cancers have been found to express significantly more c-MET than even soft tissue specimens (41,42). A 9-arm randomized discontinuation trial of cabozantinib which included patients with metastatic CRPC has been reported (43). In the CRPC arm, of the 168 patients enrolled, 100 were evaluable for response by RECIST (Response Evaluation Criteria in Solid Tumors). Fifty-five of the 65 (85%) patients with serial bone scans showed complete or partial resolution of lesion as early as 6 weeks after starting therapy. Cabozantinib continues to be evaluated in prostate cancer as Exelixis has planned two phase III studies with this agent in prostate cancer that should begin in 2012- one evaluating pain response, the other evaluating the survival benefit associated with this agent.
Bone-directed targeting for treating prostate cancer bone metastasis
The endothelins (ET-1, ET-2, and ET-3), consisting of 21 amino acids, are expressed by endothelial cells; kidney and intestine; and brain, respectively. This class of peptides is known to control vasoconstriction, mitogenesis, and bone matrix formation with their actions mediated by ET receptors, ETA and ETB. The endothelin receptors are expressed in a variety of human tumors, including prostate cancer and osteoblasts. Interaction of endothelins with their receptors results in enhanced cell proliferation, bone matrix synthesis and deposition, and resistance to apoptosis in prostate cancer (41,44). Atrasentan, a specific ET-1A inhibitor, exhibits anti-mitogenic activity, anti-osteoblastic activity, decreases rates of bone metastases, anti-angiogenesis activity, and blocks nociceptive effects.
Dasatinib, a tyrosine kinase inhibitor that inhibits the Src-family kinases (SFKs) has been studied in CRPC. SFKs are known to play an important role in bone resorption (71) and appear to be upregulated in advanced prostate cancer (45). Work by our group and others have pointed toward SFKs as regulators of metastatic behavior (46). A phase II study in metastatic CRPC demonstrated that 41% of patients have greater than 50% PSA decline with 35% reduction in bone turnover in 46% of patients (47). Bone alkaline phosphatase levels also were decreased in dasatinib-treated patients. Docetaxel was combined safely with conventional docetaxel therapy (48) showing again, potent effects on bone turnover. Based upon the preliminary data, a randomized phase III trial comparing docetaxel/prednisone in 1500 patients with CRPC, either with or without dasatinib was executed. Results from this study are pending and may be available in 2012.
The receptor activator of nuclear factor-κB (RANK)/RANKL axis has been shown to play a critical role in maintenance of osteoclast and osteoblast function. Given the imbalance of activities between these cell populations in prostate cancer, RANKL has been considered an attractive target for therapy. This has been borne out in preclinical models of prostate cancer metastasis (49). Denosumab (Xgeva, Prolia; Amgen, Thousand Oaks, CA) is a fully humanized monoclonal antibody that targets RANKL. The FDA initially gave approval for this agent in 2010 for the treatment of osteoporosis related to menopause. Subsequently, denosumab received approval for the treatment of skeletal related events in prostate cancer (50) and other solid tumors (51) and osteoporosis due to hormonal anti-cancer therapies in breast (52) and prostate cancer. Denosumab, targets RANKL axis was shown to delay the onset of bone metastasis and skeletal related events including the relief in the bone pain in men with CRPC who develop bone metastasis. Given the putative impact of RANKL on progression to osseous metastasis, a phase III trial of denosumab was initiated to test the hypothesis that treatment with denosumab would delay the onset of bone metastases in patients who were currently metastasis free (72). This study focused on a population of men at high risk for osseous metastasis (CRPC with serum PSA >8.0 ng/mL and/or PSA doubling time of <10 months). Treatment with denosumab was associated with a 15% reduction in the risk of bone metastasis with a median time to metastasis of 29.5 vs. 33.2 months in favor of denosumab.
Radioisotopes, such as strontium-89 (Metastron) and samarium-153-EDTMP (Quadramet), are approved for the palliation of bone pain in men with CRPC (73,74). Radium-223 chloride (Alpharadin; Algeta) is a selective α-emitter that has been evaluated in patients with CRPC. In contrast to the approved isotopes mentioned, improved survival was noted in patients treated with radium-223 when compared to placebo (65.3 vs. 46.4 weeks) in a phase II trial (53). As such a formal phase III study (ALSYMPCA) was initiated comparing radium-223 to placebo in men with bone metastases who had previously received docetaxel or were ineligible for docetaxel therapy (54). This trial was closed early by the independent data monitoring committee as criteria for a significant treatment benefit were reached. Treatment with radium-223 was associated with a 30% decrease in prostate cancer related death compared to placebo with median survivals of 14.0 vs. 11.2 months in favor of radium-223. Algeta has moved forward with their new drug application with hopes of approval in 2012.
Molecular therapeutics to co-target prostate cancer and cancer-associated bone cells
Cancer-host interactions play a fundamental role in directing cancer plasticity, progression, responsiveness, and resistance to treatments such as hormone therapy, chemotherapy and radiation therapy. We recommend that future development of novel therapies should focus on the cancer-host interactions. This may improve treatment efficacy since the tumor-associated microenvironment may be protective to cancer cells, preventing the regression or apoptosis of treated tumors. Targeting only the cancer cells may not be sufficient since cancer cells and their associated stroma co-evolve. The field of tumor-stroma biology has expanded our understanding of cancer as more than a single cell disease. Rather, cancer development and progression involves reciprocal interaction and co-evolution between cancer cells and host stroma with reactive oxygen species, soluble growth factors, chemokines, cytokines and extracellular matrices serving as the key mediators. We and others have shown that cancer cells and their associated stroma are remarkably plastic and capable of expressing genes mimicking the tumor microenvironment. These new understandings of cancer-stroma interaction raise the possibility of co-targeting not only the cancer cell component but also cancer-associated stroma, and blocking not only autocrine but also paracrine cell signaling. Further expansion of our understanding of tumor-stroma biology could lead to the successful development of more effective animal models to study the mechanisms of prostate cancer metastases. This will be a novel step toward the discovery of more effective therapeutic interventions for prostate cancer metastases through the interruption of cancer-stromal fibroblasts, cancer–bone, cancer-endothelium, cancer-stem cell, cancer-nervous system and cancer-immune system communications (55,66,67).
Targeting the epithelial to mesenchymal transition (EMT) in prostate cancer
EMT is a highly conserved process where polarized immotile epithelial cells transition to motile mesenchymal cells. EMT is commonly associated with cancer migration, invasion and metastasis. The common feature of EMT is the loss of E-cadherin and an increased expression of vimentin and N-cadherin. In cancer, EMT could facilitate cancer aggressive behavior by infiltrating surrounding tissues and metastasize to soft tissues and bone. EMT can be enhanced by the augmentation of specific growth factor/growth factor signaling and hence can be targeted by growth factor receptor signaling or at the level of downstream cadherin-switch such as antibody against N-cadherin to prevent the switch between E-cadherin to N-cadherin (75). In prostate cancer, EMT has been described as a notable feature of the androgen-independent prostate cancer (ARCaPE/ARCaPM, C4-2/C4-2B, and PC-3) cell models and was confirmed in clinical specimens and circulating tumor cells (CTCs) harvested from patients (32,56,66,76,77). RANKL is a potent paracrine factor for osteoclastogenesis and bone resorption. Under physiologic conditions, RANKL, expressed by osteoblasts, stimulates osteoclast maturation and bone resorption through the surface RANK receptor-expressing osteoclasts. We previously demonstrated that mesenchymal metastatic human prostate cancer cells (ARCaPM cells) express higher levels of functional RANKL, capable of promoting osteoclast maturation (78). Interestingly, RANKL-derived from cancer cells can also promote the transition of ARCaPE cells, with an epithelial phenotype, to express a mesenchymal phenotype, like those of ARCaPM cells, thus suggesting autocrine function of RANKL in the induction of EMT. In experimental human xenograft and cell models, RANKL is a biomarker associated with EMT (78). Since RANKL is also expressed by the cells in the tumor microenvironments, such as osteoblasts; B- and T-cells, we observed that RANKL can also promote MET in androgen-sensitive LNCaP cells in both autocrine and paracrine manner, and drive their bone and soft tissue metastases through an activation of downstream c-MET signaling. We confirmed that this action of RANKL in promoting EMT and downstream c-MET is highly relevant in both experimental and human prostate cancer towards their development of CRPC phenotype (79) (and Chu et al., poster presentation at the Cancer-induced bone disease meeting, November 30- December 3, 2011, Chicago, IL, USA). In addition to RANKL, prostate cancer cell lines and clinical samples are shown to secrete soluble factors such as β2-microglobulin (β2-M). This protein is not only responsible for driving EMT and bone metastasis of human prostate cancer cells but also in human breast, renal and lung cancer cells. The resulting ARCaPM cells had high levels of the mesenchymal markers such as vimentin, N-cadherin and Snail and exhibit 100% incidence of bone metastasis in an intracardiac injection model. β2-M interacts with its receptor, hemochromatosis (HFE) protein, to modulate iron responsive pathways in cancer cells. Inhibition of either β2-M or HFE results in reversion of EMT (56,80,81). These results demonstrate the role of β2-M in cancer metastasis and lethality. Thus, β2-M and its downstream signaling pathways are promising prognostic markers of cancer metastases and novel therapeutic targets for cancer therapy. Preclinical studies in both immune-compromised and immune-intact mouse models of prostate cancer revealed anti-β2-M monoclonal antibody significantly reduced tumor burden of primary tumors and bone metastasis ((56) and unpublished data). Currently, humanization of anti-β2-M is underway with the goal of initiating phase I clinical trials in prostate cancer patients with bone metastases. As such we propose that the addition to β2-M-targeted therapy to RANKL inhibition may be an effective way to treat skeletal metastasis in human prostate cancer.
Targeting immune microenvironment of prostate cancer
Impairment of immune cell function in the cancer microenvironment is believed to be an important step in tumor progression. It is hypothesized that co-targeting of immune cells in addition to cancer cells will lead to better killing of cancer cells. Recent studies highlight the tumor-promoting role of myeloid and lymphoid cells in the progression of solid tumors, linking inflammation and cancer (82-91). Though studies from the last century reported that mononuclear cells infiltrate solid tumors, it took several years to establish that such cells are causally involved in tumor progression. This became possible due to the discovery, phenotypic and functional characterization of a variety of subsets of T cells, B cells, macrophages and dendritic cells facilitated by discovery of novel markers and use of cutting edge technologies including flow cytometry.
Most of the human solid cancers develop in immune intact human beings. The progression of tumors from low-grade, localized disease to metastasis involves an interaction between the tumor cells and the host immune system. Most of our studies performed with human prostate cancer cell lines in laboratories use immune-deficient athymic nude mice (which lack T cells), SCID mice (lacking B and T cells) or NOD-SCID mice (lacking B, T and NK cells). These immune-deficient mice have allowed human prostate cancer xenografts to grow, greatly facilitating pre-clinical studies of targeted cancer therapies. Given the recent evidence that a vast majority of solid tumors are infiltrated by immune cells that facilitate tumor growth (rather than suppressing the tumor growth), it is imperative to understand the biology of these immune cells in the context of the tumor microenvironment.
Role of T lymphocytes in prostate cancer
Evidence supports a close link between inflammation and prostate cancer have come from epidemiological studies which indicate that prostate cancer is more common in populations with more baseline inflammation (92). Both CD4+ and CD8+ T cells are present in prostate glands. CD4+ T cells include both T helper 17 (TH17) and regulatory T (TReg) cell populations. Prostate-infiltrating CD8+ T cells in humans are non-functional and do not upregulate activation markers. T cells surrounding cancer cells may upregulate negative inhibitory molecules that suppress their anti-tumor activity (93). T cells may become anergic or undergo apoptosis due to reactive oxygen species generated by cancer cells. Though T cells surround prostate cancer, increasing evidence suggests that either they exhibit suppressive properties (Tregs) or they become non-functional (CD8+ T cell), thus allowing prostate cancer to grow. Overall, research from human and mouse models supports a model where evolving tumors generate T cells with an anti-cancer potential but, in the absence of some intervention, such T cells exist in a non-functional or anergic state (86,94,95).
Role of macrophages in prostate cancer
Tumor associated macrophages (TAM) influence diverse processes such as angiogenesis, tumor cell proliferation, and metastasis during tumor progression and thus play a pro-tumorigenic role (96). TAMs have been shown to play a key role in tumor growth and spread. Macrophages secrete growth factors, including platelet-derived growth factor (PDGF), epidermal growth factor (EGF) and transforming growth factor (TGF)-beta, as well as cytokines such as TNF-alpha and IL-1 that have been shown to promote metastatic spread in several animal models of tumors. In a variety of tumor types including prostate cancer, the amount of TAM has been associated with poor prognosis (97). One of the mechanisms involved in TAM-enhancement of cancer cell invasion involves a paracrine loop in which epidermal growth factor (EGF) produced by TAMs increases the invasiveness and migration of neighbouring breast cancer cells that express the EGF receptor (EGFR). Cancer cells in turn express CSF1, which acts as a potent chemoattractant and chemokine for CSF1R-expressing TAMs. This reciprocal cross-talk can be blocked by either EGFR or CSF1R antagonists, resulting in a decrease in migration and invasion of both cancer cells and macrophages (98).
Myeloid-derived suppressor cells
Another bone marrow-derived myeloid cell type (BMDC), which may share a common progenitor with TAMs, is the Myeloid-derived suppressor cells (MDSCs). MDSCs suppress the adaptive immune response by blocking the functions of CD4+ and CD8+ T cells, in part through arginase and nitric oxide production, by expanding the regulatory T cell pool, and by inhibiting NK cell activation (99). MDSC levels are increased in the bone marrow, blood and spleen of cancer patients and tumor-bearing mice, and their accumulation is associated with tumor growth and malignant progression. Disruption of transforming growth factor-β (TGF-β) signalling, through Tgfbr2 deletion, was also shown to increase MDSC homing to tumors in a spontaneous mammary cancer model, an effect that was mediated through the SDF1-CXCR4 and CXCL5-CXCR2 (also known as IL8RB) chemokine axes (100-102). In prostate cancer, MDSCs are recruited to the bone and at the primary sites (103). Clinical trials are being planned to target CXCL12 chemokine in CRPC (57). Another cytokine secreted by prostate cancer cells which recruit myeloid suppressor cells include CCL2 against which blocking antibodies are being tested for its therapeutic utility in solid tumors (58,59).
Mesenchymal stem cells
Another cell type that resides predominantly in the bone marrow, although is not of haematopoietic origin, is the mesenchymal stem cells (MSCs). MSCs are multipotent cells that differentiate into osteoblasts, chondrocytes and adipocytes. MSCs are found in large numbers in primary tumors and, MSCs have been proposed as a cellular vehicle to deliver anti-cancer drugs into the tumor.
Immunotherapy for prostate cancer: Current success and future challenges
Recently, the FDA approved the first immunotherapy-based approach (sipulucel-T, Provenge; Dendreon Inc) for treat patients with asymptomatic metastatic prostate cancer (60-62). In general, immunotherapy approaches seek to utilize the host immune cells to attack the underlying cancer. Despite a long history of negative clinical trials, in a definitive trial powered for overall survival, sipulecel-T was associated with a 23% decrease in prostate cancer mortality despite the absence of alternation of progression free survival. This finding has created significant interest in this advancing area of cancer research. Due to a variety of mechanisms by which cancer cells evade immune surveillance, cancer therapy has for years centered on chemotherapy. These toxic chemicals are designed to be more lethal to the rapidly dividing cancer cells than on normal tissue. Unfortunately, normal cells often are killed along with the malignant cells. Professor Ralph Steinman of Rockefeller University, a leading mind in cancer immunotherapy, identified the dentritic cell- a unique and important part of the immune cascade. For this Prof. Steinman was posthumously awarded the 2011 Nobel Prize in Medicine (63,104-107). The dendritic cell is one of the initial workhorses or sentinels of the immune system, processing foreign materials such as viruses and then presenting them to cytotoxic T cells that are activated in turn to attack the foreign antigens. Dr. Steinman isolated his dendritic cells, exposed them to his pancreatic cancer cells, and thus instructed his T cells to recognize those tumor antigens. The prognosis for the type of pancreatic cancer Dr. Steinman is only 4 months but Dr. Steinman survived for more than 4 years since he was first diagnosed with the disease (107). This immunotherapy regimen while not curative may have prolonged his life.
Prostate cancer immunotherapy seems promising and extends the mean survival of metastatic patients by an average of 4 months. Sipuleucel-T (Provenge), the first immunotherapeutic agent approved for the treatment of CRPC, is a dendritic-cell vaccine that is produced ex vivo from dendritic cells harvested from the patient in the clinic, which then are transported to a local GLP facility where the dendritic cells are loaded with a recombinant granulocyte macrophage colony-stimulating factor/prostatic acid-phosphatase fusion protein (61). These in vitro activated cells are reinfused into a patient. Side effects are modest, including fatigue, fevers, and chills at the time of infusion. Three randomized phase III trials comparing sipuleucel-T to placebo have been performed in patients with metastatic CRPC. In all three studies, those patients who were randomized to the placebo arm received a frozen dendritic-cell product at progression. Although the primary end point of progression-free survival was not met in either of the first two randomized trials, a survival benefit of 3-4 months was observed. The third randomized trial evaluating sipuleucel-T, involved patients (n=512) randomly assigned on a 2:1 basis to receive sipuleucel-T or placebo. A median survival benefit of 4 months was observed in favor of the patients receiving sipuleucel-T. At 3 years after study entry, 32% of patients treated with sipuleucel-T are alive compared to 23% of patients treated with placebo (60,62,64,108).
Vaccine based therapies are being currently under trials in CRPC. Two randomized trials using the allogeneic vaccine G-VAX viral vectors have failed to demonstrate a survival benefit (60,64,65,108). These viral vectors can mimic natural infection and, thus, boost the immune response. The viral vectors of poxvirus family have been used to deliver tumor (PSA) antigens as well as other immunomodulatory factors. A clinical trial was performed in 125 asymptomatic minimal CRPC patients who received either PROSTVAC-VF (Bavarian Nordic, Kvistgaard, Denmark) or control viral vectors. Although progression-free survival was similar in both groups, patients treated with PROSTVAC-VF had an 8.5-month improvement in median survival (24.1 vs. 16.6 months in control patients) (109). A randomized phase III trial is underway evaluating the role of this vaccine in asymptomatic CRPC (64).
The clinical trials of sipuleucel-T demonstrated a statistically significant and clinically meaningful improvement in overall survival in patients with mCRPC (64). However, none of these studies showed a concomitant improvement in progression-free survival. When traditional cytotoxic therapies are evaluated, progression-free survival is considered as a critical endpoint to assess the efficacy of therapy. This apparent disconnect between progression-free survival and overall survival while comparing immunotherapy versus conventional therapy can be explained in many ways. Unlike chemotherapy drugs, the primary target of immunotherapy based drugs is not the tumor itself but the immune system which targets the tumor. It may take few weeks to few months to mount a clinically significant immune response following immunotherapy. However, a vaccine or immunotherapy induces what is called long-lived memory cells which persist in the human body in the lymphoid tissues for years with the potential to continuously generate cytotoxic T cells to act against tumors, resulting in a slowing of the tumor growth. This process is well documented in vaccines that target infectious diseases. For example, vaccines against pox viruses confer lifelong immunity due to persistence of memory B cells and memory T cells. In a tumor, there is a turnover rate of tumor cells which is influenced by tumor cell division, antitumor immune response, combined with factors introduced into the tumor environment (e.g., conventional therapies). An effective anti-tumor immune response may alter the tumor growth equilibrium so that more tumor cells are killed by the immune system. This effect takes time and may not translate into immediate goals of short-term (within 3-4 months) improvements in progression-free survival, but may be long-lasting and overall survival may ultimately follow. Additional approaches to measure intermediate endpoints are the need of the hour to measure the efficacy of immunotherapy-based drugs.
Checkpoint inhibitors
Cytotoxic T-lymphocyte antigen 4 (CTLA4) is a cell-surface receptor expressed on the surface of helper T cells and interaction of CTLA-4 with its ligand downregulate T-cell responses. Ipilimumab is a fully human monoclonal antibody whichattenuates negative signals provided to T cells through the cell surface molecule CTLA-4, thereby blocking a negative checkpoint. Blocking the negative checkpoint leads to activation of T cells which would then kill cancer cells. This antibody has been evaluated in patients with metastatic melanoma and demonstrated an improvement in survival of 4 months. Ipilimumab may be very effective in CRPC. In contrast to vaccine or dendritic-cell based therapy, decline in PSA levels have been observed with this antibody therapy (110). Recent phase III clinical trial data demonstrate that ipilimumab prolongs survival in patients with melanoma, and 2 phase III overall survival trials are investigating the activity of ipilimumab in patients with mCRPC (111-114). The first trial combines a single 8 Gy dose of radiation with either ipilimumab or placebo in the post-docetaxel space, and the second evaluates the activity of ipilimumab versus placebo in chemotherapy-naïve patients.
Combination therapies
The standard of care for men with mCRPC includes docetaxel and prednisone. It is time we consider combinations of chemotherapy and immunotherapy as well. Frequent administration of doses of docetaxel in combination with immunotherapy may be a rational approach. However, studies that combines vaccine with higher doses of docetaxel (chemotherapy) and prednisone (anti-inflammatory drug) leads to immunosuppression wherein immune-cells are depleted by this approach. One way to overcome this problem might be to administer immunotherapy first followed by chemotherapy to avoid the immunosuppressive effects of chemotherapy. This approach will also facilitate a proinflammatory microenvironment in which tumor-cell killing by chemotherapy can be boosted by cytotoxic T cell mediated tumor killing. Although overall survival has been the only endpoint to demonstrate clinical benefit in clinical trials of vaccine in prostate cancer, it is possible that combination studies of therapeutic vaccines with other modalities may lead to earlier discriminatory endpoints, such as time to progression or PSA response, which could accelerate clinical trials for improved personalized oncology. It is imperative to consider cancer vaccines or immunotherapeutic approaches at the earlier stages of disease in prostate cancer and it can also be considered as an ideal adjuvant therapy post-surgery or -radiation in which presumably the bulk of the tumors have been removed and a smaller cluster of tumor cells may reside at the metastatic niche.
Summary and Conclusions
We have summarized the current approved treatments, ongoing clinical trials and preclinical studies in Table 1 and Figure 1. Prostate cancer patients with metastatic disease are treated with androgen ablation therapy. These patients respond efficiently with improvement in bone pain, regression of soft-tissue metastases, and decreases in serum PSA levels. After a period of two years, nearly all patients progress to the castrate-resistant state. Until 2004, these patients were treated for symptoms with chemotherapeutic agents, such as mitoxantrone combined with prednisone, as well as isotope therapy or external-beam radiation therapy for painful bone metastases. Two new agents were approved by the FDA in 2010, cabazitaxel (chemotherapy) and sipuleucel-T (immunotherapy), with abiraterone approved in 2011 all further boost the choice of drugs to treat this deadly bone disseminating disease (1,6-8). The primary endpoints for these drugs are vastly different as we discussed in detail earlier. The need of the hour is to research on exploration of novel biological markers to determine the appropriate drug to use in a given situation. Clearly, future studies, and eventually clinical practice, will need to incorporate newer imaging methods to track cancer cells, biological markers in blood, bone marrow, and circulating tumor cells, to determine the treatment efficacy of individual agent, or combination of hormonal agents, chemotherapy, immunotherapy and/or radiation therapy.
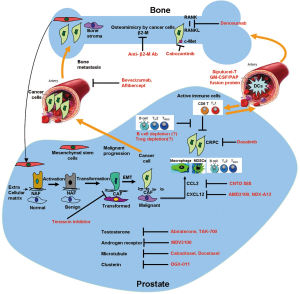
Acknowledgements
Funding: Research was supported by grants from P01-CA98912, and RO1-CA122602 to L.W.K. Chung, a Prostate Cancer Foundation Challenge Award to L.W.K.Chung and Department of Defense Awards W81XWH-08-1-0470 and PC073540 and Prosate Cancer Foundation Young Investigator Award for E.M Posadas.
The authors thank Mr. Gary Mawyer for his editorial assistance.
Footnote
Conflicts of Interest: The authors have no conflicts of interest to declare.
References
- Petrylak DP. Current clinical trials in castrate-resistant prostate cancer. Curr Urol Rep 2011;12:173-9. [PubMed]
- de Bono JS, Logothetis CJ, Molina A, et al. Abiraterone and increased survival in metastatic prostate cancer. N Engl J Med 2011;364:1995-2005. [PubMed]
- Massard C, Fizazi K. Targeting continued androgen receptor signaling in prostate cancer. Clin Cancer Res 2011;17:3876-83. [PubMed]
- Tran C, Ouk S, Clegg NJ, et al. Development of a second-generation antiandrogen for treatment of advanced prostate cancer. Science 2009;324:787-90. [PubMed]
- Scher HI, Beer TM, Higano CS, et al. Antitumour activity of MDV3100 in castration-resistant prostate cancer: a phase 1-2 study. Lancet 2010;375:1437-46. [PubMed]
- Yap TA, Zivi A, Omlin A, et al. The changing therapeutic landscape of castration-resistant prostate cancer. Nat Rev Clin Oncol 2011;8:597-610. [PubMed]
- Ong M, Winquist E. Recent advances in second-line treatment of castration-resistant prostate cancer. Curr Opin Support Palliat Care 2011;5:199-205. [PubMed]
- Attard G, de Bono JS. Translating scientific advancement into clinical benefit for castration-resistant prostate cancer patients. Clin Cancer Res 2011;17:3867-75. [PubMed]
- Molina A, Belldegrun A. Novel therapeutic strategies for castration resistant prostate cancer: inhibition of persistent androgen production and androgen receptor mediated signaling. J Urol 2011;185:787-94. [PubMed]
- Zivi A, Massard C, De-Bono J. Changing therapeutic paradigms in castrate-resistant prostate cancer. Clin Genitourin Cancer 2010;8:17-22. [PubMed]
- Mita AC, Denis LJ, Rowinsky EK, et al. Phase I and pharmacokinetic study of XRP6258 (RPR 116258A), a novel taxane, administered as a 1-hour infusion every 3 weeks in patients with advanced solid tumors. Clin Cancer Res 2009;15:723-30. [PubMed]
- de Bono JS, Oudard S, Ozguroglu M, et al. Prednisone plus cabazitaxel or mitoxantrone for metastatic castration-resistant prostate cancer progressing after docetaxel treatment: a randomised open-label trial. Lancet 2010;376:1147-54. [PubMed]
- July LV, Akbari M, Zellweger T, et al. Clusterin expression is significantly enhanced in prostate cancer cells following androgen withdrawal therapy. Prostate 2002;50:179-88. [PubMed]
- Miyake H, Nelson C, Rennie PS, et al. Acquisition of chemoresistant phenotype by overexpression of the antiapoptotic gene testosterone-repressed prostate message-2 in prostate cancer xenograft models. Cancer Res 2000;60:2547-54. [PubMed]
- Chi KN, Zoubeidi A, Gleave ME. Custirsen (OGX-011): a second-generation antisense inhibitor of clusterin for the treatment of cancer. Expert Opin Investig Drugs 2008;17:1955-62. [PubMed]
- Chi KN, Hotte SJ, Yu EY, et al. Randomized phase II study of docetaxel and prednisone with or without OGX-011 in patients with metastatic castration-resistant prostate cancer. J Clin Oncol 2010;28:4247-54. [PubMed]
- Saad F, Hotte S, North S, et al. Randomized phase II trial of Custirsen (OGX-011) in combination with docetaxel or mitoxantrone as second-line therapy in patients with metastatic castrate-resistant prostate cancer progressing after first-line docetaxel: CUOG trial P-06c. Clin Cancer Res 2011;17:5765-73. [PubMed]
- Sung SY, Chung LW. Prostate tumor-stroma interaction: molecular mechanisms and opportunities for therapeutic targeting. Differentiation 2002;70:506-21. [PubMed]
- Chung LW. Fibroblasts are critical determinants in prostatic cancer growth and dissemination. Cancer Metastasis Rev 1991;10:263-74. [PubMed]
- Camps JL, Chang SM, Hsu TC, et al. Fibroblast-mediated acceleration of human epithelial tumor growth in vivo. Proc Natl Acad Sci U S A 1990;87:75-9. [PubMed]
- Chung LW. The role of stromal-epithelial interaction in normal and malignant growth. Cancer Surv 1995;23:33-42. [PubMed]
- Thalmann GN, Rhee H, Sikes RA, et al. Human prostate fibroblasts induce growth and confer castration resistance and metastatic potential in LNCaP Cells. Eur Urol 2010;58:162-71. [PubMed]
- Chung LW, Zhau HE, Ro JY. Morphologic and biochemical alterations in rat prostatic tumors induced by fetal urogenital sinus mesenchyme. Prostate 1990;17:165-74. [PubMed]
- Chung LW, Auble K. Characterization of fetal urogenital sinus-induced prostatic hyperplasia in the mouse: time course, hormonal requirement, age dependency, and responsiveness of various adult organs to growth induction by fetal urogenital sinus tissues. Biol Reprod 1988;39:50-7. [PubMed]
- Miller GJ, Runner MN, Chung LW. Tissue interactions and prostatic growth: II. Morphological and biochemical characterization of adult mouse prostatic hyperplasia induced by fetal urogenital sinus implants. Prostate 1985;6:241-53. [PubMed]
- Chung LW, Matsuura J, Rocco AK, et al. A new mouse model for prostatic hyperplasia: induction of adult prostatic overgrowth by fetal urogenital sinus implants. Prog Clin Biol Res 1984;145:291-306. [PubMed]
- Neubauer BL, Chung LW, McCormick KA, et al. Epithelial-mesenchymal interactions in prostatic development. II. Biochemical observations of prostatic induction by urogenital sinus mesenchyme in epithelium of the adult rodent urinary bladder. J Cell Biol 1983;96:1671-6. [PubMed]
- Chung LW, Cunha GR. Stromal-epithelial interactions: II. Regulation of prostatic growth by embryonic urogenital sinus mesenchyme. Prostate 1983;4:503-11. [PubMed]
- Chung LW, Gleave ME, Hsieh JT, et al. Reciprocal mesenchymal-epithelial interaction affecting prostate tumour growth and hormonal responsiveness. Cancer Surv 1991;11:91-121. [PubMed]
- Gleave M, Hsieh JT, Gao CA, et al. Acceleration of human prostate cancer growth in vivo by factors produced by prostate and bone fibroblasts. Cancer Res 1991;51:3753-61. [PubMed]
- Rhee HW, Zhau HE, Pathak S, et al. Permanent phenotypic and genotypic changes of prostate cancer cells cultured in a three-dimensional rotating-wall vessel. In Vitro Cell Dev Biol Anim 2001;37:127-40. [PubMed]
- Sung SY, Hsieh CL, Law A, et al. Coevolution of prostate cancer and bone stroma in three-dimensional coculture: implications for cancer growth and metastasis. Cancer Res 2008;68:9996-10003. [PubMed]
- Hsieh CL, Gardner TA, Miao L, et al. Cotargeting tumor and stroma in a novel chimeric tumor model involving the growth of both human prostate cancer and bone stromal cells. Cancer Gene Ther 2004;11:148-55. [PubMed]
- Thalmann GN, Anezinis PE, Chang SM, et al. Androgen-independent cancer progression and bone metastasis in the LNCaP model of human prostate cancer. Cancer Res 1994;54:2577-81. [PubMed]
- Thalmann GN, Sikes RA, Wu TT, et al. LNCaP progression model of human prostate cancer: androgen-independence and osseous metastasis. Prostate 2000;44:91-103. [PubMed]
- Sooriakumaran P, Coley HM, Fox SB, et al. A randomized controlled trial investigating the effects of celecoxib in patients with localized prostate cancer. Anticancer Res 2009;29:1483-8. [PubMed]
- Brawer MK, Deering RE, Brown M, et al. Predictors of pathologic stage in prostatic carcinoma. The role of neovascularity. Cancer 1994;73:678-87. [PubMed]
- Figg WD, Li H, Sissung T, et al. Pre-clinical and clinical evaluation of estramustine, docetaxel and thalidomide combination in androgen-independent prostate cancer. BJU Int 2007;99:1047-55. [PubMed]
- Ning YM, Gulley JL, Arlen PM, et al. Phase II trial of bevacizumab, thalidomide, docetaxel, and prednisone in patients with metastatic castration-resistant prostate cancer. J Clin Oncol 2010;28:2070-6. [PubMed]
- Galsky MD, Vogelzang NJ. Docetaxel-based combination therapy for castration-resistant prostate cancer. Ann Oncol 2010;21:2135-44. [PubMed]
- Saylor PJ, Lee RJ, Smith MR. Emerging therapies to prevent skeletal morbidity in men with prostate cancer. J Clin Oncol 2011;29:3705-14. [PubMed]
- Yakes FM, Chen J, Tan J, et al. Cabozantinib (XL184), a novel MET and VEGFR2 inhibitor, simultaneously suppresses metastasis, angiogenesis, and tumor growth. Mol Cancer Ther 2011;10:2298-308. [PubMed]
- Ruch JM, Hussain MH. Evolving therapeutic paradigms for advanced prostate cancer. Oncology (Williston Park) 2011;25:496-504,508.
- Drake JM, Danke JR, Henry MD. Bone-specific growth inhibition of prostate cancer metastasis by atrasentan. Cancer Biol Ther 2010;9:607-14. [PubMed]
- Posadas EM, Al-Ahmadie H, Robinson VL, et al. FYN is overexpressed in human prostate cancer. BJU Int 2009;103:171-7. [PubMed]
- Saito YD, Jensen AR, Salgia R, et al. Fyn: a novel molecular target in cancer. Cancer 2010;116:1629-37. [PubMed]
- Yu EY, Wilding G, Posadas E, et al. Phase II study of dasatinib in patients with metastatic castration-resistant prostate cancer. Clin Cancer Res 2009;15:7421-8. [PubMed]
- Araujo JC, Mathew P, Armstrong AJ, et al. Dasatinib combined with docetaxel for castration-resistant prostate cancer: Results from a phase 1-2 study. Cancer 2012;118:63-71. [PubMed]
- Zhang J, Dai J, Yao Z, et al. Soluble receptor activator of nuclear factor kappaB Fc diminishes prostate cancer progression in bone. Cancer Res 2003;63:7883-90. [PubMed]
- Fizazi K, Carducci M, Smith M, et al. Denosumab versus zoledronic acid for treatment of bone metastases in men with castration-resistant prostate cancer: a randomised, double-blind study. Lancet 2011;377:813-22. [PubMed]
- Stopeck AT, Lipton A, Body JJ, et al. Denosumab compared with zoledronic acid for the treatment of bone metastases in patients with advanced breast cancer: a randomized, double-blind study. J Clin Oncol 2010;28:5132-9. [PubMed]
- Cummings SR, Tice JA, Bauer S, et al. Prevention of breast cancer in postmenopausal women: approaches to estimating and reducing risk. J Natl Cancer Inst 2009;101:384-98. [PubMed]
- Widmark A, Klepp O, Solberg A, et al. Endocrine treatment, with or without radiotherapy, in locally advanced prostate cancer (SPCG-7/SFUO-3): an open randomised phase III trial. Lancet 2009;373:301-8. [PubMed]
- Reid AH, Attard G, Danila DC, et al. Significant and sustained antitumor activity in post-docetaxel, castration-resistant prostate cancer with the CYP17 inhibitor abiraterone acetate. J Clin Oncol 2010;28:1489-95. [PubMed]
- Ruiz C, Huang W, Hegi ME, et al. Growth promoting signaling by tenascin-C Cancer Res 2004;64:7377-85. [corrected]. [PubMed]
- Josson S, Nomura T, Lin JT, et al. β2-microglobulin induces epithelial to mesenchymal transition and confers cancer lethality and bone metastasis in human cancer cells. Cancer Res 2011;71:2600-10. [PubMed]
- Duda DG, Kozin SV, Kirkpatrick ND, et al. CXCL12 (SDF1alpha)-CXCR4/CXCR7 pathway inhibition: an emerging sensitizer for anticancer therapies? Clin Cancer Res 2011;17:2074-80. [PubMed]
- Mizutani K, Sud S, Pienta KJ. Prostate cancer promotes CD11b positive cells to differentiate into osteoclasts. J Cell Biochem 2009;106:563-9. [PubMed]
- Qian DZ, Rademacher BL, Pittsenbarger J, et al. CCL2 is induced by chemotherapy and protects prostate cancer cells from docetaxel-induced cytotoxicity. Prostate 2010;70:433-42. [PubMed]
- Drake CG. Prostate cancer as a model for tumour immunotherapy. Nat Rev Immunol 2010;10:580-93. [PubMed]
- Cheever MA, Higano CS. PROVENGE (Sipuleucel-T) in prostate cancer: the first FDA-approved therapeutic cancer vaccine. Clin Cancer Res 2011;17:3520-6. [PubMed]
- Kantoff PW, Higano CS, Shore ND, et al. Sipuleucel-T immunotherapy for castration-resistant prostate cancer. N Engl J Med 2010;363:411-22. [PubMed]
- Katsnelson A. Kicking off adaptive immunity: the discovery of dendritic cells. J Exp Med 2006;203:1622. [PubMed]
- Gulley JL, Drake CG. Immunotherapy for prostate cancer: recent advances, lessons learned, and areas for further research. Clin Cancer Res 2011;17:3884-91. [PubMed]
- Dummer R. GVAX (Cell Genesys). Curr Opin Investig Drugs 2001;2:844-8. [PubMed]
- Josson S, Matsuoka Y, Chung LW, et al. Tumor-stroma co-evolution in prostate cancer progression and metastasis. Semin Cell Dev Biol 2010;21:26-32. [PubMed]
- Chung LW, Baseman A, Assikis V, et al. Molecular insights into prostate cancer progression: the missing link of tumor microenvironment. J Urol 2005;173:10-20. [PubMed]
- Stadler WM, Cao D, Vogelzang NJ, et al. A randomized Phase II trial of the antiangiogenic agent SU5416 in hormone-refractory prostate cancer. Clin Cancer Res 2004;10:3365-70. [PubMed]
- Ferrara N. VEGF as a therapeutic target in cancer. Oncology 2005;69:11-6. [PubMed]
- Sonpavde G, Periman PO, Bernold D, et al. Sunitinib malate for metastatic castration-resistant prostate cancer following docetaxel-based chemotherapy. Ann Oncol 2010;21:319-24. [PubMed]
- Yang JC, Bai L, Yap S, et al. Effect of the specific Src family kinase inhibitor saracatinib on osteolytic lesions using the PC-3 bone model. Mol Cancer Ther 2010;9:1629-37. [PubMed]
- Smith MR, Saad F, Coleman R, et al. Denosumab and bone-metastasis-free survival in men with castration-resistant prostate cancer: results of a phase 3, randomised, placebo-controlled trial. Lancet 2012;379:39-46. [PubMed]
- Lewington VJ, McEwan AJ, Ackery DM, et al. A prospective, randomised double-blind crossover study to examine the efficacy of strontium-89 in pain palliation in patients with advanced prostate cancer metastatic to bone. Eur J Cancer 1991;27:954-8. [PubMed]
- Collins C, Eary JF, Donaldson G, et al. Samarium-153-EDTMP in bone metastases of hormone refractory prostate carcinoma: a phase I/II trial. J Nucl Med 1993;34:1839-44. [PubMed]
- Tanaka H, Kono E, Tran CP, et al. Monoclonal antibody targeting of N-cadherin inhibits prostate cancer growth, metastasis and castration resistance. Nat Med 2010;16:1414-20. [PubMed]
- Zhau HE, Odero-Marah V, Lue HW, et al. Epithelial to mesenchymal transition (EMT) in human prostate cancer: lessons learned from ARCaP model. Clin Exp Metastasis 2008;25:601-10. [PubMed]
- Xu J, Wang R, Xie ZH, et al. Prostate cancer metastasis: role of the host microenvironment in promoting epithelial to mesenchymal transition and increased bone and adrenal gland metastasis. Prostate 2006;66:1664-73. [PubMed]
- Odero-Marah VA, Wang R, Chu G, et al. Receptor activator of NF-kappaB Ligand (RANKL) expression is associated with epithelial to mesenchymal transition in human prostate cancer cells. Cell Res 2008;18:858-70. [PubMed]
- Hu P, Chu GC, Zhu G, et al. Multiplexed Quantum Dot Labeling of Activated c-Met Signaling in Castration-Resistant Human Prostate Cancer. PLoS One 2011;6:e28670. [PubMed]
- Huang WC, Havel JJ, Zhau HE, et al. Beta2-microglobulin signaling blockade inhibited androgen receptor axis and caused apoptosis in human prostate cancer cells. Clin Cancer Res 2008;14:5341-7. [PubMed]
- Huang WC, Wu D, Xie Z, et al. beta2-microglobulin is a signaling and growth-promoting factor for human prostate cancer bone metastasis. Cancer Res 2006;66:9108-16. [PubMed]
- Tan W, Zhang W, Strasner A, et al. Tumour-infiltrating regulatory T cells stimulate mammary cancer metastasis through RANKL-RANK signalling. Nature 2011;470:548-53. [PubMed]
- Ammirante M, Luo JL, Grivennikov S, et al. B-cell-derived lymphotoxin promotes castration-resistant prostate cancer. Nature 2010;464:302-5. [PubMed]
- Andreu P, Johansson M, Affara NI, et al. FcRgamma activation regulates inflammation-associated squamous carcinogenesis. Cancer Cell 2010;17:121-34. [PubMed]
- de Visser KE, Korets LV, Coussens LM. De novo carcinogenesis promoted by chronic inflammation is B lymphocyte dependent. Cancer Cell 2005;7:411-23. [PubMed]
- Sfanos KS, Bruno TC, Maris CH, et al. Phenotypic analysis of prostate-infiltrating lymphocytes reveals TH17 and Treg skewing. Clin Cancer Res 2008;14:3254-61. [PubMed]
- Hanahan D, Weinberg RA. Hallmarks of cancer: the next generation. Cell 2011;144:646-74. [PubMed]
- Keibel A, Singh V, Sharma MC. Inflammation, microenvironment, and the immune system in cancer progression. Curr Pharm Des 2009;15:1949-55. [PubMed]
- Müller-Hübenthal B, Azemar M, Lorenzen D, et al. Tumour Biology: tumour-associated inflammation versus antitumor immunity. Anticancer Res 2009;29:4795-805. [PubMed]
- Mantovani A, Allavena P, Sica A, et al. Cancer-related inflammation. Nature 2008;454:436-44. [PubMed]
- Coussens LM, Werb Z. Inflammation and cancer. Nature 2002;420:860-7. [PubMed]
- Schenk JM, Kristal AR, Neuhouser ML, et al. Biomarkers of systemic inflammation and risk of incident, symptomatic benign prostatic hyperplasia: results from the prostate cancer prevention trial. Am J Epidemiol 2010;171:571-82. [PubMed]
- Sfanos KS, Bruno TC, Meeker AK, et al. Human prostate-infiltrating CD8+ T lymphocytes are oligoclonal and PD-1+. Prostate 2009;69:1694-703. [PubMed]
- Bruno TC, Rothwell C, Grosso JF, et al. Anti-tumor effects of endogenous prostate cancer-specific CD8 T cells in a murine TCR transgenic model. Prostate 2012;72:514-22. [PubMed]
- Zou W, Chen L. Inhibitory B7-family molecules in the tumour microenvironment. Nat Rev Immunol 2008;8:467-77. [PubMed]
- Qian BZ, Pollard JW. Macrophage diversity enhances tumor progression and metastasis. Cell 2010;141:39-51. [PubMed]
- Nonomura N, Takayama H, Nakayama M, et al. Infiltration of tumour-associated macrophages in prostate biopsy specimens is predictive of disease progression after hormonal therapy for prostate cancer. BJU Int 2011;107:1918-22. [PubMed]
- Qian B, Deng Y, Im JH, et al. A distinct macrophage population mediates metastatic breast cancer cell extravasation, establishment and growth. PLoS One 2009;4:e6562. [PubMed]
- Oberlies J, Watzl C, Giese T, et al. Regulation of NK cell function by human granulocyte arginase. J Immunol 2009;182:5259-67. [PubMed]
- Toh B, Wang X, Keeble J, et al. Mesenchymal transition and dissemination of cancer cells is driven by myeloid-derived suppressor cells infiltrating the primary tumor. PLoS Biol 2011;9:e1001162. [PubMed]
- Zamarron BF, Chen W. Dual roles of immune cells and their factors in cancer development and progression. Int J Biol Sci 2011;7:651-8. [PubMed]
- Umemura N, Saio M, Suwa T, et al. Tumor-infiltrating myeloid-derived suppressor cells are pleiotropic-inflamed monocytes/macrophages that bear M1- and M2-type characteristics. J Leukoc Biol 2008;83:1136-44. [PubMed]
- Park SI, Soki FN, McCauley LK. Roles of bone marrow cells in skeletal metastases: no longer bystanders. Cancer Microenviron 2011;4:237-46. [PubMed]
- Volchenkov R, Sprater F, Vogelsang P, et al. The 2011 Nobel Prize in physiology or medicine. Scand J Immunol 2012;75:1-4. [PubMed]
- Mellman I, Nussenzweig M. Retrospective. Ralph M. Steinman (1943-2011). Science 2011;334:466. [PubMed]
- Shortman K. Ralph Steinman and dendritic cells. Immunol Cell Biol 2012;90:1-2. [PubMed]
- Travis J. Nobel Prize in physiology or medicine. Immunology prize overshadowed by untimely death of awardee. Science 2011;334:31. [PubMed]
- May KF Jr, Gulley JL, Drake CG, et al. Prostate cancer immunotherapy. Clin Cancer Res 2011;17:5233-8. [PubMed]
- Kantoff PW, Schuetz TJ, Blumenstein BA, et al. Overall survival analysis of a phase II randomized controlled trial of a Poxviral-based PSA-targeted immunotherapy in metastatic castration-resistant prostate cancer. J Clin Oncol 2010;28:1099-105. [PubMed]
- Fong L, Kwek SS, O'Brien S, et al. Potentiating endogenous antitumor immunity to prostate cancer through combination immunotherapy with CTLA4 blockade and GM-CSF. Cancer Res 2009;69:609-15. [PubMed]
- Egen JG, Kuhns MS, Allison JP. CTLA-4: new insights into its biological function and use in tumor immunotherapy. Nat Immunol 2002;3:611-8. [PubMed]
- Thompson RH, Kwon ED, Allison JP. Inhibitors of B7-CD28 costimulation in urologic malignancies. Immunotherapy 2009;1:129-39. [PubMed]
- Yuan J, Ginsberg B, Page D, et al. CTLA-4 blockade increases antigen-specific CD8(+) T cells in prevaccinated patients with melanoma: three cases. Cancer Immunol Immunother 2011;60:1137-46. [PubMed]
- Hurwitz AA, Yanover P, Markowitz M, et al. Prostate cancer: advances in immunotherapy. BioDrugs 2003;17:131-8. [PubMed]