The disease burden of kidney cancer in Asian countries and regions from 1990 to 2021: a study based on the Global Burden of Disease database
Highlight box
Key findings
• The kidney cancer (KC) burden has increased significantly in most Asian countries and regions, and the sociodemographic index (SDI) was significantly positively correlated with the KC burden.
• Among all risk factors, the KC burden attributed to high body mass index (HBMI) was still increasing and also significantly positively correlated with SDI.
What is known and what is new?
• KC is a common urinary system tumor with poor prognosis and powerful threat. The KC burden in developed countries is usually higher than that in developing countries, but there are no related studies using the Global Burden of Disease 2021 data to assess the burden of KC in Asia comprehensively.
• This study highlights that the burden of KC is increasing in Asia and HBMI is a risk factor worthy of attention.
What is the implication, and what should change now?
• Our study can provide valuable information for health policy makers to formulate the better KC prevention and treatment policies in Asia in the future, and we found that diet control and moderate exercise to control body mass index could be an important measure to decrease the KC burden.
Introduction
Kidney cancer (KC) is a common urinary system tumor with poor prognosis. More than 90% of KCs are renal cell carcinomas (RCCs), which originate from the renal tubular epithelium T, and the most common type of KC in children is Wilms tumor (1,2). The global incidence of KC has been increasing since the 1970s, with great differences between countries (3). Overall, the incidence rate (IR) of KC in males is 1.5 times higher than that of in females, with the IR in developed countries, including high-income countries such as the United States, European countries, and Australia, usually being higher than that in developing countries (4,5). The mortality rate (MR) in high-income countries has peaked and started to decline, while the MR in Asian and African countries has remained relatively stable (6,7). Well-recognized risk factors that are significantly associated with the development of KC include smoking, obesity, and hypertension (2,6,8). Therefore, KC is a concerning threat to human health. Revealing the spatial and temporal disease burden of KC will help to better understand the epidemiology of KC, thereby providing key references for the prevention and management of KC.
Compared with Europe, America, and Africa, Asia has the largest population and is mainly composed of developing countries. Additionally, the incidence of KC has increased rapidly in recent years. Changes in the disease burden of KC in Asia will have an important impact on the global burden of KC. Up-to-date information on the incidence, prevalence, mortality, and disability-adjusted life years (DALYs) of KC in Asia is essential for informed medical decision-making in Asia. These data can be used to develop effective prevention programs, allocate resources appropriately, and identify priority research areas.
The Global Burden of Disease (GBD) database provides a unique framework for assessing disease burden using multiple epidemiological indicators. Previous studies have used data from GBD 2017 and GBD 2019 databases to estimate the global burden of KC (8,9), and some studies have reported the burden of KC in some regions and countries (1,10,11). However, there are no studies using the latest GBD 2021 data, nor are there any studies specifically targeting the burden of KC in Asia. Therefore, this study conducted comprehensive analyses of the KC burden in Asia from 1990 to 2021, aiming to provide valuable information for the formulation of better KC prevention and treatment policies in Asia in the future. We present this article in accordance with the STROBE reporting checklist (available at https://tau.amegroups.com/article/view/10.21037/tau-2024-652/rc).
Methods
Acquisition of KC disease burden data
The disease burden data of KC were downloaded from the GBD database (2021, https://ghdx.healthdata.org/). All official Asian countries and regions included in GBD were summarized in Table 1. The relevant indicators included the incidence, prevalence, mortality, DALYs and risk factors of KC in Asia and in Asian countries and regions. The sociodemographic index (SDI) values were also obtained, which reflected the comprehensive development level of the countries and regions in Asia and could be used to study the correlation between the burden of KC and the degree of social development in different Asian countries and regions. The specific definitions and calculation methods of the above indicators have been described in detail in previous studies (12-14). The study was conducted in accordance with the Declaration of Helsinki and its subsequent amendments.
Table 1
Administrative division | Official countries and regions |
---|---|
Central Asia | Armenia, Azerbaijan, Georgia, Kazakhstan, Kyrgyzstan, Mongolia, Tajikistan, Turkmenistan, Uzbekistan |
South Asia | Bangladesh, Bhutan, India, Nepal, Pakistan |
East Asia | China, North Korea, Japan, South Korea, Taiwan |
Southeast Asia | Brunei, Cambodia, Indonesia, Laos, Malaysia, Maldives, Mauritius, Myanmar, Philippines, Seychelles, Singapore, Sri Lanka, Thailand, Timor-Leste, Viet Nam |
Western Asia | Afghanistan, Algeria, Bahrain, Iran, Iraq, Jordan, Kuwait, Lebanon, Oman, Palestine, Qatar, Saudi Arabia, Syria, Turkey, United Arab Emirates, Yemen |
The joinpoint regression and age-period-cohort model
Joinpoint regression analyses were used to evaluate the temporal trends of the IR, prevalence rate (PR), MR, and DALY rate (DR) of KC in Asia. The Joinpoint regression model could identify multiple inflection points in time series data to determine significant trend shifts, which could help decision makers better understand the long-term trends in cancer epidemiology, evaluate the effectiveness of prevention and control measures, and provide information for future policy decisions. The points (joinpoints) where the trend changed significantly were found, and the overall trend of KC was finally fitted (15). The joinpoint software could output the best fitting model, then calculate the annual percentage change (APC) for each period, the overall average annual percentage change (AAPC), and the corresponding P values. APC (or AAPC) >0 indicated an increase in the burden of KC, APC (or AAPC) <0 indicates a decrease in the burden of KC, and APC (or AAPC) =0 indicated no change (16).
Since age, period and birth cohort were confounding factors, we applied the age-period-cohort model to assess the effects of age, period and birth cohort on KC burden. The age effect reflected the relationship between age increase and KC burden; the period effect demonstrated the relationship between the trend of social change and KC burden; and the cohort effect emphasized the relationship between birth cohort and KC burden (17,18). Stata software was used to calculate the coefficients of age, period and cohort, and then converted the coefficients into relative risk (RR) values.
Health inequalities, frontier analyses and the autoregressive integrated moving average (ARIMA) model
The concentration index (CI) was used to measure the degree of health inequalities and was calculated using the health equity assessment toolkit (HEAT) software (19). The software had powerful computational capabilities to display disaggregated data and summary indicators of inequality in an interactive and customizable way, thus facilitating the interpretation and reporting of health inequalities in specific countries. Based on the SDI, the cumulative scores of epidemiological indicators contained in the GBD database and the cumulative relative distribution of the population were fitted into a Lorenz curve. When the curve was above the diagonal, it meant that the disease burden was concentrated in relatively poor countries, and the CI should be negative. When the curve was below the diagonal, it meant that the disease burden was concentrated in relatively wealthy countries, and the CI should be positive (20,21).
The frontier analyses were applied to assess the relationship between KC burden and sociodemographic development. The frontier indicated the minimum achievable burden based on development status. The distance between epidemiological indicators of a country or region and the frontier of a country or region represented the health gains that were not achieved based on the current level of development. We referred to previous studies for detailed descriptions (22-24).
We fitted the changes in KC burden from 1990 to 2021 and used the ARIMA model to predict the KC burden for the next 15 years (25).
Statistical analyses
The correlation between SDI and KC burden was performed using the Spearman test. All statistical analyses were completed using R software 4.3.2 (including frontier analyses), Stata 18, and Graphpad 10.1.2. P<0.05 was considered statistically significant.
Results
KC burden in Asian countries and regions
The comprehensive analyses of the KC burden in Asian countries and regions were conducted. The relevant indicators included age standardized incidence rate (ASIR), age standardized prevalence rate (ASPR), age standardized mortality rate (ASMR), and age standardized DALY rate (ASDR). The data for the above four indicators in 1990 were visualized in Figure 1, while the data for 2021 were visualized in Figure 2, and the specific details were summarized in Table 2.
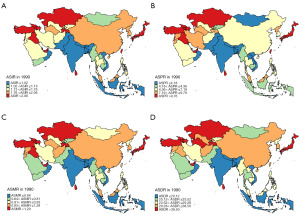
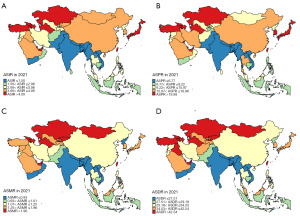
Table 2
Location | ASIR | ASPR | ASMR | ASDR | |||||||
---|---|---|---|---|---|---|---|---|---|---|---|
1990 | 2021 | 1990 | 2021 | 1990 | 2021 | 1990 | 2021 | ||||
Afghanistan | 1.48 | 2.06 | 6.02 | 9.75 | 1.07 | 1.21 | 31.37 | 34.54 | |||
Algeria | 0.58 | 1.16 | 2.67 | 6.44 | 0.38 | 0.56 | 9.91 | 13.35 | |||
Armenia | 3.26 | 5.23 | 11.91 | 22.05 | 2.06 | 2.85 | 56.51 | 72.93 | |||
Azerbaijan | 3.79 | 5.07 | 13.80 | 20.86 | 2.49 | 2.91 | 77.96 | 85.44 | |||
Bahrain | 3.96 | 5.48 | 17.53 | 30.86 | 2.55 | 2.48 | 61.81 | 57.26 | |||
Bangladesh | 0.70 | 0.88 | 1.78 | 3.15 | 0.60 | 0.60 | 17.59 | 16.14 | |||
Bhutan | 0.68 | 1.14 | 1.78 | 4.07 | 0.58 | 0.79 | 17.06 | 21.39 | |||
Brunei | 3.58 | 4.39 | 10.26 | 17.2 | 2.76 | 2.71 | 67.48 | 65.14 | |||
Cambodia | 1.12 | 1.73 | 4.26 | 7.71 | 0.79 | 1.01 | 24.02 | 29.17 | |||
China | 1.79 | 3.32 | 7.19 | 17.75 | 1.14 | 1.25 | 35.84 | 34.18 | |||
North Korea | 1.78 | 2.17 | 8.95 | 11.57 | 0.90 | 0.93 | 28.87 | 29.18 | |||
Georgia | 2.93 | 5.58 | 12.33 | 22.99 | 1.63 | 3.13 | 49.16 | 87.31 | |||
India | 0.56 | 1.02 | 1.51 | 3.51 | 0.47 | 0.69 | 13.67 | 18.40 | |||
Indonesia | 1.02 | 1.80 | 4.36 | 8.23 | 0.64 | 0.99 | 20.12 | 28.87 | |||
Iran | 1.76 | 2.97 | 9.76 | 18.33 | 0.93 | 1.16 | 28.86 | 30.71 | |||
Iraq | 2.13 | 3.48 | 10.25 | 19.66 | 1.33 | 1.59 | 38.5 | 43.46 | |||
Japan | 3.40 | 4.75 | 15.24 | 24.92 | 1.75 | 1.96 | 43.68 | 42.54 | |||
Jordan | 1.54 | 2.66 | 7.55 | 15.84 | 0.95 | 1.15 | 25.82 | 30.41 | |||
Kazakhstan | 4.15 | 4.87 | 15.49 | 21.58 | 2.62 | 2.57 | 77.6 | 73.09 | |||
Kuwait | 1.86 | 3.82 | 10.44 | 24.62 | 0.94 | 1.36 | 25.29 | 34.81 | |||
Kyrgyzstan | 1.20 | 3.77 | 4.23 | 15.97 | 0.81 | 2.14 | 24.41 | 61.35 | |||
Laos | 1.11 | 1.50 | 3.87 | 6.18 | 0.81 | 0.93 | 25.27 | 27.51 | |||
Lebanon | 2.04 | 3.93 | 9.90 | 23.17 | 1.25 | 1.74 | 32.14 | 41.56 | |||
Malaysia | 1.89 | 2.85 | 7.88 | 13.69 | 1.12 | 1.38 | 29.28 | 34.76 | |||
Maldives | 0.97 | 1.14 | 3.57 | 5.86 | 0.67 | 0.52 | 17.06 | 13.50 | |||
Mauritius | 1.49 | 2.22 | 7.21 | 11.55 | 0.76 | 0.99 | 21.47 | 27.48 | |||
Mongolia | 1.13 | 4.09 | 3.18 | 15.21 | 0.87 | 2.59 | 23.52 | 73.17 | |||
Myanmar | 1.11 | 1.48 | 4.22 | 6.77 | 0.76 | 0.85 | 24.33 | 25.75 | |||
Nepal | 0.56 | 0.89 | 1.41 | 2.89 | 0.48 | 0.65 | 14.09 | 16.92 | |||
Oman | 0.91 | 1.82 | 4.18 | 10.3 | 0.59 | 0.81 | 15.61 | 20.63 | |||
Pakistan | 0.84 | 1.30 | 2.01 | 3.84 | 0.72 | 0.98 | 20.60 | 28.47 | |||
Palestine | 2.08 | 2.74 | 10.12 | 15.48 | 1.25 | 1.28 | 33.36 | 33.2 | |||
Philippines | 1.56 | 2.10 | 6.86 | 9.22 | 0.86 | 1.11 | 28.35 | 34.53 | |||
Qatar | 3.77 | 4.62 | 17.19 | 27.18 | 2.38 | 1.95 | 56.77 | 45.16 | |||
South Korea | 1.85 | 4.02 | 6.55 | 21.18 | 1.25 | 1.61 | 33.9 | 36.79 | |||
Saudi Arabia | 1.19 | 3.48 | 5.55 | 19.98 | 0.77 | 1.49 | 20.77 | 38.08 | |||
Seychelles | 2.11 | 2.66 | 9.78 | 13.05 | 1.16 | 1.28 | 32.76 | 34.20 | |||
Singapore | 2.73 | 3.86 | 10.23 | 20.08 | 1.71 | 1.62 | 42.94 | 36.24 | |||
Sri Lanka | 5.65 | 1.93 | 25.07 | 10.14 | 3.23 | 0.82 | 86.58 | 21.21 | |||
Syria | 1.74 | 2.76 | 8.48 | 15.75 | 1.08 | 1.27 | 30.12 | 32.12 | |||
Taiwan | 2.93 | 9.29 | 16.98 | 56.73 | 1.06 | 2.76 | 30.34 | 69.05 | |||
Tajikistan | 2.49 | 2.11 | 8.08 | 7.56 | 1.82 | 1.43 | 52.1 | 41.53 | |||
Thailand | 1.54 | 3.06 | 7.19 | 17.48 | 0.82 | 1.14 | 22.99 | 33.41 | |||
Timor-Leste | 0.88 | 1.10 | 3.43 | 4.62 | 0.60 | 0.67 | 19.11 | 19.97 | |||
Turkey | 3.39 | 4.90 | 17.02 | 30.33 | 2.00 | 1.94 | 58.92 | 51.88 | |||
Turkmenistan | 2.06 | 5.54 | 8.79 | 22.20 | 1.29 | 3.30 | 48.62 | 99.44 | |||
United Arab Emirates | 2.95 | 4.73 | 13.74 | 23.54 | 1.85 | 2.54 | 49.02 | 56.30 | |||
Uzbekistan | 1.47 | 2.39 | 5.77 | 9.50 | 0.92 | 1.44 | 29.99 | 42.24 | |||
Viet Nam | 0.51 | 1.18 | 2.15 | 5.92 | 0.31 | 0.54 | 8.38 | 14.17 | |||
Yemen | 1.08 | 1.42 | 4.68 | 6.90 | 0.75 | 0.84 | 22.39 | 23.14 |
ASIR, age standardized incidence rate; ASPR, age standardized prevalence rate; ASMR, age standardized mortality rate; ASDR, age standardized disability-adjusted life year rate.
The three countries and regions with the highest ASIR in 1990 were Sri Lanka, Kazakhstan and Bahrain. The three countries and regions with the highest ASIR in 2021 were Taiwan, Georgia and Turkmenistan. The three countries and regions with the largest increase in ASIR from 1990 to 2021 were Mongolia (362%), Taiwan (317%) and Kyrgyzstan (314%). The three countries and regions with the highest ASPR in 1990 were Sri Lanka, Bahrain and Qatar. The three countries and regions with the highest ASPR in 2021 were Taiwan, Bahrain and Turkey. The three countries and regions with the largest increase in ASPR from 1990 to 2021 were Mongolia (478%), Kyrgyzstan (378%) and Saudi Arabia (360%). The three countries and regions with the highest ASMR in 1990 were Sri Lanka, Brunei and Kazakhstan. The three countries and regions with the highest ASMR in 2021 were Turkmenistan, Georgia and Azerbaijan. The three countries and regions with the largest increase in ASMR from 1990 to 2021 were Mongolia (298%), Kyrgyzstan (264%) and Taiwan (260%). The three countries and regions with the highest ASDR in 1990 were Sri Lanka, Azerbaijan and Kazakhstan. The three countries and regions with the highest ASDR in 2021 were Turkmenistan, Georgia and Azerbaijan. The three countries and regions with the largest increase in ASDR from 1990 to 2021 were Mongolia (311%), Kyrgyzstan (251%) and Taiwan (228%).
Sex differences in KC burden
Through preliminary analyses and joinpoint analyses, we found that ASIR and ASPR showed a significant upward trend in both, female, and male subgroups (Figure 3A-3D, Tables S1,S2). ASMR showed a slight upward trend in all three subgroups, but the degree of increase has slowed down, and the ASMR of females has declined since 2002 (Figure 3E,3F, Table S3). ASDR showed a slight upward trend in both and male subgroups, but the ASDR of both groups showed a slight downward trend after 2003, and that of females showed a slight downward trend (Figure 3G,3H, Table S4).
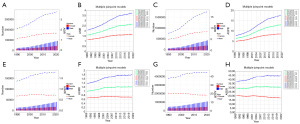
In 1990, the IR, PR, MR, DR and the corresponding absolute number of KC all showed similar trends, with a peak at the <5 years stage, followed by a gradual decline to the lowest point at the 15–19 years stage, and then an upward trend. Except for PR, which showed a downward trend after the 70–74 years stage, the other indicators remained on the rise (Figure S1A-S1H). In 2021, the IR, PR, MR, DR and the corresponding absolute number of KC were basically the same as in 1990, but the peak in the <5 years stage in 1990 disappeared. In most cases, IR, PR, MR, DR and the corresponding absolute number of male subgroup were greater than those of female subgroup (Figure S1I-S1P).
Through ARIMA prediction, except for ASMR of female, ASDR of both, and ASDR of female, the remaining indicators would increase significantly in the next 15 years (Figure S2A-S2L).
The age-period-cohort model and health inequalities
We conducted age-period-cohort model analyses on the RR of KC incidence and mortality in three dimensions: age, period, and birth cohort. After adjusting for period and birth cohort, the RR of KC incidence first decreased and then increased with age, with the lowest point at age 15. After adjusting for age and birth cohort, the RR of KC incidence increased with period. After adjusting for age and period, the RR of KC incidence decreased with birth cohort (Figure S3A-S3C). After adjusting for period and birth cohort, the RR of KC mortality first decreased and then increased with age, with the lowest point at age 15. After adjusting for age and birth cohort, the RR of KC mortality increased with period. After adjusting for age and period, the RR of KC mortality first increased and then decreased with birth cohort, with the highest point at 1915–1919 (Figure S3D-S3F).
Health inequalities analyses were conducted subsequently. The Lorenz curve and the CIs of two years (1990 and 2021) both indicated that the disease burden of the four indicators of KC was concentrated in relatively wealthy countries, and the disease burden of KC in wealthy countries increased slightly in 2021 (Figure 4A-4D). ASIR, ASPR, ASMR and ASDR were all positively correlated with SDI (Figure 4E-4H).
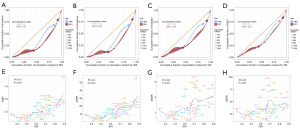
Frontier analyses
In the frontier analyses of ASIR, ASPR, ASMR and ASDR based on KC in Asian countries and regions from 1990 to 2021, with the increase of SDI, ASIR, ASPR, ASMR and ASDR showed an upward trend and were further away from the frontier (Figure 5A-5D). The results of the frontier analyses in 2021 suggested that in terms of ASIR, ten countries and regions such as Taiwan, Georgia and Turkmenistan had significantly higher ASIR and were far from the frontier. In contrast, the ASIR of countries and regions such as Bangladesh, Nepal and India were closer to the frontier (Figure 5E). When evaluating ASPR, ten countries and regions such as Taiwan, Bahrain and Qatar had significantly higher ASPR and were far from the frontier. In contrast, the ASPR of countries such as Nepal, Bangladesh and India were closer to the frontier (Figure 5F). When evaluating ASMR, ten countries and regions such as Turkmenistan, Georgia and Azerbaijan had significantly higher ASMR and were far from the frontier. In contrast, the ASMR of countries such as Maldives, Viet Nam and Algeria were closer to the frontier (Figure 5G). When evaluating ASDR, ten countries and regions such as Turkmenistan, Georgia and Azerbaijan had significantly higher ASDR and were far from the frontier. In contrast, the ASDR of countries such as Maldives, Viet Nam and Algeria were closer to the frontier (Figure 5H).
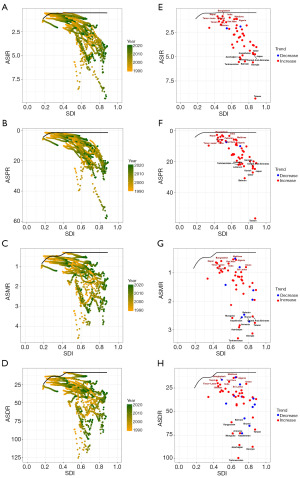
KC burden attributable to high body mass index (HBMI) in Asia
We analyzed the risk factors attributable to ASMR and ASDR of KC. Three risk factors for KC were found in the GBD database (HBMI, smoking, and occupational carcinogens). The results showed that the ASMR and ASDR attributable to HBMI increased significantly over time, while the other two risk factors did not change significantly (Figure S4A,S4B).
ASMR [1990 (Figure S5A) and 2021 (Figure S5B)] and ASDR [1990 (Figure S5C) and 2021 (Figure S5D)] attributed to HBMI in Asia were summarized (Table 3). The three countries and regions with the highest ASMR in 1990 were Qatar, Azerbaijan and Kazakhstan. The three countries and regions with the highest ASMR in 2021 were United Arab Emirates, Bahrain and Turkmenistan. The three countries and regions with the largest increase in ASMR from 1990 to 2021 were Taiwan (460%), Kyrgyzstan (340%) and Turkmenistan (333%). The three countries and regions with the highest ASDR in 1990 were Azerbaijan, Kazakhstan and Qatar. The three countries and regions with the highest ASDR in 2021 were Turkmenistan, Georgia and Azerbaijan. The three countries and regions with the largest increase in ASDR from 1990 to 2021 were Taiwan (420%), Viet Nam (395%) and Kyrgyzstan (353%).
Table 3
Location | ASMR | ASDR | |||
---|---|---|---|---|---|
1990 | 2021 | 1990 | 2021 | ||
Afghanistan | 0.13 | 0.21 | 3.65 | 5.71 | |
Algeria | 0.05 | 0.13 | 1.08 | 2.87 | |
Armenia | 0.40 | 0.65 | 10.43 | 16.40 | |
Azerbaijan | 0.48 | 0.69 | 13.94 | 18.99 | |
Bahrain | 0.47 | 0.70 | 11.46 | 16.42 | |
Bangladesh | 0.02 | 0.04 | 0.42 | 0.99 | |
Bhutan | 0.05 | 0.12 | 1.51 | 3.11 | |
Brunei | 0.22 | 0.38 | 6.16 | 10.65 | |
Cambodia | 0.03 | 0.06 | 0.85 | 1.80 | |
China | 0.07 | 0.17 | 1.76 | 4.62 | |
North Korea | 0.04 | 0.08 | 1.14 | 2.09 | |
Georgia | 0.32 | 0.69 | 9.28 | 19.37 | |
India | 0.02 | 0.06 | 0.46 | 1.54 | |
Indonesia | 0.03 | 0.08 | 0.79 | 2.52 | |
Iran | 0.11 | 0.28 | 2.96 | 6.96 | |
Iraq | 0.29 | 0.42 | 7.81 | 11.05 | |
Japan | 0.15 | 0.22 | 3.72 | 4.81 | |
Jordan | 0.21 | 0.35 | 5.36 | 8.84 | |
Kazakhstan | 0.48 | 0.57 | 13.27 | 15.86 | |
Kuwait | 0.21 | 0.45 | 5.35 | 11.02 | |
Kyrgyzstan | 0.15 | 0.51 | 4.00 | 14.14 | |
Laos | 0.03 | 0.08 | 1.00 | 2.26 | |
Lebanon | 0.24 | 0.48 | 6.00 | 11.24 | |
Malaysia | 0.11 | 0.20 | 2.92 | 5.31 | |
Maldives | 0.04 | 0.06 | 1.04 | 1.64 | |
Mauritius | 0.08 | 0.17 | 2.33 | 4.75 | |
Mongolia | 0.16 | 0.50 | 4.14 | 13.89 | |
Myanmar | 0.03 | 0.06 | 1.04 | 1.88 | |
Nepal | 0.02 | 0.05 | 0.47 | 1.39 | |
Oman | 0.08 | 0.23 | 2.23 | 5.98 | |
Pakistan | 0.04 | 0.12 | 1.14 | 3.15 | |
Palestine | 0.25 | 0.36 | 6.26 | 9.01 | |
Philippines | 0.06 | 0.13 | 1.73 | 3.80 | |
Qatar | 0.52 | 0.62 | 12.43 | 14.44 | |
South Korea | 0.09 | 0.18 | 2.43 | 4.29 | |
Saudi Arabia | 0.15 | 0.47 | 3.80 | 12.12 | |
Seychelles | 0.15 | 0.27 | 4.36 | 7.45 | |
Singapore | 0.13 | 0.24 | 3.53 | 5.61 | |
Sri Lanka | 0.21 | 0.09 | 5.67 | 2.45 | |
Syria | 0.19 | 0.39 | 4.84 | 9.39 | |
Taiwan | 0.10 | 0.46 | 2.70 | 11.34 | |
Tajikistan | 0.30 | 0.29 | 7.99 | 7.88 | |
Thailand | 0.05 | 0.15 | 1.41 | 4.36 | |
Timor-Leste | 0.01 | 0.03 | 0.39 | 0.88 | |
Turkey | 0.36 | 0.53 | 9.46 | 13.67 | |
Turkmenistan | 0.21 | 0.70 | 5.97 | 20.23 | |
United Arab Emirates | 0.34 | 0.79 | 8.99 | 17.66 | |
Uzbekistan | 0.17 | 0.33 | 4.99 | 9.08 | |
Viet Nam | 0.01 | 0.03 | 0.20 | 0.79 | |
Yemen | 0.06 | 0.14 | 1.60 | 3.63 |
ASMR, age standardized mortality rate; ASDR, age standardized disability-adjusted life year rate.
Through preliminary analyses and joinpoint analyses, we found that ASMR and ASPR showed a significant upward trend in both, female, and male subgroups (Figure 6A-6D). In 1990, the absolute number of KC mortalities and DALYs attributed to HBMI first increased and then decreased with age, with the peak of the absolute number of male mortalities occurring at 60–64 years old, the peak of the absolute number of female mortalities occurring at 65–69 years old, the peak of the absolute number of male DALYs occurring at 55–59 years old, and the peak of the absolute number of female DALYs occurring at 60–64 years old (Figure 6E-6H). In 2021, the absolute number of KC mortalities attributed to HBMI increased with age, the absolute number of DALYs first increased and then decreased with age, with the peak of the absolute number of male DALYs occurring at 55–59 years old, and the peak of the absolute number of female DALYs occurring at 65–69 years old. In both 1990 and 2021, MR and DR increased significantly with age in both sexes (Figure 6I-6L) (Tables S5,S6). Finally, it was predicted that both ASMR and ASDR attributed to HBMI would increase significantly in the next 15 years (Figure S6A,S6F).
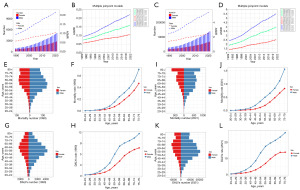
We conducted age-period-cohort model analyses on the RR of KC mortality attributed to HBMI. After adjusting for period and birth cohort, the RR of KC mortality increased with age. After adjusting for age and birth cohort, the RR of KC mortality increased with period. After adjusting for age and period, the RR of KC mortality decreased with birth cohort (Figure S7A-S7C).
Health inequalities analyses were conducted subsequently. The Lorenz curve and the CIs of two years (1990 and 2021) both indicated that the disease burden of the ASMR and ASDR attributed to HBMI was concentrated in relatively wealthy countries, and the disease burden of KC in wealthy countries increased slightly in 2021 (Figure 7A,7B). ASMR and ASDR attributed to HBMI were all positively correlated with SDI (Figure 7C,7D).
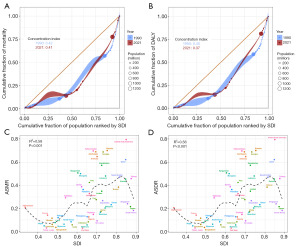
In the frontier analyses of ASMR attributed to HBMI and ASDR attributed to HBMI based on KC in Asian countries and regions from 1990 to 2021, with the increase of SDI, ASMR and ASDR showed an upward trend and were further away from the frontier (Figure S8A,S8B). The results of the frontier analyses in 2021 suggested that in terms of ASMR, ten countries such as Georgia, Bahrain and the United Arab Emirates had significantly higher ASMR and were far from the frontier. In contrast, the ASMR of countries such as Timor-Leste, Viet Nam and Bangladesh were closer to the frontier (Figure S8C). When evaluating ASDR, ten countries such as Turkmenistan, Georgia and Azerbaijan had significantly higher ASDR and were far from the frontier. In contrast, the ASDR of countries such as Timor-Leste, Viet Nam and Bangladesh were closer to the frontier (Figure S8D).
Discussion
We conducted comprehensive analyses of the KC disease burden and risk factors (HBMI) in Asia. The KC burden has increased significantly in Asia. The SDI is significantly positively correlated with ASIR, ASPR, ASMR and ASDR. Among all risk factors, the KC burden attributed to HBMI (ASMR and ASDR) is still increasing and also significantly positively correlated with SDI. The rising burden of KC in high-SDI countries can be attributed to overweight, smoking and hypertension (26). In addition, countries and regions with high SDI have an increased probability of incidentally discovering renal structural abnormalities during abdominal imaging examinations or routine physical examinations for other diseases due to increased health awareness among the population, widespread use of advanced diagnostic technologies (even small renal masses can be identified), and favorable economic conditions. This phenomenon of overdiagnosis has led to an increase in the burden of KC in countries and regions with high SDI (27-29).
Compared with the more economically developed Europe and North America, the burden of KC in Asian countries and regions is relatively low, but it has increased rapidly in recent years. The main reason could be that with the development of the Asian economy and the improvement of diagnostic techniques, the detection rate of KC has increased (7). In addition, lifestyle and diet in Asian countries has shown a westernization trend with increased calorie intake and lack of adequate exercise, which could increase HBMI and promote the incidence and mortality of KC (3,30). An increasingly aged population may also contribute to KC incidence (31,32). Among Asian countries and regions, Mongolia had the fastest growth in ASIR, ASPR, ASMR, and ASDR from 1990 to 2021. Taiwan had the fastest growth in ASMR and ASDR attributed to HBMI. These results suggest that KC might become one of the major public health problems in economically underdeveloped countries and regions that had a lower disease burden previously.
We also find that in Asia, the KC burden in males is much heavier than that in females. Genetics, hypertension, obesity, lifestyle, and sex hormones all contribute to sex differences in the burden of KC. From a genetic point of view, escape from X-chromosome linked inactivation and Y-chromosome loss can lead to a higher burden of KC in males than in females (33). Hypertension is independently associated with the risk of KC, with a higher incidence of hypertension in males than in females (34,35). Male hypertensive patients are 1.32 times more likely to develop KC (36). Although the use of antihypertensive medications is significantly associated with an increased risk of KC, it is not associated with gender subgroups (37). Males tend to develop visceral fat, which resulted in a significantly higher BMI than females which has been shown to be a risk factor for various cancers (38-41). But obese premenopausal females having an increased risk of KC (odds ratio 1.67) (42). Smoking is an important risk factor for the KC burden, and it significantly increases the risk of KC incidence and disease-specific mortality (43). The smoking rate among Asian males was significantly higher than that among females, and the sex difference in the smoking rate would exacerbate the difference in KC burden between males and females (44). Although the KC burden caused by smoking did not change significantly from 1990 to 2021 in the analyses of attributable risk factors, it has remained at a high level. And a study pointed that the sex differences in KC decreases with age, suggesting that female hormones may play an important role (45). Estrogen and progesterone have a protective effect on renal blood flow, glomerular filtration rate, and nephron ion and water reabsorption processes, while testosterone tends to impair these functions (46). Therefore, the biological effects of estrogen are the most important factors in enhancing female renal function and preventing renal disease. For both males and females, a peak in KC burden occurred in the <5 years stage (1990), which was attributed to Wilms tumor (the most common KC in children). By 2021, the KC burden in the <5 years stage had decreased significantly, which was closely related to the improvement of medical technology.
The results of the age-period-cohort model were generally consistent. The RR of the age effect was generally on an upward trend. DNA damage caused by aging and the production of inflammatory cytokines could promote the occurrence and progression of tumors (47-49). The period effect had a significant increase in the disease burden of KC, which might be related to changes in dietary patterns and the increase in the obese population. HBMI could significantly increase the risk of KC and is an independent prognostic factor for KC (34,50,51). The cohort effect represented the impact of socioeconomic, behavioral and environmental factors on the risk of KC. The RR of the cohort effect was generally on a continuous downward trend. This decline was the result of socioeconomic and medical development. In addition, the health awareness of the younger generation has improved, and they paid more attention to a reasonable diet and appropriate exercise, which was also an important reason why the KC burden attributed to HBMI has decreased with the birth cohort.
Frontier analyses showed that the KC burden has tilted more heavily toward developed countries and regions between 1990 and 2021, and had a clear positive correlation with SDI. The 2021 frontier analyses results showed that the KC burden in developed countries and regions was far from the optimal frontier, and there was room for improvement. Developing countries were in the leading position in dealing with the KC burden, which could serve as a reference for developed countries to formulate health care policies.
This study provided comprehensive assessments of the KC burden in Asia, offering valuable insights for health policy makers. The burden of KC in Asia is increasing, and we predict that it will continue to increase in the future. Several autosomal dominant tumor syndromes predispose patients to KC, including von Hippel-Lindau syndrome, hereditary leiomyoma and RCC syndrome, hereditary papillary RCC and Birt-Hogg-Dube syndrome, which are caused by germline mutations in von Hippel-Lindau tumor suppressor (VHL), fumarate hydratase (FH), MET proto-oncogene, receptor tyrosine kinase (MET), and folliculin (FLCN), respectively. Patients with germline mutations in BRCA1 associated deubiquitinase 1 (BAP1), succinate dehydrogenase complex iron sulfur subunit B (SDHB), succinate dehydrogenase complex subunit C (SDHC), succinate dehydrogenase complex subunit D (SDHD), TSC complex subunit 1 (TSC1), TSC complex subunit 2 (TSC2), and melanocyte inducing transcription factor (MITF) are also at increased risk for KC (6). In Asian countries and regions where the burden of KC has increased significantly, the characteristics of these KC-related genes can be investigated to clarify the mechanisms of KC growth. Compared with genetic investigations, interventions on modifiable risk factors are also an effective means of preventing KC. In our study results, the risk factor HBMI deserves further attention. Asian countries and regions with high SDI have a higher burden of KC caused by HBMI, and the burden of KC in males is greater than that in females. Therefore, it is crucial to prioritize additional education on healthy lifestyles and diets for males in all Asian countries, aiming to reduce the burden in regions and countries with higher SDI and curb the growth of the burden in less developed regions and countries.
However, there are several limitations in this study that need to be discussed. Firstly, due to the diversity of data sources, there might be differences between assessments of KC burden in the GBD database and the actual situation. Due to various objective limitations, it was difficult to collect health data in countries with low SDI values, which could affect the accuracy and completeness of GBD data. Secondly, some grouping information of GBD is missing. Due to the diversity of history, religion and political system in Asia, there are significant socio-cultural differences (such as diet, customs and economic development level) between countries and regions. Subgroup analyses based on the similarities and differences in social culture may obtain more meaningful results. There are numerous histological types in KC (e.g., clear cell carcinoma, chromophobe cell carcinoma and chromophilic cell carcinoma), and these different subtypes can also affect the tumor burden of KC. It is also meaningful to conduct subgroup analysis of histological classification in specific regions. Finally, due to the fact that the current estimates in the GBD database were calculated based on past trends and covariates, there is a lag. The future GBD database should make corresponding improvements to address these limitations, which is beneficial for the development of targeted intervention measures and the adjustment of healthcare policies in Asia.
Conclusions
After comprehensive analyses, the burden of KC in Asia is on the rise and was significantly positively correlated with SDI. The main attributable risk factor, HBMI, should be given further attention. Investigation of genetic characteristics in Asian countries and regions with high incidence of KC and additional interventions in healthy lifestyle and diet for Asian men will be effective means to curb the continued growth of KC burden.
Acknowledgments
None.
Footnote
Reporting Checklist: The authors have completed the STROBE reporting checklist. Available at https://tau.amegroups.com/article/view/10.21037/tau-2024-652/rc
Peer Review File: Available at https://tau.amegroups.com/article/view/10.21037/tau-2024-652/prf
Funding: This project was supported by
Conflicts of Interest: All authors have completed the ICMJE uniform disclosure form (available at https://tau.amegroups.com/article/view/10.21037/tau-2024-652/coif). The authors have no conflicts of interest to declare.
Ethical Statement:
Open Access Statement: This is an Open Access article distributed in accordance with the Creative Commons Attribution-NonCommercial-NoDerivs 4.0 International License (CC BY-NC-ND 4.0), which permits the non-commercial replication and distribution of the article with the strict proviso that no changes or edits are made and the original work is properly cited (including links to both the formal publication through the relevant DOI and the license). See: https://creativecommons.org/licenses/by-nc-nd/4.0/.
References
- Safiri S, Hassanzadeh K, Ghaffari Jolfayi A, et al. Kidney cancer in the Middle East and North Africa region: a 30-year analysis (1990-2019). Sci Rep 2024;14:13710. [Crossref] [PubMed]
- Cirillo L, Innocenti S, Becherucci F. Global epidemiology of kidney cancer. Nephrol Dial Transplant 2024;39:920-8. [Crossref] [PubMed]
- Scelo G, Larose TL. Epidemiology and Risk Factors for Kidney Cancer. J Clin Oncol 2018; Epub ahead of print. [Crossref] [PubMed]
- Capitanio U, Montorsi F. Renal cancer. Lancet 2016;387:894-906. [Crossref] [PubMed]
- Levi F, Ferlay J, Galeone C, et al. The changing pattern of kidney cancer incidence and mortality in Europe. BJU Int 2008;101:949-58. [Crossref] [PubMed]
- Bukavina L, Bensalah K, Bray F, et al. Epidemiology of Renal Cell Carcinoma: 2022 Update. Eur Urol 2022;82:529-42. [Crossref] [PubMed]
- Znaor A, Lortet-Tieulent J, Laversanne M, et al. International variations and trends in renal cell carcinoma incidence and mortality. Eur Urol 2015;67:519-30. [Crossref] [PubMed]
- Bai X, Yi M, Dong B, et al. The global, regional, and national burden of kidney cancer and attributable risk factor analysis from 1990 to 2017. Exp Hematol Oncol 2020;9:27. [Crossref] [PubMed]
- Zi H, He SH, Leng XY, et al. Global, regional, and national burden of kidney, bladder, and prostate cancers and their attributable risk factors, 1990-2019. Mil Med Res 2021;8:60. [Crossref] [PubMed]
- Huang Q, Zi H, Luo L, et al. Secular trends of morbidity and mortality of prostate, bladder, and kidney cancers in China, 1990 to 2019 and their predictions to 2030. BMC Cancer 2022;22:1164. [Crossref] [PubMed]
- Zhang L, Li Y, Ren N, et al. Incidence trends of kidney cancer in China from 1990 to 2019: A joinpoint and age-period-cohort analysis. Clin Nephrol 2024;101:181-90. [Crossref] [PubMed]
- Global incidence, prevalence, years lived with disability (YLDs), disability-adjusted life-years (DALYs), and healthy life expectancy (HALE) for 371 diseases and injuries in 204 countries and territories and 811 subnational locations, 1990-2021: a systematic analysis for the Global Burden of Disease Study 2021. Lancet 2024;403:2133-61. [Crossref] [PubMed]
- Global burden of 288 causes of death and life expectancy decomposition in 204 countries and territories and 811 subnational locations, 1990-2021: a systematic analysis for the Global Burden of Disease Study 2021. Lancet 2024;403:2100-32. [Crossref] [PubMed]
- Global burden and strength of evidence for 88 risk factors in 204 countries and 811 subnational locations, 1990-2021: a systematic analysis for the Global Burden of Disease Study 2021. Lancet 2024;403:2162-203. [Crossref] [PubMed]
- Cao Y, Lu H, Duan P, et al. Global, regional, and national burdens of interpersonal violence in young women aged 10-24 years from 1990 to 2019: a trend analysis based on the global burden of disease study 2019. Front Psychol 2023;14:1241862. [Crossref] [PubMed]
- Shu Y, Wu Z, Yang X, et al. The burden of epilepsy in the People's Republic of China from 1990 to 2019: epidemiological trends and comparison with the global burden of epilepsy. Front Neurol 2023;14:1303531. [Crossref] [PubMed]
- Tang L, Xie L, Liu Y. Temporal trends of ischemic stroke attributable to high fasting plasma glucose in China from the global burden of disease study 2019. Front Endocrinol (Lausanne) 2024;15:1408691. [Crossref] [PubMed]
- Gao Y, Liu K, Fang S. Trend analysis of stroke subtypes mortality attributable to high body-mass index in China from 1990 to 2019. BMC Public Health 2024;24:2155. [Crossref] [PubMed]
- Global pattern, trend, and cross-country inequality of early musculoskeletal disorders from 1990 to 2019, with projection from 2020 to 2050. Med 2024;5:943-962.e6. [Crossref] [PubMed]
- Yang X, Liu C, Liu Y, et al. The global burden, trends, and inequalities of individuals with developmental and intellectual disabilities attributable to iodine deficiency from 1990 to 2019 and its prediction up to 2030. Front Nutr 2024;11:1366525. [Crossref] [PubMed]
- Ordunez P, Martinez R, Soliz P, et al. Rheumatic heart disease burden, trends, and inequalities in the Americas, 1990-2017: a population-based study. Lancet Glob Health 2019;7:e1388-97. [Crossref] [PubMed]
- Pan H, Zhao Z, Deng Y, et al. The global, regional, and national early-onset colorectal cancer burden and trends from 1990 to 2019: results from the Global Burden of Disease Study 2019. BMC Public Health 2022;22:1896. [Crossref] [PubMed]
- Ruan R, Liu X, Zhang Y, et al. Global, Regional, and National Advances Toward the Management of Rheumatic Heart Disease Based on the Global Burden of Disease Study 2019. J Am Heart Assoc 2023;12:e028921. [Crossref] [PubMed]
- Wang F, Ma B, Ma Q, et al. Global, regional, and national burden of inguinal, femoral, and abdominal hernias: a systematic analysis of prevalence, incidence, deaths, and DALYs with projections to 2030. Int J Surg 2024;110:1951-67. [Crossref] [PubMed]
- Zhang C, Liu Y, Zhao H, et al. Global Patterns and Trends in Total Burden of Hepatitis B from 1990 to 2019 and Predictions to 2030. Clin Epidemiol 2022;14:1519-33. [Crossref] [PubMed]
- Arabsalmani M, Mohammadian-Hafshejani A, Ghoncheh M, et al. Incidence and mortality of kidney cancers, and human development index in Asia; a matter of concern. J Nephropathol 2017;6:30-42. [Crossref] [PubMed]
- Patel AR, Prasad SM, Shih YC, et al. The association of the human development index with global kidney cancer incidence and mortality. J Urol 2012;187:1978-83. [Crossref] [PubMed]
- Gupta K, Miller JD, Li JZ, et al. Epidemiologic and socioeconomic burden of metastatic renal cell carcinoma (mRCC): a literature review. Cancer Treat Rev 2008;34:193-205. [Crossref] [PubMed]
- Ayubi E, Shahbazi F, Khazaei S. Decomposing difference in the kidney cancer burden measures between 1990 and 2019 based on the global burden of disease study. Sci Rep 2024;14:10390. [Crossref] [PubMed]
- Chow WH, Dong LM, Devesa SS. Epidemiology and risk factors for kidney cancer. Nat Rev Urol 2010;7:245-57. [Crossref] [PubMed]
- Liu Z, Jiang Y, Fang Q, et al. Future of cancer incidence in Shanghai, China: Predicting the burden upon the ageing population. Cancer Epidemiol 2019;60:8-15. [Crossref] [PubMed]
- Yeung WJ, Lee Y. Aging in East Asia: New Findings on Retirement, Health, and Well-Being. J Gerontol B Psychol Sci Soc Sci 2022;77:589-91. [Crossref] [PubMed]
- Pallauf M, Ged Y, Singla N. Molecular differences in renal cell carcinoma between males and females. World J Urol 2023;41:1727-39. [Crossref] [PubMed]
- Macleod LC, Hotaling JM, Wright JL, et al. Risk factors for renal cell carcinoma in the VITAL study. J Urol 2013;190:1657-61. [Crossref] [PubMed]
- Doumas M, Papademetriou V, Faselis C, et al. Gender differences in hypertension: myths and reality. Curr Hypertens Rep 2013;15:321-30. [Crossref] [PubMed]
- Gelfond J, Al-Bayati O, Kabra A, et al. Modifiable risk factors to reduce renal cell carcinoma incidence: Insight from the PLCO trial. Urol Oncol 2018;36:340.e1-6. [Crossref] [PubMed]
- Peired AJ, Campi R, Angelotti ML, et al. Sex and Gender Differences in Kidney Cancer: Clinical and Experimental Evidence. Cancers (Basel) 2021;13:4588. [Crossref] [PubMed]
- Pou KM, Massaro JM, Hoffmann U, et al. Visceral and subcutaneous adipose tissue volumes are cross-sectionally related to markers of inflammation and oxidative stress: the Framingham Heart Study. Circulation 2007;116:1234-41. [Crossref] [PubMed]
- Tahergorabi Z, Khazaei M, Moodi M, et al. From obesity to cancer: a review on proposed mechanisms. Cell Biochem Funct 2016;34:533-45. [Crossref] [PubMed]
- O'Rourke RW. Obesity and cancer: at the crossroads of cellular metabolism and proliferation. Surg Obes Relat Dis 2014;10:1208-19. [Crossref] [PubMed]
- Iyengar NM, Gucalp A, Dannenberg AJ, et al. Obesity and Cancer Mechanisms: Tumor Microenvironment and Inflammation. J Clin Oncol 2016;34:4270-6. [Crossref] [PubMed]
- Sun L, Chao F, Luo B, et al. Impact of Estrogen on the Relationship Between Obesity and Renal Cell Carcinoma Risk in Women. EBioMedicine 2018;34:108-12. [Crossref] [PubMed]
- Cumberbatch MG, Rota M, Catto JW, et al. The Role of Tobacco Smoke in Bladder and Kidney Carcinogenesis: A Comparison of Exposures and Meta-analysis of Incidence and Mortality Risks. Eur Urol 2016;70:458-66. [Crossref] [PubMed]
- Luo LS, Luan HH, Zhang P, et al. The disease burden of bladder cancer and its attributable risk factors in five Eastern Asian countries, 1990-2019: a population-based comparative study. BMC Public Health 2024;24:2404. [Crossref] [PubMed]
- Hew MN, Zonneveld R, Kümmerlin IP, et al. Age and gender related differences in renal cell carcinoma in a European cohort. J Urol 2012;188:33-8. [Crossref] [PubMed]
- Harvey BJ, Alvarez de la Rosa D. Sex Differences in Kidney Health and Disease. Nephron 2025;149:77-103. [PubMed]
- Faget DV, Ren Q, Stewart SA. Unmasking senescence: context-dependent effects of SASP in cancer. Nat Rev Cancer 2019;19:439-53. [Crossref] [PubMed]
- Lu T, Pan Y, Kao SY, et al. Gene regulation and DNA damage in the ageing human brain. Nature 2004;429:883-91. [Crossref] [PubMed]
- Conley KE, Jubrias SA, Esselman PC. Oxidative capacity and ageing in human muscle. J Physiol 2000;526:203-10. [Crossref] [PubMed]
- Bergström A, Hsieh CC, Lindblad P, et al. Obesity and renal cell cancer--a quantitative review. Br J Cancer 2001;85:984-90. [Crossref] [PubMed]
- Nam GE, Cho KH, Han K, et al. Obesity, abdominal obesity and subsequent risk of kidney cancer: a cohort study of 23.3 million East Asians. Br J Cancer 2019;121:271-7. [Crossref] [PubMed]