Weekly ascorbic acid infusion in castration-resistant prostate cancer patients: a single-arm phase II trial
Introduction
The use of complementary and alternative medicine (CAM) is becoming increasingly popular for the treatment of patients diagnosed with cancer. Thirty percent of prostate cancer (PC) patients are treated with at least one CAM method (1). Metastatic castration-resistant prostate cancer (mCRPC) is the end stage in a sequence of PC disease landmarks. The initial phase of mCRPC is asymptomatic, with progression only being detectable by biomarkers and imaging techniques. Following the asymptomatic window, a period of increased mortality is observed, with a median survival of 2–3 years.
Treatment with intravenous (IV) ascorbic acid (AA) is widely used in CAM clinics (2), but quality evidence regarding the efficacy and safety of this treatment is lacking. Indeed, two randomized controlled trials (3,4) of oral AA for the treatment of advanced cancers were not able to reproduce the improved overall survival (OS) that was observed in a large case series reported in the 1970s (5,6).
AA shows in vitro cytostatic and cytotoxic properties in tumor cell lines, including the NCI60 screening panel, and to have some in vivo cytostatic and cytotoxic properties (7,8). The mechanisms underlying these properties of AA have not been fully elucidated, and it remains unclear whether the effect of AA on tumor level, if any, is able to stabilize the disease or cause remission. The cytotoxic concentrations of AA are more than 100-fold higher than those obtainable by oral administration and can only be achieved by IV administration (9), which was not used in the randomized controlled trials (3,4). The average IV AA dose used in CAM for various indications, including cancer, is 28 g every 4 days (2).
The present study investigated the efficacy and safety of weekly high-dose IV AA in mCRPC patients using standardized clinical trial principles (10,11).
Methods
Study design
The study was designed as a non-comparative, single-center, phase II trial. Patients were recruited from the outpatient urology clinic at Copenhagen University Hospital Herlev. The study was initially designed with 20-g infusions, but the dose was increased after the enrollment of eight participants (unpublished data), as the predefined plasma concentration range was not obtained with 20-g infusions. All patients continued their normally scheduled visits to ensure the best standard of care. If patients were referred to chemotherapy, their participation in the present trial was discontinued.
Patient eligibility
The eligibility criteria included adenocarcinoma of the prostate with at least one metastatic lesion visible by bone or CT scan; ongoing androgen-deprivation therapy with castration-level testosterone (<1.7 nmol/L); disease progression [indicated by prostate-specific antigen (PSA) level or imaging findings] as defined by the Prostate Cancer Clinical Trials Working Group (PCWG-2) (10), no prior chemotherapy; and Eastern Cooperative Oncology Group (ECOG) performance of 0–2. The key exclusion criteria were surgical removal or radiation of the prostate; significant renal impairment; significant cardiac disease; history of oxalate renal stones; hemochromatosis; and glucose-6-phosphate dehydrogenase deficiency.
All patients signed informed consent forms. The trial was approved by the Regional Ethics Committee (H-C-2009-018), the Danish Health and Medicines Authority (2612-3978), and the Danish Data Protection Agency (2007-58-0015/750.19-15). It was registered (Eudra-CT 2008-008692-33/NCT01080352) and followed the current Guidelines for Good Clinical Practice and the Declaration of Helsinki.
Intervention
Patients received one weekly infusion of AA, as previously described (12). In brief, the initial dose was 5 g, followed by 30 g in week 2 and a final dose of 60 g in week 3. The infusion rate was 1 g/min. The trial target dose of 60 g was continued once per week until the initial efficacy evaluation at 12 weeks. Patients were also given a daily oral dose of 500 mg AA from the first infusion, and it was continued for 26 weeks to avoid a hypothetical rebound deficiency following infusion (13).
Statistical considerations
Data were analyzed using R v.3.0.3. Data are presented as medians along with a Q1–Q3 interquartile range. Data collected at baseline and at week 12 were compared using a predefined paired non-parametric statistical test due to the study design and the small sample size. A P value <0.05 was considered statistically significant. The number of participants needed to achieve sufficient statistical power was calculated based on the primary efficacy marker using a slightly modified Ahern’s study design (14). The minimum response was determined based on TAX327-controls (15). p0 was estimated as 5%, p1 =30%, α =0.05 and power =80%. Further investigations were warranted if ≥3 of the 20 patients met the primary endpoint for efficacy.
Trial evaluation (efficacy and safety)
Drug
Plasma AA concentrations were measured at the end of the infusion and 30 minutes after the end of the infusion on weeks 1–6, 9, and 12. The predefined goal for plasma AA concentration was >5–10 mM. The analytical procedures have been described previously (16).
Efficacy
The primary endpoint for efficacy was binary and was defined as ≥50% PSA reduction from baseline to 12 weeks. If achieved, an optional 8-week extension of the AA treatment was offered. The following additional biomarkers from the Halabi (17) and Smaletz (18) prognostic model were collected as markers of potential efficacy: hemoglobin (Hgb), lactate dehydrogenase (LDH), alkaline phosphatase (ALP), and albumin levels. All venous blood samples were subjected to standard accredited analysis at the Department of Clinical Biochemistry, Herlev University Hospital immediately after sampling. Biomarkers of bone metabolism were included to provide information on turnover rate, including two markers of bone formation and one marker of bone resorption: bone-specific alkaline phosphatase (bALP; an isoform of ALP), pro-collagen type I N-terminal (PINP), and urinary type I collagen cross-linked N telopeptides (uNTx). bALP was measured in venous serum blood samples, and uNTx was measured in spot urine samples (second morning void), normalized to urine creatinine, and analyzed at the Department of Clinical Chemistry, Aarhus University Hospital. PINP was measured in venous serum blood samples at the Department of Clinical Chemistry, Copenhagen University Hospital Glostrup.
Metastatic burden in bone was evaluated by 99 m-Tc bisphosphonate bone scans at weeks 0, 12, 26, and 52. Bone scans were performed as routine investigations at the institutional Department of Clinical Physiology and were analyzed using a computerized detection system (EXINI bone software) to minimize interpretation bias (19). A link between inflammation and cancer has been suggested by epidemiological and experimental data (20,21). To quantify the inflammatory response and the level of oxidative stress, biomarkers of both parameters were measured, namely C-reactive protein (CRP), YKL-40, 8-oxo-7, 8-dihydro-2’-deoxyguanosine (8-oxodG), and 8-oxo-guanosine (8-oxoGuo). The CRP level was analyzed at the Department of Clinical Biochemistry, Herlev University Hospital, immediately after sampling. The analytic kit was not highly sensitive; hence, concentrations below 3 mg/L were reported as “<3”. The plasma YKL-40 level was analyzed at the Department of Medicine, Copenhagen University Hospital Herlev. Markers of oxidative stress-induced DNA (8-oxodG) and RNA (8-oxoGuo) damage were measured in urine samples collected over a 24-h period, which ended on the day of baseline sampling and on the day of the last infusion; this analysis was performed at the Laboratory of Clinical Pharmacology, Copenhagen University Hospital Rigshospitalet. Performance status was recorded at every visit using the ECOG Performance Status Scale (22). Changes in health-related quality of life (HRQoL) were assessed by the European Organization for Research and Treatment of Cancer (EORTC) self-administered questionnaires QLQ-C30 (v3.0) (23) and QLQ-PR25 (24). Scores were transformed to a 0–100 scale according to the manuals of the questionnaires with the R package “QoLR v1.0”.
Safety
Patients remained under observation by the study personnel during the infusion and for 30 min afterwards. Blood was screened for hemolysis 30 min after the infusion. Every 3 weeks, a “safety blood-chemistry profile” was drawn to examine liver toxicity, kidney function, and electrolyte and hemoglobin levels. All adverse events (AE) were scored using the Common Terminology Criteria for Adverse Events v4.03 (CTCAE) (25). Deviations from the reference values present at baseline were only classified as AEs in cases of progression by one CTCAE grade.
Long-term follow-up
Follow-up visits were planned at 20, 26 and 52 weeks after trial initiation to monitor the long-term effects of the treatment on potential responders. All of the efficacy markers were evaluated at each follow-up visit. Follow-up was discontinued if the patient was enrolled in another clinical trial or if chemotherapy was initiated.
Results
Patient characteristics
Twenty-three patients were enrolled in this study between October 2011 and November 2013. All patients were male Caucasians. Detailed baseline characteristics of all the enrolled patients are shown in Tables 1 and 2. Prior to enrollment, patients received a least one additional hormone manipulation other than androgen deprivation therapy. Three patients discontinued treatment before the initial efficacy evaluation. Details are provided in Figure 1.
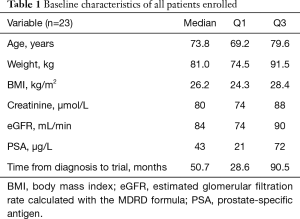
Full table
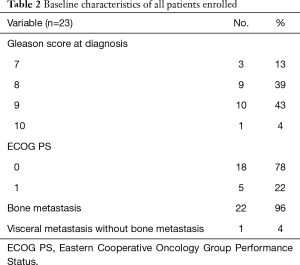
Full table
Drug evaluation
With the infusion of 60 g of AA (0.74 g/kg, Q1–Q3: 0.66–0.81), all patients reached an AA plasma concentration within the predefined range. At infusion, the mean termination plasma AA concentration was 19.3 mM, and it was 15.1 mM after an additional 30 min. The detailed pharmacokinetic data of 10 participants have been published previously (12). The median baseline AA level was 54 µM (Q1–Q3: 45.6–73.5). At week 26, when oral AA supplementation was terminated, the median plasma AA was 88.4 µM (range 45.0–146.6 µM); hence, the patients were non-deficient (8).
Efficacy evaluation
Data from all of the patients who completed the study protocol until the initial efficacy evaluation at 12 weeks are summarized in Tables 3-6. No patients achieved the primary endpoint for efficacy, i.e., a PSA reduction of 50%. Baseline-normalized percentage changes are presented in Figure 2. Fifteen patients (75%) displayed a nominal PSA increase, although three of these patients did not meet the PCWG-2 criteria for progression (>25% increase). Nine of 20 patients at week 6 and 10 of 20 patients at week 9 experienced PSA progression. Overall, PSA increased by 17 µg/L (Q1–Q3: 1–37, P=0.003). Five patients had a lower PSA at 12 weeks than at the baseline, and the maximum response (−27%) represented an absolute decrease of >2 µg/L.
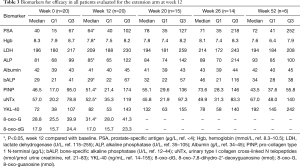
Full table
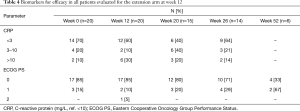
Full table
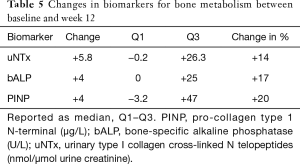
Full table

Full table
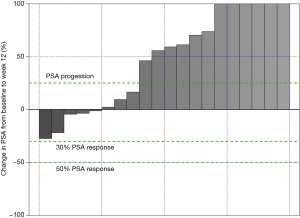
No improvement in the secondary efficacy markers was observed. The median ALP level increased (P=0.01) and the Hgb level decreased (P=0.0001) from the baseline to 12 weeks. The LDH level tended to increase and the albumin level to decrease, implying disease progression. No marker for bone turnover decreased, but minor increases of 14–20% were observed, as shown in Table 5. The following results were found for the outcomes, dichotomized as a decrease or increase from the baseline: 9 patients had no response for any bone marker, 7 patients showed a response for 1 biomarker, 2 patients showed a response for 2 biomarkers, and 2 patients showed a decrease in all 3 biomarkers. None of the patients started antiresorptive therapy during the intervention period; 2 patients received 120 mg/4 weeks denosumab prior to and during the trial, and 6/15 patients with follow-up data started denosumab at some point during the follow-up period.
Markers of inflammation and oxidative stress did not change from the baseline, except for a minor increase in 8-oxodG (DNA damage) (P=0.03). The dichotomized CRP response with a normal range cut-off (>10) did not differ from the baseline to 12 weeks by McNemar’s test.
Three patients (19%) showed reductions in BSI and the number of lesions at 12 weeks compared to the baseline; all had one fewer high-probability lesion. Details by week are shown in Table 6. For technical reasons, 3 bone scans were missing from the analysis at baseline, and 1 bone scan was missing at weeks 12 and 26, leaving only 16 paired datasets for evaluation.
HRQoL and ECOG performance status
Sixteen patients (80%) had an unchanged ECOG score at week 12; 2 patients had an improved score, and 2 had a worse score, with a drop of 1–2 steps. Table 7 shows the HRQoL data. Only 1 scale, physical functioning, differed from baseline to week 12, and no trends of improvement were seen for the other scales.
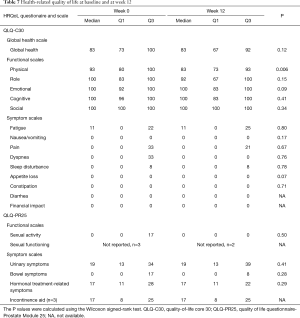
Full table
Safety
A total of 53 AEs were recorded in the trial; 5/23 patients (21.7%) had no AEs, and 4/23 patients (17.3%) had only one AE. Detailed data are shown in Table 8. Eleven of the AEs were recorded as serious due to hospitalization, although three of them were elective procedures. One patient discontinued the AA therapy due to side effects (increased lower-urinary-tract symptoms). The most frequent findings were hypertension and anemia. Two episodes of pulmonary embolism were recorded, although one episode was asymptomatic and was discovered by a pre-chemotherapy-evaluation CT scan during the post-trial follow-up.
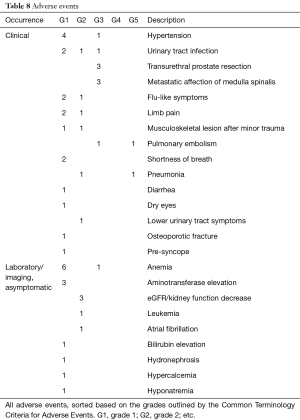
Full table
Discussion
The present study does not indicate that high-dose IV AA given once per week for 12 weeks induces tumor regression, as evaluated by a significant PSA reduction in patients with mCRPC, although the plasma concentrations of AA did reach the cytotoxic range for cancer cells. This result is in accord with all previous prospective studies, which showed no clinical signs of tumor growth inhibition (26). In the present trial, 12 (52%) of the 23 enrolled patients experienced either biochemical or clinical progression (leading to withdrawal) at 6 weeks, which is a short time to progression compared to a similar cohort studied previously (27).
The criteria for an 8-week treatment extension were based on PCWG-1 recommendations, which imply a survival benefit (11). The use of a ≥50% PSA response as the surrogacy criterion for OS has been questioned. Based on data from TAX327 (28) and SWOG99-16 (29), a ≥30% PSA reduction may improve the accuracy of the response criterion. Irrespective of whether the ≥50% or ≥30% response criterion was applied, no patient achieved a response, as the greatest observed reduction in PSA was 27%.
One of the major limitations of our study is the absence of a control group. A spontaneous 50% PSA decline in mCPRC is rare; it has been previously reported to occur in 1.3% or 3% of cases (27,30). By using PSA as a marker of treatment efficacy, two major criteria are fulfilled: the tumor must produce PSA, and the action of the investigated compound must induce changes that can be detected as changes in PSA. To be included in the present trial, the tumor had to be PSA-producing, but it remains unknown if AA is capable of changing PSA levels per se. Immunotherapy may improve OS without generating a significant PSA response. AA may enhance immune-cell activity (31), but no study has shown any cancer-directed immunotherapy using AA alone. To pursue possible effects of AA not related to PSA expression by tumor cells, additional biomarkers of the PCWG-2 and two prognostic nomograms were included (10,17,18). No clear trend of improvement was observed for any variable.
The long-term follow-up was primarily designed to identify responders and any potential lasting effects. Because no patient continued to the extension arm, they were candidates for docetaxel, which generates a selection bias for follow-up, with the “least-progressing” patients being left on-study.
Our measure of general oxidative stress-induced DNA and RNA damage was urinary excretion of oxidized nucleosides. It has previously been shown that large oral doses of AA do not reduce intracellular oxidative stress (32), and the data from the present study confirm that, even with the high plasma concentrations of AA obtained by infusion, the overall oxidative stress level did not appear to be affected.
Sufficient data were not collected for the calculation of the PSA doubling time prior to enrollment. Although the use of PSA kinetics as a surrogate endpoint has not been validated, such data could potentially provide information about the slowing of tumor progression (33). Our patient cohort had a high performance status at the baseline that remained stable during the intervention period. Compared to reference material, the QoL scores were high (34). However, the present study did not show a trend of improvement in QoL; rather, the opposite was observed. Four previous studies have investigated HRQoL using validated questionnaires, and most of them reported improvements. No study included a parallel control group or addressed selection bias or the natural course of the disease (35-38). Studies without a control group may overestimate the effect of the intervention because many cancer patients turn to CAMs to satisfy needs other than tumor treatment, including general health improvement, which can potentially contribute to an improved QoL score (39-41).
AA is highly osmotically active and may cause fluid or electrolyte disturbances. However, in the present study, treatment-induced toxicity was limited and was likely related to the vehicle rather than to AA itself. The natural course of the advancement of mCRPC explains most of the remaining recorded AEs. Cancer is known to increase the risk of thromboembolic events and may explain the two episodes of pulmonary embolism (42). However, as another trial reported pulmonary embolism in 2 of 14 patients (43), we believe that future studies should continue to examine this AE.
We utilized IV infusion of AA, which represents a pulsed delivery with a high peak concentration and rapid clearance within hours. In two recent phase I trials, even higher doses were applied, namely 1.5 g/kg and 110 g/m2, inducing peak plasma concentrations of 26 and 37 mM, respectively (37,38). Only half of the plasma concentration of AA is found in the tumor tissue and the surrounding extracellular fluids (44), and tissue hypoxia may further attenuate AA toxicity (45). In vivo studies in rodents have typically used daily intraperitoneal administration of 4 g/kg of AA, which generates a stable millimolar plasma concentration profile over time. However, despite continued exposure to presumably therapeutic concentrations of AA, tumor volume was only reduced by 22–53% in treated animals compared to controls (46-48).
Thus, if AA has antineoplastic properties, it appears that the effect of pulsed, periodic IV AA administration may be limited. Whether different AA delivery approaches possess higher therapeutic potential in humans remains to be investigated in future trials.
Conclusions
Infusion with 60 g of AA once per week did not induce disease remission in mCRPC patients; therefore, the findings of the present study do not support the use of high-dose IV AA for late-stage PC. At present, the lack of efficacy of this treatment suggests that it should not be used outside clinical trials. A large-scale controlled trial is needed to determine whether high-dose IV AA displays any therapeutic relevance.
Acknowledgements
This work was supported with unrestricted grants by “Kirsten og Freddy Johansens Fond” (private foundation) and the Capital Region of Denmark.
We would like to thank Prof. Julia S. Johansen and laboratory technicians from the Department of Medicine, Herlev University Hospital for performing the YKL40 analysis, laboratory technicians Joan Frandsen and Katja Christensen for analytical work, and research nurse Christine Humphrys for assistance in the clinical trial phase.
Footnote
Conflicts of Interest: The authors have no conflicts of interest to declare.
Ethical Statement: The trial was approved by the Regional Ethics Committee (H-C-2009-018), the Danish Health and Medicines Authority (2612-3978), and the Danish Data Protection Agency (2007-58-0015/750.19-15). It was registered (Eudra-CT 2008-008692-33/NCT01080352) and followed the current Guidelines for Good Clinical Practice and the Declaration of Helsinki. Written informed consent was obtained from the patient for publication of this manuscript and any accompanying images.
References
- Bishop FL, Rea A, Lewith H, et al. Complementary medicine use by men with prostate cancer: a systematic review of prevalence studies. Prostate Cancer Prostatic Dis 2011;14:1-13. [Crossref] [PubMed]
- Padayatty SJ, Sun AY, Chen Q, et al. Vitamin C: intravenous use by complementary and alternative medicine practitioners and adverse effects. PLoS One 2010;5:e11414. [Crossref] [PubMed]
- Creagan ET, Moertel CG, O'Fallon JR, et al. Failure of high-dose vitamin C (ascorbic acid) therapy to benefit patients with advanced cancer. A controlled trial. N Engl J Med 1979;301:687-90. [Crossref] [PubMed]
- Moertel CG, Fleming TR, Creagan ET, et al. High-dose vitamin C versus placebo in the treatment of patients with advanced cancer who have had no prior chemotherapy. A randomized double-blind comparison. N Engl J Med 1985;312:137-41. [Crossref] [PubMed]
- Cameron E, Campbell A. The orthomolecular treatment of cancer. II. Clinical trial of high-dose ascorbic acid supplements in advanced human cancer. Chem Biol Interact 1974;9:285-315. [Crossref] [PubMed]
- Cameron E, Pauling L. Supplemental ascorbate in the supportive treatment of cancer: Prolongation of survival times in terminal human cancer. Proc Natl Acad Sci U S A 1976;73:3685-9. [Crossref] [PubMed]
- National Institutes of Health, U.S. Department of Health and Human Service. Developmental Therapeutics Program 2014. Available online: https://dtp.cancer.gov/
- Du J, Cullen JJ, Buettner GR. Ascorbic acid: chemistry, biology and the treatment of cancer. Biochim Biophys Acta 2012;1826:443-57. [PubMed]
- Padayatty SJ, Sun H, Wang Y, et al. Vitamin C pharmacokinetics: implications for oral and intravenous use. Ann Intern Med 2004;140:533-7. [Crossref] [PubMed]
- Scher HI, Halabi S, Tannock I, et al. Design and end points of clinical trials for patients with progressive prostate cancer and castrate levels of testosterone: recommendations of the Prostate Cancer Clinical Trials Working Group. J Clin Oncol 2008;26:1148-59. [Crossref] [PubMed]
- Bubley GJ, Carducci M, Dahut W, et al. Eligibility and response guidelines for phase II clinical trials in androgen-independent prostate cancer: recommendations from the Prostate-Specific Antigen Working Group. J Clin Oncol 1999;17:3461-7. [Crossref] [PubMed]
- Nielsen TK, Højgaard M, Andersen JT, et al. Elimination of ascorbic acid after high-dose infusion in prostate cancer patients: a pharmacokinetic evaluation. Basic Clin Pharmacol Toxicol 2015;116:343-8. [Crossref] [PubMed]
- Tsao CS, Salimi SL. Evidence of rebound effect with ascorbic acid. Med Hypotheses 1984;13:303-10. [Crossref] [PubMed]
- A'Hern RP. Sample size tables for exact single-stage phase II designs. Stat Med 2001;20:859-66. [Crossref] [PubMed]
- Tannock IF, de Wit R, Berry WR, et al. Docetaxel plus prednisone or mitoxantrone plus prednisone for advanced prostate cancer. N Engl J Med 2004;351:1502-12. [Crossref] [PubMed]
- Lykkesfeldt J. Ascorbate and dehydroascorbic acid as reliable biomarkers of oxidative stress: analytical reproducibility and long-term stability of plasma samples subjected to acidic deproteinization. Cancer Epidemiol Biomarkers Prev 2007;16:2513-6. [Crossref] [PubMed]
- Halabi S, Small EJ, Kantoff PW, et al. Prognostic model for predicting survival in men with hormone-refractory metastatic prostate cancer. J Clin Oncol 2003;21:1232-7. [Crossref] [PubMed]
- Smaletz O, Scher HI, Small EJ, et al. Nomogram for overall survival of patients with progressive metastatic prostate cancer after castration. J Clin Oncol 2002;20:3972-82. [Crossref] [PubMed]
- Ulmert D, Kaboteh R, Fox JJ, et al. A novel automated platform for quantifying the extent of skeletal tumour involvement in prostate cancer patients using the Bone Scan Index. Eur Urol 2012;62:78-84. [Crossref] [PubMed]
- Grivennikov SI, Greten FR, Karin M. Immunity, inflammation, and cancer. Cell 2010;140:883-99. [Crossref] [PubMed]
- Grivennikov SI, Karin M. Inflammation and oncogenesis: a vicious connection. Curr Opin Genet Dev 2010;20:65-71. [Crossref] [PubMed]
- Oken MM, Creech RH, Tormey DC, et al. Toxicity and response criteria of the Eastern Cooperative Oncology Group. Am J Clin Oncol 1982;5:649-55. [Crossref] [PubMed]
- Aaronson NK, Ahmedzai S, Bergman B, et al. The European Organization for Research and Treatment of Cancer QLQ-C30: a quality-of-life instrument for use in international clinical trials in oncology. J Natl Cancer Inst 1993;85:365-76. [Crossref] [PubMed]
- van Andel G, Bottomley A, Fosså SD, et al. An international field study of the EORTC QLQ-PR25: a questionnaire for assessing the health-related quality of life of patients with prostate cancer. Eur J Cancer 2008;44:2418-24. [Crossref] [PubMed]
- National Cancer Institute, U.S. Department of Health and Human Services. Common Terminology Criteria for Adverse Events v4.0. 2010. Available online: http://evs.nci.nih.gov/ftp1/CTCAE/About.html
- Jacobs C, Hutton B, Ng T, et al. Is there a role for oral or intravenous ascorbate (vitamin C) in treating patients with cancer? A systematic review. Oncologist 2015;20:210-23. [Crossref] [PubMed]
- Beer TM, Armstrong AJ, Rathkopf DE, et al. Enzalutamide in metastatic prostate cancer before chemotherapy. N Engl J Med 2014;371:424-33. [Crossref] [PubMed]
- Armstrong AJ, Garrett-Mayer E, Ou Yang YC, et al. Prostate-specific antigen and pain surrogacy analysis in metastatic hormone-refractory prostate cancer. J Clin Oncol 2007;25:3965-70. [Crossref] [PubMed]
- Petrylak DP, Ankerst DP, Jiang CS, et al. Evaluation of prostate-specific antigen declines for surrogacy in patients treated on SWOG 99-16. J Natl Cancer Inst 2006;98:516-21. [Crossref] [PubMed]
- Kantoff PW, Higano CS, Shore ND, et al. Sipuleucel-T immunotherapy for castration-resistant prostate cancer. N Engl J Med 2010;363:411-22. [Crossref] [PubMed]
- Huijskens MJ, Walczak M, Sarkar S, et al. Ascorbic acid promotes proliferation of natural killer cell populations in culture systems applicable for natural killer cell therapy. Cytotherapy 2015;17:613-20. [Crossref] [PubMed]
- Porkkala-Sarataho E, Salonen JT, Nyyssönen K, et al. Long-term effects of vitamin E, vitamin C, and combined supplementation on urinary 7-hydro-8-oxo-2'-deoxyguanosine, serum cholesterol oxidation products, and oxidation resistance of lipids in nondepleted men. Arterioscler Thromb Vasc Biol 2000;20:2087-93. [Crossref] [PubMed]
- Colloca G. Prostate-specific antigen kinetics as a surrogate endpoint in clinical trials of metastatic castration-resistant prostate cancer: a review. Cancer Treat Rev 2012;38:1020-6. [Crossref] [PubMed]
- Scott N, Fayers P, Aaronson NK, et al. EORTC QLQ-C30 reference values. 2008. Available online: http://groups.eortc.be/qol/sites/default/files/img/newsletter/reference_values_manual2008.pdf
- Yeom CH, Jung GC, Song KJ. Changes of terminal cancer patients’ health-related quality of life after high dose vitamin C administration. J Korean Med Sci 2007;22:7-11. [Crossref] [PubMed]
- Takahashi H, Mizuno H, Yanagisawa A. High-dose intravenous vitamin C improves quality of life in cancer patients. Per Med Universe 2012;1:49-53. [Crossref]
- Stephenson CM, Levin RD, Spector T, et al. Phase I clinical trial to evaluate the safety, tolerability, and pharmacokinetics of high-dose intravenous ascorbic acid in patients with advanced cancer. Cancer Chemother Pharmacol 2013;72:139-46. [Crossref] [PubMed]
- Hoffer LJ, Levine M, Assouline S, et al. Phase I clinical trial of i.v. ascorbic acid in advanced malignancy. Ann Oncol 2008;19:1969-74. [Crossref] [PubMed]
- Miller M, Boyer MJ, Butow PN, et al. The use of unproven methods of treatment by cancer patients. Frequency, expectations and cost. Support Care Cancer 1998;6:337-47. [Crossref] [PubMed]
- Zachariae R, Johannessen H. A Methodological Framework for Evaluating the Evidence for Complementary and Alternative Medicine (CAM) for Cancer. Cancers (Basel) 2011;3:773-88. [Crossref] [PubMed]
- Bonacchi A, Toccafondi A, Mambrini A, et al. Complementary needs behind complementary therapies in cancer patients. Psychooncology 2015;24:1124-30. [Crossref] [PubMed]
- Lee AY, Levine MN. Venous thromboembolism and cancer: risks and outcomes. Circulation 2003;107:I17-21. [Crossref] [PubMed]
- Monti DA, Mitchell E, Bazzan AJ, et al. Phase I evaluation of intravenous ascorbic acid in combination with gemcitabine and erlotinib in patients with metastatic pancreatic cancer. PLoS One 2012;7:e29794. [Crossref] [PubMed]
- Kuiper C, Vissers MC, Hicks KO. Pharmacokinetic modeling of ascorbate diffusion through normal and tumor tissue. Free Radic Biol Med 2014;77:340-52. [Crossref] [PubMed]
- Sinnberg T, Noor S, Venturelli S, et al. The ROS-induced cytotoxicity of ascorbate is attenuated by hypoxia and HIF-1alpha in the NCI60 cancer cell lines. J Cell Mol Med 2014;18:530-41. [Crossref] [PubMed]
- Pollard HB, Levine MA, Eidelman O, et al. Pharmacological ascorbic acid suppresses syngeneic tumor growth and metastases in hormone-refractory prostate cancer. In Vivo 2010;24:249-55. [PubMed]
- Chen Q, Espey MG, Sun AY, et al. Pharmacologic doses of ascorbate act as a prooxidant and decrease growth of aggressive tumor xenografts in mice. Proc Natl Acad Sci U S A 2008;105:11105-9. [Crossref] [PubMed]
- Verrax J, Calderon PB. Pharmacologic concentrations of ascorbate are achieved by parenteral administration and exhibit antitumoral effects. Free Radic Biol Med 2009;47:32-40. [Crossref] [PubMed]