Intensity-modulated radiotherapy for prostate cancer
Introduction
Prostate cancer is the most common malignancy in men (1). Patients with localized prostate cancer have multiple treatment options including active surveillance, prostatectomy, brachytherapy, and external beam radiation therapy (EBRT). A patient’s preference toward a specific treatment is often driven by the invasiveness of the treatment modality and the expected side effects from that particular intervention. EBRT, considered as one of the least invasive treatments, has changed drastically over the past several decades with the ultimate goal of improving survival outcomes with decreasing toxicity. Older techniques were replaced with 3-dimensional (3D) conformal radiation therapy (CRT), and further technological advancements in radiation imaging, planning, and delivery has allowed the introduction and wide adoption of intensity-modulated radiation therapy (IMRT). IMRT uses 3D imaging to guide beams of radiation to the tumor from many different angles while changing (modulating) the intensity and shape of the beam to match the shape of the tumor. These adjustments of the radiation allow for the prescribed amount of radiation to be delivered to each part of the tumor while minimizing the amount of radiation normal surrounding healthy tissue receives. For prostate cancer, multiple randomized and nonrandomized series have shown improved tumor control with the use of dose escalated therapy (2-7). The enhanced conformality of IMRT allow for dose escalation to the prostate while reducing dose to the bladder and rectum, and trials have demonstrated reduced toxicity with IMRT (8,9). In addition to the fundamental principles of the IMRT technique, there are numerous biologic, anatomic, and clinical features which also make prostate cancer a model site for implementation of IMRT. In this review, we describe the evolution of EBRT to IMRT, describe the specific features of prostate cancer which make this disease an ideal site for implementation and routine use of IMRT, comparisons between IMRT and 3D-CRT including genitourinary (GU) and gastrointestinal (GI) toxicities, clinical outcomes of IMRT including dose escalation, and future directions utilizing this technology.
Evolution of external beam radiation to IMRT
Historically, definitive radiation for prostate cancer was accomplished using 2-dimensional (2D) or 3D-CRT. The most common conventional beam arrangement was a “four field box” arrangement consisting of a pair of anterior-posterior and posterior-anterior (AP-PA) beams, as well as a pair of lateral opposed beams. This approach was commonly used into the early 1990s, until 3D-CRT became more widely adopted. 3D-CRT takes advantage of sophisticated computer software, and included computed tomography (CT) simulation to integrate volumetric data regarding the patient’s tumor and organs at risk to allow for beam angles and radiation portals that were difficult to implement using conventional 2-dimensional planning.
Dearnaley et al. conducted a randomized study of conventional vs. 3D-CRT to a dose of 64 Gy in 2 Gy fractions, which at that time was a standard dose for prostate cancer (10). Of the 225 men treated, significantly fewer men developed radiation proctitis and bleeding in the 3D-CRT group compared to the conventional group (5% vs. 15% grade 2+) and there was no difference in tumor control. As toxicity was reduced with 3D-CRT, several groups conducted studies to escalate the radiation dose to the prostate tumor. The outcomes of dose escalation with 3D-CRT have been summarized in several other review articles (11-14). In general, they observed that the radiation dose could be escalated from the range of 64–68 Gy to the range of 74–81 Gy using 3D-CRT, and resulted in improved biochemical progression free survival rates. These results are summarized by a meta-analysis conducted by Viani et al., which pooled seven randomized trials with a total patient population of 2,812 (15). They identified that dose-escalated radiation resulted in significant reductions in biochemical failure, but there were no differences in the rate of all-cause or prostate cancer specific mortality. Furthermore, there were also more cases of late grade 2+ GI toxicity in the high-dose radiation groups, with the absolute difference typically ranging between 5–10% between the study arms.
In particular, the Radiation Therapy Oncology Group (RTOG) conducted protocol 9406 which used 3D-CRT to sequentially increase the radiation dose to several levels including 68.4 (1.8 Gy per fraction), 73.8 (1.8 Gy per fraction), 79.2 (1.8 Gy per fraction), 74.0 (2.0 Gy per fraction), and 78.0 (2.0 Gy per fraction) (16). These regimens resulted in disease free and biochemical progression free survival rates which compared favorably with historical data. Additionally, the toxicity results with 3D-CRT, including the maximum dose levels of 79.2 Gy in 1.8 Gy per fraction and 78.0 Gy in 2.0 Gy per fraction were low compared to historical controls (17). However, the 78 Gy in 2.0 Gy fractions dose level was ultimately associated with a greater incidence of late grade 2+ toxicity compared to 79.2 Gy in 1.8 Gy per fraction (30–33% vs. 9–13%). These results established a dose of 79.2 Gy in 1.8 Gy fractions for prostate cancer that is commonly used at Washington University in St. Louis.
An additional refinement on 3D-CRT is a technique known as IMRT. IMRT uses beam modulation and “field in field” techniques made possible by multi-leaf collimators combined with sophisticated inverse planning software. The IMRT approach allows the radiation oncologist to prescribe doses to target structures such as the prostate and proximal seminal vesicles and dose constraints to nearby normal structures such as the rectum and bladder. The computer software then determines the beam modulation to optimally achieve the radiation prescription. The resulting radiation distribution appears “sculpted” to cover the target and to avoid normal structures in a way that would typically not be achievable using conventional planning methods as seen in Figure 1. Although the mathematics underlying IMRT were published in the late 1980s and early 1990s, clinical IMRT systems were not implemented until the mid to late 1990s and commercial systems were not in common use until the early 2000s.
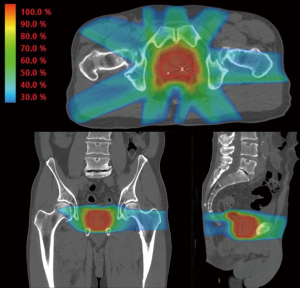
There are no randomized controlled trials directly comparing dose escalated IMRT and 3D-CRT in prostate cancer. However, several single institution retrospective studies have demonstrated improved biochemical control and/or reduced GI toxicity with IMRT as compared to 3D-CRT (18-20). Additionally, in RTOG 0126, a randomized study of dose escalation in intermediate risk prostate cancer, a reduction in late grade 2+ GI toxicity of 15% vs. 22% was observed with IMRT compared to 3D-CRT in the 79.2 Gy high dose arm (8). These studies will be discussed in detail later in this review.
The reduction in toxicity of IMRT as compared to 3D-CRT has led to its rapid adoption in the United States. In a SEER-Medicare study, IMRT use increased substantially as a proportion of patients treated with radiation from 28% in 2002 to 82% in 2005 (21). According to the most recent 2016 American College of Radiology Appropriateness Criteria, the use of 3D-CRT for dose escalated treatment of prostate cancer is typically no longer appropriate if other options, such as IMRT, are available (22).
Anatomic and biologic features which make prostate cancer a model site for implementation of IMRT
The prostate is located in close proximity to the rectum, bladder, penile bulb, and femoral heads which are at risk of receiving radiation dose and subsequent toxicity due to prostate cancer treatment. IMRT has been shown in dosimetric studies to substantially decrease the amount of unintentional radiation delivered to these normal structures. In RTOG 0126, a comparison of the dosimetry of IMRT and 3D-CRT plans in the 79.2 Gy arm showed that the percent of the rectum treated to at least 75 Gy (rectum V75) was reduced from 15.8% to 13.0%, and the V65 was reduced from 27.4% to 23.0% (8). The bladder V75 was reduced from 17.7% to 13.1% and the V65 was reduced from 25.3% to 19.7%. A dosimetric study by Hardcastle et al. showed similar findings suggesting a reduction in the rectum V75 from 23.2% to 9.9%, resulting in an estimated reduction in rectal complication rates from 14% to 5% (23). Luxton et al. have estimated that the mean dose to the femoral heads can be approximately halved by using IMRT over 3D-CRT (24). Additional studies have shown the possibility of IMRT in reducing radiation dose to the penile bulb (mean dose 33 vs. 49 Gy for IMRT vs. 3D-CRT) (25) The normal structure dose constraints used for IMRT plan optimization used at Washington University in St. Louis are listed in Table 1. The ability of IMRT to reduce dose to nearby normal structures without sacrificing dose coverage of the target makes it an ideal treatment modality for prostate cancer.
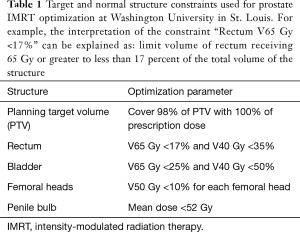
Full table
There may be additional radiobiological considerations that make prostate cancer a good target for IMRT, due to the alpha/beta ratio of the tumor and the use of hypofractionated radiation treatments. The response of tumor cells and normal tissue to therapeutic doses of radiation can be characterized by the alpha/beta ratio (α/β). This radiobiologic parameter is useful in determining the effect of various dose and fractionation schemes on the tissue. In this model, α represents the cells that die after one “hit” of radiation and β represents the cells that die after two “hits”, representing the ability to repair sublethal damage. The ratio of these two parameters, α/β, represents the “sensitivity” of a tissue to radiation. Rapidly proliferating cells, such as most tumors, are characterized by a α/β of ≥10 whereas most normal tissues, which are comprised of slower proliferating cells, have an α/β of 2–3. Tissues with low α/β are thought to be more sensitive to radiation given in large doses per treatment (large fraction sizes), while tissues with high α/β are thought to be more responsive to smaller fraction sizes. The combination of α/β ratio, dose per fraction, and number of fractions in a radiation treatment course can be used to calculate the biologically effective dose (BED), which is a measure of damage to tumor cells that can be calculated from many different radiation regimens.
Surprisingly, while most tumors have high α/β values, radiobiological models based on clinical data suggest that prostate cancer has a low α/β ratio of about 1.5 Gy, which is actually lower than that of many normal tissues in the pelvis (26,27). This finding implies that although dose escalation using conventional fractionation (1.8–2.0 Gy per fraction) has been effective for prostate cancer, an alternative method of improving the therapeutic ratio may include hypofractionation, the use of doses >2.0 Gy per radiation treatment (28).
The efficacy and toxicity of moderate hypofractionation has been tested in several studies (29). The United Kingdom CHHiP study randomized patients to 74 Gy in 2 Gy fractions vs. two hypofractionated regimens of 60 Gy in 20 fractions or 57 Gy in 19 fractions (29). IMRT was mandated in this study, and there were no differences in toxicity or cancer-related outcomes (29,30). The RTOG conducted a randomized study comparing a dose of 73.8 Gy in 1.8 Gy fractions to moderate hypofractionation to a dose of 70 Gy in 28 fractions (31). This study of 1,092 men with low risk prostate cancer established that the hypofractionated regimen was non-inferior in efficacy compared to the conventional regimen (31). However, there was an increase in late GI/GU adverse events with hypofractionated treatment. Both 3D-CRT and IMRT was allowed on this study. The Dutch HYPRO study also randomized patients between a conventional and hypofractionated dose regimen, and there was a ~95% utilization of IMRT in both arms (32). The late toxicity results of this study showed that the incidence of grade 3+ GI toxicity between the two regimens were not different (2.6% vs. 3.3%), but the late grade 3+ GU toxicity was not non-inferior between the conventional and hypofractionated treatments (13% and 19% respectively) (32). Five-year rates of treatment failure and relapse free survival were not significantly different between the two groups (32).
Although further analysis and reporting of the data is needed, the evidence above may suggest that IMRT is important to the delivery of moderate hypofractionation without excess GI toxicity. However, the ability of IMRT to reduce GU toxicity is limited as a portion of the bladder and prostatic urethra are necessarily within the treatment volume. Future studies will better define the appropriate tissue constraints to use for hypofractionated radiation, and the full benefit of IMRT in this setting.
Technical aspects of IMRT
IMRT combines inverse treatment planning and computer-controlled intensity modulation of the radiation to deliver 3D-CRT. Since the introduction of IMRT, many techniques for IMRT treatment of the prostate have been implemented (33). A common approach is the use of multiple coplanar fields arranged at equal or nearly equal spacing around the patient (33). Usually between 5 to 9 static fields are used for this approach, and in general, dose homogeneity and conformality improve as the number of treatment fields increases, however the benefit with field numbers beyond 7 to 9 diminishes (33,34). Noncoplanar beam arrangements, volumetric-modulated arc therapy (VMAT) and tomotherapy have been developed in attempts to further improve the dose distribution and are commonly used at many institutions.
IMRT treatment plans are designed by using specialized computer algorithms commonly termed “inverse treatment planning and optimization” systems (33). The user selects objectives, usually termed constraints and goals, that describe the desired dose to each target and organ at risk, and penalties are assigned based on the relative importance of each objective (33). These constraints and penalties are then combined into the mathematical algorithm according to a cost function and the intensity of beamlets within each field are adjusted iteratively until the cost function is minimized and thereby determining the intensity of each beam that leads to the closest profile as stated in the objective goals (33). Optimization objectives are both planning system and patient specific, therefore many institutions have developed institutional constraint templates that are used as a starting point for the planning process (33). The delivery of IMRT is than achieved by using either sequential delivery of multiple static apertures from a multileaf collimator (MLC) termed “step and shoot”, or as dynamic multileaf movement termed “sliding window” (35). Other techniques for delivery are available, but these two methods remain the most common.
After an acceptable IMRT plan has been generated, it is important to ensure the plan is properly implemented. Delivery of the complex intensity profiles created during the IMRT optimization process is performed by sophisticated mechanical and computerized delivery systems on a linear accelerator. Quality assurance is an integral part of this process and is typically performed with dosimetric verification of the leaf motion and sequencing for each field as well as computer based verification of the dose distribution and monitor unit settings for each field.
Outcomes with IMRT
Evidence from several single institution studies and multiple randomized control trials demonstrate a dose-response relationship with doses above 68 Gy associated with improved local and biochemical control (4,7,36). A summary of randomized dose escalation trials is found in Table 2. Additionally, dose-volume toxicity relationships have also been established for rectal bleeding and other GI and GU toxicities with increased high dose associated with increased risk of toxicity (4,37-40). IMRT is a method to escalate dose while achieving safe dose-volume constraints to organs at risk.
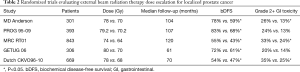
Full table
Published in 2007, Vora et al. evaluated biochemical control rates and prognostic factors for patients with localized prostate cancer treated with either high-dose IMRT or conventional-dose 3D-CRT. A total of 271 patients received 3D-CRT with a median dose of 68.4 Gy (range, 66.0–71.0 Gy), and 145 patients received IMRT with a median dose of 75.6 Gy (range, 70.2–77.4 Gy). Using the ASTRO Phoenix definition, the 5-year biochemical control rate was 74.4% for 3D-CRT and 84.6% with IMRT (P=0.033). On both univariate and multivariate analysis, increased dose was associated with improved biochemical control (19).
Despite improvements in prostate RT and increased dose conformity, rectal complications have not been eliminated and rectal toxicity remains a major concern for men undergoing EBRT. Giordano et al. (41) performed a population based analysis using the SEER database to determine the rates and predictors of late lower GI toxicity after prostate radiation prior to the IMRT era. They compared a group of men with prostate cancer treated with EBRT (n=24,130) compared to those treated without RT (n=33,835) from 1992 to 1999. For patients with a minimum of 5 years follow-up, the rates of GI diagnoses were 19.4% higher in the radiation group (41). Hemorrhage was the most common complication, and was increased by 19% for patients treated with radiation (39.6% of RT patients vs. comparison rates of 18.2% in patients treated with radical prostatectomy and 20.7% in patients with no local therapy) (41). Several single institutional series have reported a reduction in late toxicity since the introduction of IMRT as compared to conventional RT.
Jani et al. reviewed the records of 461 patients with localized prostate who received either IMRT (n=106) or conventional RT defined as 4- or 6-field conformal therapy (n=355) from 1998 to 2005 (42). Late GI toxicities were found to be lower with IMRT (P<0.001) and regression analyses demonstrated that IMRT was the only factor predictive of late GI toxicity. On analysis of the DVH data, it was hypothesized that the GI toxicity rates were lower for the IMRT group due to lower mid-to-high range rectal dosimetric metrics (V40, V50, V60, and V70). There was no association of decreased GU complications with IMRT compared to conventional treatment (42).
Sanguineti et al. compared the late rectal toxicity rates after 3D-CRT to the prostate alone and whole-pelvis IMRT along with a prostate boost to the same nominal total dose to the prostate in both groups (43). Sixty-eight patients were treated to the prostate alone using 3D-CRT to a total dose of 76 Gy. A second group of patients consisted of 45 patients treated with IMRT covering the pelvic lymph nodes and seminal vesicles to 54 Gy in 1.8 Gy fractions and the prostate to 60 Gy in the same 30 fractions followed by a boost to the prostate alone to 76 Gy (43). Planning was similar for both groups, with both receiving 76 Gy to the prostate, with the main difference being the inclusion of the pelvic lymph nodes in the IMRT group (43). Late toxicity was prospectively scored using the RTOG scale and all patients had a minimum of 12 months follow-up. At 2-years, the cumulative incidence of grade 2 late rectal toxicity was greater for the group receiving 3D-CRT (21.2%±6%) compared to IMRT (6%±4%) despite the IMRT group receiving treatment to the pelvic lymph nodes. On multivariate analysis the difference was statistically in favor of IMRT [hazard ratio (HR): 0.1; 95% CI, 0.0–0.6; P=0.01]. No patients in either group developed grade 3+ toxicity (43).
Wortel et al. analyzed the late side effects after treatment with image-guided (IG)-IMRT or 3D-CRT, evaluating 2 prospective cohorts of men treated with localized prostate cancer to investigate hypothesized reductions in toxicity (44). Patients from two Dutch randomized trials were treated with 3D-CRT (n=189) or IG-IMRT (n=242) to 78 Gy in 39 fractions with identical toxicity scoring protocols (modified RTOG-EORTC scoring criteria). The 5-year cumulative incidence of grade ≥2 GI toxicity was 24.9% for IG-IMRT and 37.6% following 3D-CRT (adjusted HR: 0.59, P=0.005). There was significant reduction in proctitis (HR: 0.37, P=0.047) and increased stool frequency (HR: 0.23, P<0.001). GU grade ≥2 toxicity remained comparable between the two groups. Other predictors of late grade ≥2 complications were baseline complaints, acute toxicity, and age (44).
The RTOG 0126 was a prospective randomized phase III trial comparing escalated high dose RT to conventional dose RT for localized early stage intermediate risk prostate cancer which opened in March 2002 and closed to accrual in 2008. The primary endpoint of the study was to determine whether 3D-CRT or IMRT to 79.2 Gy in 44 fractions would lead to improved overall survival in patients with intermediate risk prostate cancer compared to those patients treated with the same techniques to 70.2 Gy in 39 fractions. The protocol initially included only 3D-CRT, however, in September 2003 the trial was amended to allow IMRT, and treatment modality was added as a stratification variable in order to help avoid treatment arm modality imbalances. Of the 1,532 patients enrolled on the trial, 763 were randomized to the high dose treatment arm. In this arm, patients treated with 3D-CRT received 55.8 Gy to a planning target volume that included the prostate and seminal vesicles, and then a 23.4 Gy boost to the prostate alone. Patients receiving IMRT were treated to the prostate and seminal vesicles to 79.2 Gy. In 2013, a preliminary toxicity analysis of 3D-CRT versus IMRT was published reporting acute and late effects between the two techniques (8). 748 of the 763 patients were eligible of whom 491 received 3D-CRT and 257 received IMRT. After dosimetric analysis, the median % of the bladder receiving at least xGy (pVx equals partial volume receiving ‘x’ Gray) for pV65, pV70 and pV75 were 25.3%, 22.2%, and 17.7% for 3D-CRT and 19.7%, 16.6% and 13.1% for IMRT. The median rectum pV65, pV70 and pV75 were 27.4%, 21.7%, and 15.8% for 3D-CRT and 23.0%, 18.2% and 13.0% for IMRT. For both the bladder and rectum, the volumes receiving 65, 70, and 75 Gy were significantly lower in the IMRT cohort (all P<0.0001). For grade 2+ acute GI/GU toxicity, both univariate and multivariate analyses showed a significant decrease in collective GI/GU toxicities in favor of IMRT. There was no significant difference between 3D-CRT or IMRT for acute or late grade 2+ or 3+ GU toxicities. Univariate analysis indicated a statistically significant decrease in late grade 2+ GI toxicity for IMRT (P=0.039). In multivariate analysis, IMRT had a 26% reduction in late grade 2+ GI toxicity compared to 3D-CRT (P=0.099). Additionally, small volumes of the rectum exceeding high threshold radiation doses (i.e., >70 Gy) were associated with nearly a two-fold risk of late grade 2 or greater toxicity. If more than 10% or 15% of the rectum volume exceeded 75 or 70 Gy, respectively, patients had a significantly greater risk of late GI toxicity. After both modality and the dose thresholds were included in the GI toxicity analysis, there was still a separation between the 3D and IMRT arms at each dose constraint level with a larger, however not statistically significant, separation for the >10% dose constraint groups. Based on these preliminary findings of RTOG 0126, IMRT is associated with a significant reduction in acute grade 2+ GI/GU toxicity and there is a trend for a clinically meaningful reduction in late grade 2+ GI toxicity with IMRT. The occurrence of acute GI toxicity and large (>15%) volumes of rectum >70 Gy are associated with late rectal toxicity. The final results of this trial are pending publication this year.
In September 2017, a national population based study was performed comparing treatment-related toxicity in men who received IMRT versus 3D-CRT for prostate cancer (45). Patients treated for prostate cancer between January 2010 and December 2013 in the English National Health Service were included (N=23,222). A total of 16,289 patients treated with 3D-CRT and 6,933 patients with IMRT. Patients with severe toxicity, defined as at least grade 3 according to the National Cancer Institute Common Terminology Criteria for Adverse Events scoring system were identified. A competing risks regression analysis was used to estimate HRs, comparing the incidence of severe GI and GU complications after IMRT and 3D-CRT, adjusting for patient, disease, and treatment characteristics. The use of IMRT, as opposed to 3D-CRT, increased from 3.1% in 2010 to 64.7% in 2013 in this cohort. Patients who received IMRT were less likely than those receiving 3D-CRT to experience severe GI toxicity [4.9 vs. 6.5 per 100 person-years; adjusted HR: 0.66; 95% confidence interval (CI), 0.61–0.72]. Similar rates of GU toxicity were observed (2.3 vs. 2.4 per 100 person-years; adjusted HR: 0.94; 95% CI, 0.84–1.06) (45).
Lastly, a meta-analysis of 23 studies (n=9,556) comparing the clinical outcomes, including GI toxicity, GU toxicity, biochemical control and overall survival was performed (46). IMRT was significantly associated with decreased grade 2–4 acute GI toxicity (risk ratio =0.59 (95% CI, 0.44–0.78), late GI toxicity (risk ratio =0.54, 95% CI, 0.38–0.78), late rectal bleeding (risk ratio =0.48, 95% CI, 0.27–0.85), and achieved better bio-chemical control (risk ratio =1.17, 95% CI, 1.08–1.27) in comparison with 3D-CRT (46). IMRT and 3D-CRT remained the same in regard to grade 2–4 acute rectal toxicity (risk ratio =1.03, 95% CI, 0.45–2.36), late GU toxicity (risk ratio =1.03, 95% CI, 0.82–1.30) and overall survival (risk ratio =1.07, 95% CI, 0.96–1.19), while IMRT slightly increased the morbidity of grade 2–4 acute GU toxicity (risk ratio =1.08, 95% CI, 1.00–1.17) (46).
IMRT for pelvic lymph node radiation
A subset of patients with prostate cancer, typically those with high risk disease, may benefit from radiation to the pelvic lymph nodes in addition to the prostate and proximal seminal vesicles. In these situations, the use of IMRT might also be beneficial in reducing toxicity in comparison to 3D-CRT. Although no randomized study has been conducted in prostate cancer comparing 3D-CRT and IMRT techniques in treating the pelvic lymph nodes, some insight can be gained from extrapolating from other disease sites in which pelvic nodal coverage is indicated. For instance, the multi-national TIME-C study enrolled 278 patients with endometrial or cervical cancer and randomized the patients after surgery to IMRT or four-field pelvic radiation treatment and evaluated patients using the Expanded Prostate Cancer Index Composite (EPIC) (47). A preliminary presentation of the toxicity outcomes at ASTRO in 2016 suggested that IMRT reduced GI toxicity including diarrhea and fecal incontinence compared to 3D-CRT (47). Patients receiving IMRT also had smaller declines in EPIC urinary domain scores. Limitations in extrapolating data from this study to patients with prostate cancer includes the fact that patients in TIME-C received prior surgery, a subset received concurrent chemotherapy, and the volumes treated with radiation for gynecological tumors may differ from that for prostate cancer.
Additional insight on IMRT to the pelvic volume may be gained by examining retrospective and single arm prospective studies in prostate cancer. A dosimetric study by Guckenberger et al. compared the risk of toxicity using normal tissue complication probability (NTCP) calculations after IMRT to the prostate only compared to additional irradiation of the pelvic lymphatic region in a retrospective cohort of 10 patients (48). They concluded from this planning study that the NTCP model predicted similar risks of rectal (5–8%) and bladder (1%) toxicity after prostate-only or prostate and pelvis IMRT. However the risk of toxicity to the small bowel was estimated to be increased with inclusion of the pelvic volume IMRT to 0.8–3.2%. Deville et al. retrospectively compared patients treated with dose-escalated IMRT to 79.2 Gy to the prostate alone or also with 45 Gy by IMRT to the pelvis (49). Although the acute grade 2+ GI toxicity was greater with the inclusion of pelvic radiation (50% vs. 13%), there was no difference in late GI or GU toxicity with the addition of pelvic IMRT (49). These estimated risks are also similar to the observed toxicity reported in a phase 1–2 study of dose-escalated IMRT to the prostate and pelvic nodes reported by Reis Ferreira et al. (50). They enrolled 447 patients with locally advanced prostate cancer and treated with IMRT of 70–74 Gy to the prostate and dose escalated IMRT to the pelvic lymph nodes to 50–60 Gy. The 2-year rates of grade 2+ bowel and bladder toxicity in patients receiving conventionally fractionated pelvic IMRT ranged from 8.3–13.2% and 2.9–5.9% respectively. The importance of reducing toxicity is especially important as it is becoming recognized that larger radiation fields such as those extending to L4/L5 may be necessary to adequately cover the lymph node regions commonly involved with prostate cancer metastasis (51).
In the setting of modern dose escalation and androgen depravation therapy, the benefit of pelvic nodal irradiation in patients with unfavorable intermediate risk and high risk prostate cancer is still debatable. RTOG 0924 is an ongoing study investigating the benefit of pelvic radiation in addition to ADT and prostate dose escalation (52). Although both 3D-CRT and IMRT is allowed for the pelvic field, it is expected that most of the patients will be treated with IMRT. Outcomes and toxicity data from this randomized study will better define the benefit and risks of covering the nodal regions with IMRT in prostate cancer.
Future directions
As described above, clinical studies suggest that prostate cancer has biologic characteristics which may make it more sensitive to larger doses per fraction (i.e., hypofractionation) (27,53). These higher doses per fraction may lead to better tumor kill compared to conventional fractionation while offering increased patient convenience from a shorter course of treatment and also fewer burdens on the health care system with decreased treatment costs. The CHHiP trial (a randomized, phase 3, non-inferiority trial) demonstrated that hypofractionated radiotherapy with IMRT using 60 Gy in 20 fractions is non-inferior to conventional fractionation using 74 Gy in 37 fractions and is recommended as a new standard of care for external-beam radiotherapy of localized prostate cancer (54). Further hypofractionation, specifically stereotactic body radiation therapy (SBRT) which uses even larger doses per fraction, are currently under investigation. RTOG 0938 is a randomized phase II trial of hypofractionated radiation therapy for favorable risk prostate cancer in which patients are assigned to either 36.25 Gy in 5 fractions (7.25 Gy per fraction) versus 51.6 Gy in 12 fractions (4.3 Gy per fraction) (55). This protocol requires the use of IMRT or related technologies (i.e., Tomotherapy/VMAT/Cyberknife and proton therapy) given the large doses per fraction and risk to healthy pelvic organs (55).
Additional methods to improve the toxicity profile of IMRT for prostate cancer are the use of a hydrogel spacer. Given that the prostate and rectum are often in immediate physical contact, even the most conformal IMRT plan cannot always spare high dose radiation being delivered to the rectum. An absorbable hydrogel injected between the prostate and rectum has been shown in a randomized trial to have a clinically significant 25% reduction in the rectal V70 Gy in >97% of men, correlating with a reduction in rectal toxicity, improvement in bowel quality of life, and improved sexual function (56-59).
Conclusions
Radiation therapy for prostate cancer has evolved dramatically over the past 2 decades. Treatment has evolved from X-ray fields based on bony anatomy to dose escalated radiation therapy with image-guidance and IMRT. Published dose-volume constraints that can reduce or prevent rectal injury have been established and are achievable with IMRT. Multiple randomized, retrospective, and population based studies have shown that men who receive radiation therapy using IMRT were less likely to experience severe GI toxicity compared with those who received 3D-CRT. The radiobiology of prostate cancer also suggests it may be more responsive to hypofractionated treatment, and IMRT is a method to deliver higher doses per fraction while minimizing dose to organs at risk. Randomized trials investigating extreme hypofractionation (SBRT) are underway.
Acknowledgements
None.
Footnote
Conflicts of Interest: The authors have no conflicts of interest to declare.
References
- Miller KD, Siegel RL, Lin CC, et al. Cancer treatment and survivorship statistics, 2016. CA Cancer J Clin 2016;66:271-89. [Crossref] [PubMed]
- Hanks GE, Hanlon AL, Epstein B, et al. Dose response in prostate cancer with 8-12 years' follow-up. Int J Radiat Oncol Biol Phys 2002;54:427-35. [Crossref] [PubMed]
- Dearnaley DP, Sydes MR, Graham JD, et al. Escalated-dose versus standard-dose conformal radiotherapy in prostate cancer: first results from the MRC RT01 randomised controlled trial. Lancet Oncol 2007;8:475-87. [Crossref] [PubMed]
- Peeters ST, Heemsbergen WD, Koper PC, et al. Dose-response in radiotherapy for localized prostate cancer: results of the Dutch multicenter randomized phase III trial comparing 68 Gy of radiotherapy with 78 Gy. J Clin Oncol 2006;24:1990-6. [Crossref] [PubMed]
- Pollack A, Zagars GK, Smith LG, et al. Preliminary results of a randomized radiotherapy dose-escalation study comparing 70 Gy with 78 Gy for prostate cancer. J Clin Oncol 2000;18:3904-11. [Crossref] [PubMed]
- Zelefsky MJ, Leibel SA, Gaudin PB, et al. Dose escalation with three-dimensional conformal radiation therapy affects the outcome in prostate cancer. Int J Radiat Oncol Biol Phys 1998;41:491-500. [Crossref] [PubMed]
- Zietman AL, DeSilvio ML, Slater JD, et al. Comparison of conventional-dose vs high-dose conformal radiation therapy in clinically localized adenocarcinoma of the prostate: a randomized controlled trial. JAMA 2005;294:1233-9. [Crossref] [PubMed]
- Michalski JM, Yan Y, Watkins-Bruner D, et al. Preliminary toxicity analysis of 3-dimensional conformal radiation therapy versus intensity modulated radiation therapy on the high-dose arm of the Radiation Therapy Oncology Group 0126 prostate cancer trial. Int J Radiat Oncol Biol Phys 2013;87:932-8. [Crossref] [PubMed]
- Zelefsky MJ, Fuks Z, Happersett L, et al. Clinical experience with intensity modulated radiation therapy (IMRT) in prostate cancer. Radiother Oncol 2000;55:241-9. [Crossref] [PubMed]
- Dearnaley DP, Khoo VS, Norman AR, et al. Comparison of radiation side-effects of conformal and conventional radiotherapy in prostate cancer: a randomised trial. Lancet 1999;353:267-72. [Crossref] [PubMed]
- Yamazaki H, Nakamura S, Nishimura T, et al. Transitioning from conventional radiotherapy to intensity-modulated radiotherapy for localized prostate cancer: changing focus from rectal bleeding to detailed quality of life analysis. J Radiat Res 2014;55:1033-47. [Crossref] [PubMed]
- Pearlstein KA, Chen RC. Comparing dosimetric, morbidity, quality of life, and cancer control outcomes after 3D conformal, intensity-modulated, and proton radiation therapy for prostate cancer. Semin Radiat Oncol 2013;23:182-90. [Crossref] [PubMed]
- Zaorsky NG, Harrison AS, Trabulsi EJ, et al. Evolution of advanced technologies in prostate cancer radiotherapy. Nat Rev Urol 2013;10:565-79. [Crossref] [PubMed]
- Martin NE, D'Amico AV. Progress and controversies: Radiation therapy for prostate cancer. CA Cancer J Clin 2014;64:389-407. [Crossref] [PubMed]
- Viani GA, Stefano EJ, Afonso SL. Higher-than-conventional radiation doses in localized prostate cancer treatment: a meta-analysis of randomized, controlled trials. Int J Radiat Oncol Biol Phys 2009;74:1405-18. [Crossref] [PubMed]
- Michalski J, Winter K, Roach M, et al. Clinical outcome of patients treated with 3D conformal radiation therapy (3D-CRT) for prostate cancer on RTOG 9406. Int J Radiat Oncol Biol Phys 2012;83:e363-70. [Crossref] [PubMed]
- Michalski JM, Winter K, Purdy JA, et al. Toxicity after three-dimensional radiotherapy for prostate cancer with RTOG 9406 dose level IV. Int J Radiat Oncol Biol Phys 2004;58:735-42. [Crossref] [PubMed]
- Zelefsky MJ, Yamada Y, Fuks Z, et al. Long-term results of conformal radiotherapy for prostate cancer: impact of dose escalation on biochemical tumor control and distant metastases-free survival outcomes. Int J Radiat Oncol Biol Phys 2008;71:1028-33. [Crossref] [PubMed]
- Vora SA, Wong WW, Schild SE, et al. Analysis of biochemical control and prognostic factors in patients treated with either low-dose three-dimensional conformal radiation therapy or high-dose intensity-modulated radiotherapy for localized prostate cancer. Int J Radiat Oncol Biol Phys 2007;68:1053-8. [Crossref] [PubMed]
- Viani GA, Viana BS, Martin JE, et al. Intensity-modulated radiotherapy reduces toxicity with similar biochemical control compared with 3-dimensional conformal radiotherapy for prostate cancer: A randomized clinical trial. Cancer 2016;122:2004-11. [Crossref] [PubMed]
- Nguyen PL, Gu X, Lipsitz SR, et al. Cost implications of the rapid adoption of newer technologies for treating prostate cancer. J Clin Oncol 2011;29:1517-24. [Crossref] [PubMed]
- Zaorsky NG, Showalter TN, Ezzell GA, et al. ACR Appropriateness Criteria((R)) external beam radiation therapy treatment planning for clinically localized prostate cancer, part I of II. Adv Radiat Oncol 2016;2:62-84. [Crossref] [PubMed]
- Hardcastle N, Davies A, Foo K, et al. Rectal dose reduction with IMRT for prostate radiotherapy. J Med Imaging Radiat Oncol 2010;54:235-48. [Crossref] [PubMed]
- Luxton G, Hancock SL, Boyer AL. Dosimetry and radiobiologic model comparison of IMRT and 3D conformal radiotherapy in treatment of carcinoma of the prostate. Int J Radiat Oncol Biol Phys 2004;59:267-84. [Crossref] [PubMed]
- Kao J, Turian J, Meyers A, et al. Sparing of the penile bulb and proximal penile structures with intensity-modulated radiation therapy for prostate cancer. Br J Radiol 2004;77:129-36. [Crossref] [PubMed]
- Brenner DJ, Hall EJ. Fractionation and protraction for radiotherapy of prostate carcinoma. Int J Radiat Oncol Biol Phys 1999;43:1095-101. [Crossref] [PubMed]
- Fowler J, Chappell R, Ritter M. Is alpha/beta for prostate tumors really low? Int J Radiat Oncol Biol Phys 2001;50:1021-31. [Crossref] [PubMed]
- Tree AC, Alexander EJ, Van As NJ, et al. Biological dose escalation and hypofractionation: what is there to be gained and how will it best be done? Clin Oncol (R Coll Radiol) 2013;25:483-98. [Crossref] [PubMed]
- Dearnaley D, Syndikus I, Sumo G, et al. Conventional versus hypofractionated high-dose intensity-modulated radiotherapy for prostate cancer: preliminary safety results from the CHHiP randomised controlled trial. Lancet Oncol 2012;13:43-54. [Crossref] [PubMed]
- Wilkins A, Mossop H, Syndikus I, et al. Hypofractionated radiotherapy versus conventionally fractionated radiotherapy for patients with intermediate-risk localised prostate cancer: 2-year patient-reported outcomes of the randomised, non-inferiority, phase 3 CHHiP trial. Lancet Oncol 2015;16:1605-16. [Crossref] [PubMed]
- Lee WR, Dignam JJ, Amin MB, et al. Randomized Phase III Noninferiority Study Comparing Two Radiotherapy Fractionation Schedules in Patients With Low-Risk Prostate Cancer. J Clin Oncol 2016;34:2325-32. [Crossref] [PubMed]
- Aluwini S, Pos F, Schimmel E, et al. Hypofractionated versus conventionally fractionated radiotherapy for patients with prostate cancer (HYPRO): late toxicity results from a randomised, non-inferiority, phase 3 trial. Lancet Oncol 2016;17:464-74. [Crossref] [PubMed]
- Cahlon O, Hunt M, Zelefsky MJ. Intensity-modulated radiation therapy: supportive data for prostate cancer. Semin Radiat Oncol 2008;18:48-57. [Crossref] [PubMed]
- Yu CX, Li XA, Ma L, et al. Clinical implementation of intensity-modulated arc therapy. Int J Radiat Oncol Biol Phys 2002;53:453-63. [Crossref] [PubMed]
- Spirou SV, Chui CS. Generation of arbitrary intensity profiles by dynamic jaws or multileaf collimators. Med Phys 1994;21:1031-41. [Crossref] [PubMed]
- Pollack A, Zagars GK, Starkschall G, et al. Prostate cancer radiation dose response: results of the M. D. Anderson phase III randomized trial. Int J Radiat Oncol Biol Phys 2002;53:1097-105. [Crossref] [PubMed]
- Boersma LJ, van den Brink M, Bruce AM, et al. Estimation of the incidence of late bladder and rectum complications after high-dose (70-78 GY) conformal radiotherapy for prostate cancer, using dose-volume histograms. Int J Radiat Oncol Biol Phys 1998;41:83-92. [Crossref] [PubMed]
- Koper PC, Heemsbergen WD, Hoogeman MS, et al. Impact of volume and location of irradiated rectum wall on rectal blood loss after radiotherapy of prostate cancer. Int J Radiat Oncol Biol Phys 2004;58:1072-82. [Crossref] [PubMed]
- Vargas C, Martinez A, Kestin LL, et al. Dose-volume analysis of predictors for chronic rectal toxicity after treatment of prostate cancer with adaptive image-guided radiotherapy. Int J Radiat Oncol Biol Phys 2005;62:1297-308. [Crossref] [PubMed]
- Vavassori V, Fiorino C, Rancati T, et al. Predictors for rectal and intestinal acute toxicities during prostate cancer high-dose 3D-CRT: results of a prospective multicenter study. Int J Radiat Oncol Biol Phys 2007;67:1401-10. [Crossref] [PubMed]
- Giordano SH, Lee A, Kuo YF, et al. Late gastrointestinal toxicity after radiation for prostate cancer. Cancer 2006;107:423-32. [Crossref] [PubMed]
- Jani AB, Su A, Correa D, Gratzle J. Comparison of late gastrointestinal and genitourinary toxicity of prostate cancer patients undergoing intensity-modulated versus conventional radiotherapy using localized fields. Prostate Cancer Prostatic Dis 2007;10:82-6. [Crossref] [PubMed]
- Sanguineti G, Cavey ML, Endres EJ, et al. Does treatment of the pelvic nodes with IMRT increase late rectal toxicity over conformal prostate-only radiotherapy to 76 Gy? Strahlenther Onkol 2006;182:543-9. [Crossref] [PubMed]
- Wortel RC, Incrocci L, Pos FJ, et al. Late side effects after image guided intensity modulated radiation therapy compared to 3d-conformal radiation therapy for prostate cancer: results from 2 prospective cohorts. Int J Radiat Oncol Biol Phys 2016;95:680-9. [Crossref] [PubMed]
- Sujenthiran A, Nossiter J, Charman SC, et al. National population-based study comparing treatment-related toxicity in men who received intensity modulated versus 3-dimensional conformal radical radiation therapy for prostate cancer. Int J Radiat Oncol Biol Phys 2017;99:1253-60. [Crossref] [PubMed]
- Yu T, Zhang Q, Zheng T, et al. The effectiveness of intensity modulated radiation therapy versus three-dimensional radiation therapy in prostate cancer: a meta-analysis of the literatures. PloS One 2016;11. [Crossref] [PubMed]
- Klopp AH, Yeung AR, Deshmukh S, et al. A Phase III randomized trial comparing patient-reported toxicity and quality of life (QOL) during pelvic intensity modulated radiation therapy as compared to conventional radiation therapy. Int J Radiat Oncol Biol Phys 2016;96:S3. [Crossref]
- Guckenberger M, Baier K, Richter A, et al. Does intensity modulated radiation therapy (IMRT) prevent additional toxicity of treating the pelvic lymph nodes compared to treatment of the prostate only? Radiat Oncol 2008;3:3. [Crossref] [PubMed]
- Deville C, Both S, Hwang WT, et al. Clinical toxicities and dosimetric parameters after whole-pelvis versus prostate-only intensity-modulated radiation therapy for prostate cancer. Int J Radiat Oncol Biol Phys 2010;78:763-72. [Crossref] [PubMed]
- Reis Ferreira M, Khan A, Thomas K, et al. Phase 1/2 dose-escalation study of the use of intensity modulated radiation therapy to treat the prostate and pelvic nodes in patients with prostate cancer. Int J Radiat Oncol Biol Phys 2017;99:1234-42. [Crossref] [PubMed]
- Spratt DE, Vargas HA, Zumsteg ZS, et al. Patterns of lymph node failure after dose-escalated radiotherapy: implications for extended pelvic lymph node coverage. Eur Urol 2017;71:37-43. [Crossref] [PubMed]
- Androgen-deprivation therapy and radiation therapy in treating patients with prostate cancer. NCT01368588. Available online: https://clinicaltrials.gov/ct2/show/NCT01368588
- Brenner DJ, Martinez AA, Edmundson GK, et al. Direct evidence that prostate tumors show high sensitivity to fractionation (low alpha/beta ratio), similar to late-responding normal tissue. Int J Radiat Oncol Biol Phys 2002;52:6-13. [Crossref] [PubMed]
- Dearnaley D, Hall E. Hypofractionated radiotherapy for prostate cancer - Authors' reply. Lancet Oncol 2016;17. [Crossref] [PubMed]
- RTOG 0938 Protocol Information: a randomized Phase II trial of hypofractionated radiotherapy for favorable risk prostate cancer. Available online: https://www.rtog.org/ClinicalTrials/ProtocolTable/StudyDetails.aspx?study=0938
- Hamstra DA, Mariados N, Sylvester J, et al. Continued Benefit to Rectal Separation for Prostate Radiation Therapy: Final Results of a Phase III Trial. Int J Radiat Oncol Biol Phys 2017;97:976-85. [Crossref] [PubMed]
- Fischer-Valuck BW, Chundury A, Gay H, et al. Hydrogel spacer distribution within the perirectal space in patients undergoing radiotherapy for prostate cancer: Impact of spacer symmetry on rectal dose reduction and the clinical consequences of hydrogel infiltration into the rectal wall. Pract Radiat Oncol 2017;7:195-202. [Crossref] [PubMed]
- Hamstra DA, Mariados N, Sylvester J, et al. Sexual quality of life following prostate intensity modulated radiation therapy (IMRT) with a rectal/prostate spacer: Secondary analysis of a phase 3 trial. Pract Radiat Oncol 2018;8:e7-15. [Crossref] [PubMed]
- Mariados N, Sylvester J, Shah D, et al. Hydrogel spacer prospective multicenter randomized controlled pivotal trial: dosimetric and clinical effects of perirectal spacer application in men undergoing prostate image guided intensity modulated radiation therapy. Int J Radiat Oncol Biol Phys 2015;92:971-7. [Crossref] [PubMed]