Endocrine disrupting chemicals and impact on male reproductive health
Introduction
The endocrine system maintains homeostasis of the bodily systems through hormones that can travel long distances in the body and often have amplified effects. Endocrine disrupting chemicals (EDCs) are the substances which change the course of endocrine systems in a way that adversely affects the organism itself or its progeny (1). These chemicals can be found in a variety of everyday products and goods, such as in foods, water, plastics, shampoos, clothes, toothpastes, soaps, fertilizers, paper, textiles, carpets, utensils, bedding, toy, cosmetics, deodorant, etc. (2-4). Because of the use of EDCs in several consumer goods and personal care products, humans are exposed to the harmful effects of these substances in a variety of ways which include ingestion, germination, inhalation, and dermal contact. Hence, EDCs call for greater attention because of their increasing utility in daily products and possible correlation with compromised male reproductive health. The endocrine system is particularly important for male reproductive development because androgens (such as testosterone) promote the maturation of male secondary characteristics as well as the process of spermatogenesis. Male reproductive health- specifically sperm count and testosterone-have been declining (5,6), which is correlated with an increase in a variety of EDCs, such as perfluoroalkyl compounds (2). Regional differences have also been reported in urban versus rural areas showing a statistical correlation between poor semen quality and higher levels of EDCs found in pesticides, such as alachlor, diazinon, atrazine, metolachlor, and 2,4-dichlorophenoxyacetic acid (7). Such evidence linking the increasing prevalence of EDCs to declining semen quality and male reproductive health calls attention to the detrimental effects of EDCs.
Semen parameters
Semen parameters are used to measure the quality of sperm and are important because they can be used to predict male infertility, which is defined as the inability to fertilize an egg after 12 months of unprotected intercourse. From 1999 till 2010, the reference values defined in the WHO 1999 manual were used to consider a semen analysis normal or abnormal. In 2010, these values were re-evaluated and new cutoffs were introduced which are lower than WHO 1999 (Table 1). A conventional semen analysis report is considered the first diagnostic test in male infertility. Based on the semen parameters results assessed against WHO 2010 criteria, a decision of abnormal and normal is declared. However, it is not feasible to certify a male fertile or infertile just based on the results of a conventional semen analysis. Low quality semen can be used as a predictor for male infertility, but such men can still be fertile. Similarly, men with high quality semen may still be infertile. However, according to the WHO, only 30% of men with high quality semen are infertile, whereas men with low quality semen are much more likely to be infertile. Thus, the semen parameters are significant as indicators of compromised male fertility.
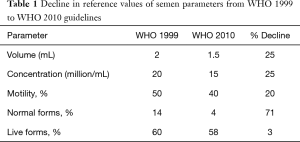
Full table
Decline in semen parameters and EDCs
Over the past several years, male reproductive health has been on the decline (5,8). Globally, incidences of testicular cancer (TC), cryptorchidism, and hypospadias have been rising, and sperm quality has been decreasing (5). Male fertility is measured, in large part, by qualitative and quantitative measures of semen parameters (9). A continuous decrease in sperm count, volume, motility, morphology, and concentration has been reported, which has contributed to an increase in male infertility (5,8-10). For instance, Auger et al. (1995) showed a 2.1% decrease in sperm count and a 0.6% per year decrease in sperm motility from 1973 to 1992 (11). Swan et al. (2000) reported that mean sperm concentration has declined 1.5% per year in the U.S. from 1938 to 1988, and 3.1% per year in Europe from 1971 to 1990 (12). Rolland et al. (2013) showed a 32.2% decrease in sperm concentration, and 33.4% in sperm morphology from 1989 to 2005 (13).
EDC levels have simultaneously been rising, supporting the argument that EDC exposure contributes to decreased semen quality and thus increased male infertility. For instance, polybrominated diphenyl ethers (PBDE) have been increasing globally over the past 30 years; dichlorodiphenyltrichloroethane (DDT) and dichlorodiphenylethane (DDE) use has been increased in Africa and other parts of the world; and multiple EDCs such as bisphenol A (BPA), alkylphenols, pentachlorophenol (PCP), and triclosan have become more prominent in the environment because of manufacturing and because of their presence in widespread consumer products such as soaps, toothpastes, and wood preservations (14-16). Associations between decreasing male fertility and increasing EDC levels suggest that the decline of male reproductive health over the past few decades is due not only to genetic but also environmental factors.
EDC’s leading to decreased semen quality
Phthalate metabolites, used in plastic to soften PVC polymers, have been identified in 75% of Americans. Children have shown 2–4 times higher levels as compared to adults (17). Similarly, the plasticizer BPA has been detected in urine samples of at least 90% of subjects in the United States, Germany, and Canada (1). Both phthalates and BPA have been studied as EDCs which negatively affect male reproductive health. The pre and neonatal exposure and subsequent effects of these chemicals are especially striking.
Phthalate exposure in neonatal male rats has been shown to reduce testicular testosterone levels, which results in down regulation of certain genes associated with steroidogenesis (1). Similarly, exposure to the metabolite di(n-butyl) phthalate (DBP) has shown reduction in testosterone production in rodents (18). In neonatal marmosets, DBP exposure induced significant Leydig cell aggregation (LCA), which can be an indicator of potential conditions such as cryptorchidism, hypospadias, TC, and reduced semen quality collectively grouped as testicular dysgenesis syndrome (TDS) (18). The adverse effects of phthalates on steroidogenesis in neonatal rodent primates, including humans, is a matter of great concern because higher levels of phthalates have been identified in younger children (1). Exposure to EDCs during critical periods of neonatal development has been an issue which warrants attention to the idea that prenatal exposure may be an even more significant factor than neonatal exposure in the development of TDS (1). Furthermore, in women 2–4 times higher phthalates have been reported as compared to men, suggesting a greater risk of exposing the developing child to such higher phthalates levels (17). In utero exposure to phthalate esters DBP and di-(2-ethylhexyl) phthalate (DEHP) caused inhibition of testosterone production as well as increased LCA, both conditions which are indicators of impaired spermatogenesis (7). However, in utero exposure resulted in abnormal seminiferous cord formation and multinucleation of spermatogonia in cryptorchid boys (7). Embryonic exposure to DEHP has been linked to a decrease in sperm motility, disruption of testicular germ cell organization and spermatogonial stem cell function (17). Shortened anogenital distance (AGD) and impaired testicular descent have also been reported as a result of phthalates exposure (19).
BPA has been associated with an increased risk of cryptorchidism and reduced semen quality (3). Bilateral cryptorchidism is associated with greater risk of azoospermia, poor semen quality, increased risk of TC and reduced paternal fertility rates, even after treatment (20). Increased prostate cancer progression has also been correlated with BPA exposure. The prostate gland has a crucial role in sperm motility by providing prostatic fluid (1,21). Prostate cancer is rated as the second most common male malignancy in North America (21). BPA has shown higher levels in prostate cancer patients compared to non-prostate cancer men. Further, BPA exposure has shown reduction in normal sperm morphology and motility in mice model (3).
Like rodents, human exposure to EDCs has also been correlated with decreased semen quality. For example, Faure et al. 2013 found that men exposed to dioxins had a greater number of morphologically abnormal sperm and low linear motility (9). Jurewicz et al. reported that increased exposure to polychlorinated biphenyls (PCBs) was associated with decreased sperm count, motility, and normal morphology (22). Additionally, pesticides have shown decreased normal sperm morphology, count, volume and motility (15,22). Organophosphate exposure also reduced semen volume and increased pH, and exposure to phthalates decreased concentration, normal morphology, and motility (22). These findings in humans indicate that EDC exposure does affect human semen quality in a way that is similarly modeled by rodents in other studies.
Though the effects of individual EDCs have been reported, it is important to consider the fact that humans can be simultaneously exposed to a large variety of these chemicals. EDCs can have dose-additive effects that can result in as large as a 50% discrepancy in terms of malformation in men (1). The combined effect of EDCs is more alarming and calls for greater attention because it is more realistic in mimicking human exposure.
Mechanisms of action
Different EDCs impact semen quality through various mechanisms. It has been shown that phthalates, BPA, dioxins, and PCB are all associated with decreased semen quality; however, their mechanisms of action affect different parts of the endocrine system in relation to semen quality and male reproduction.
Phthalates are of particular health concern due to their presence in common consumer goods and their impact on male reproduction. For example, several studies have shown that mono-(2-ethyl-hexyl) phthalate (MEHP), the reactive metabolite of DEHP, activates both PPAR (peroxisome proliferator-activated receptor) α and PPARγ pathways (23,24). This stimulates PPAR:RXR (retinoid X receptor) heterodimers to compete for binding sites on DNA and limited coactivators required for gene transcription, thereby inhibiting the transcription of aromatase, an enzyme involved in sexual development (23,25). Additionally, MEHP-activated PPARγ ligands decrease cAMP response element-binding protein (CREB) and Sp1 from binding to DNA for transcription, thereby preventing them from positively regulating the transcription of steroidogenic genes (24). StAR and p450c17 in particular are tightly regulated by cAMP. PPARγ ligands significantly diminish these steroidogenic proteins, leading to low sperm quality (24). StAR’s function is to mediate the rate-limiting step in steroidogenesis by transporting cholesterol into Leydig cells’ mitochondria, while p450scc and p450c17 are steroid-converting enzymes (24,26). MEHP decreases the production of these proteins through the PPAR pathways, thus negatively impacting male reproductive health. It has also been shown that high levels of MEHP (2,000 µM and over) inhibit the activity of these steroidogenic proteins (in addition to inhibiting 3β- and 17β-HSD enzymes specific to Leydig cell function) by inducing overloaded oxidative stress in Leydig cells, which decreases testosterone synthesis (26).
Phthalates’ interference with cholesterol in Leydig cells poses a major issue. StAR helps in the transport of cholesterol to Leydig cell mitochondria. High levels of MEHP decrease the production of StAR and reduce cholesterol transport to mitochondria, which is a necessary step for testosterone production (24). MEHP also decreases the production of p450scc, which converts cholesterol into pregnenolone, an essential step in testosterone synthesis (27). Furthermore, DBP, another phthalate, decreases fetal plasma cholesterol levels, consequently leading to low fetal testosterone synthesis, which can impair testicular descendance and secondary sexual characters (27) (Figure 1).
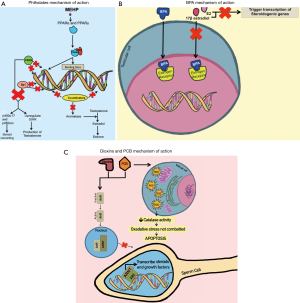
In addition to decreasing fetal plasma cholesterol levels, DBP has been shown to cause lesions in seminiferous cords and multinucleated gonocytes (27). Sertoli cells carry stem cell factor (SCF) ligands while gonocytes carry c-kit receptors required for interaction between Sertoli cells and gonocytes. DBP decreases the expression of both SCF ligands and c-kit receptors, thereby preventing interaction of Sertoli cells and gonocytes. In a similar mode of action, MEHP inhibits Sertoli cell-gonocyte interactions. It inhibits Sertoli cell proliferation by decreasing cyclin D2 mRNA production, which is involved in the G1 phase of the cell cycle (28). MEHP also causes abnormal vacuolation in Sertoli cells and disrupts the intermediate filament vimentin, which normally allows spermatogenic cells to anchor to Sertoli cells (28,29). MEHP affects spermatogenesis by decreasing the number of Sertoli cells, reducing the Sertoli cell proliferation, disrupting the interactions with gonocytes, and triggering testicular apoptosis by increased expression of the Fas ligand (28,30,31).
The negative role of MEHP on semen quality has also been documented by its passing through the blood-testis barrier (BTB), which distinguishes the basal from the adluminal component of the seminiferous epithelium (31). This separation is essential in creating a specialized microenvironment in the adluminal compartment in which germ cells can undergo meiosis and maturation (31). Tight junctions between Sertoli cells and tight junction-related proteins in these cells, including claudin-11, occludin, and zonula occludens-1 (ZO-1), contribute to BTB formation. Disruptions in these tight junctions significantly decrease the division of germ cells and the production of sperm and may lead to development of autoimmunity. MEHP greatly reduces the levels of claudin-11 and occludin mRNA expression in Sertoli cells by activating the p44/42 MAPK pathway, thereby disrupting tight junctions and thus spermatogenesis (31). BPA similarly induces reduction of occludin and ZO-1 in Sertoli cells through activation of the p44/42 MAPK pathway (31).
BPA also affects estrogen (ERα and ERβ) and androgen receptors (25,32-35). For instance, BPA competes with estrogens such as 17β-estradiol and E2, which normally bind to estrogen receptors in the testis and trigger transcription of steroidogenic genes (25,32,33,36). Normally, the 3-hydroxyl group of E2 interacts with Glu353 and Arg394 via hydrogen bonding to ERα, and the 17β-estradiol interacts with His524; the 4-hydroxyl group of BPA competitively interacts with these amino acids and thus decreases steroidogenesis (34). Additionally, the hydrophobic propane and B-ring moieties of BPA help it bind to the hydrophobic binding sites of estrogen receptors (37). BPA also interacts with ASC1 to inhibit binding of androgens to receptors, which is detrimental to prostate development (33). Another receptor that BPA interferes with is the luteinizing hormone (LH) receptor in Leydig cells (33). BPA prevents LH receptors and adenylate cyclase from coupling in Leydig cells, thus inhibiting testosterone synthesis (33).
BPA also affects sperm quality by upregulating the mRNA level of the aryl hydrocarbon receptor (AhR) repressor (37). AhR is a transcription factor whose function is to induce expression of the CYP1 gene, which encodes aromatase, an enzyme that biosynthesizes steroids. By suppressing AhR, BPA inhibits aromatase activity and steroidogenesis (37) (Figure 1). Additionally, AhR usually mediates the effects of other endocrine disruptors such as polyaromatic hydrocarbons, dioxins, and PCBs (37).
BPA also leads to the accumulation of reactive oxygen species (ROS), which affects mitochondria and the endoplasmic reticulum (ER) (38). Pathological levels of ROS damage the ER and compromise its function of protein folding, thereby triggering the unfolded protein response (UPR) (38). The UPR favors binding of glucose-regulated protein 78 (GRP78) to unfolded and misfolded proteins. This binding in turn stimulates the production of ER stress transducers protein kinase RNA-like ER kinase (PERK), inositol-requiring enzyme 1 (IRE1), and activating transcription factor 6 (ATF6) (38,39). These stress transducers collectively stimulate apoptotic factors in the testicular cells. IRE1 activates the apoptotic transcription factor Chop; ATF6 activates apoptotic genes such as BiP, GRP94, and Chop; and PERK triggers the translation of ATF4, which then drives the activation of Chop (38). All these events lead to raised ROS levels, causing a condition called oxidative stress which negatively affects spermatogenesis and semen quality (38). ROS accumulation also triggers mitochondrial apoptosis in testicular cells by activating the Fas/FasL apoptotic signaling pathway, further debilitating spermatogenesis (38,40). BPA also suppresses the Akt/mTOR pathway in testicular mitochondria, which is crucial in spermatogenesis (40).
Dioxins and PCB are two more EDCs that negatively impact semen quality using similar mechanisms of action. Dioxins and PCBs bind to the AhR, which is normally inactive (9). They activate AhR, causing it to translocate to the nucleus and dimerize with the AhR nuclear translocator (ARNT) complex (41). The ARNT complex normally binds to the xenobiotic responsive element (XRE) on target DNA to transcribe steroids and growth factors. In cases of high dioxins and PCB levels, AhR blocks the ARNT complex, leading to impaired steroidogenesis (41). Since sperm possess AhR, dioxins and PCBs interfere with these receptors and cause detrimental consequences for male reproductive health in terms of capacitation, the acrosome reaction, sperm-egg binding, and fertilization (9). Further, dioxins and PCBs inhibit Sertoli cell proliferation and the development of seminiferous tubules, which results in reduced semen production (42). Additionally, dioxins and PCBs interrupt the hypothalamic-pituitary-thyroid (HPT) axis, which is responsible for regulating hormones and steroid production (43). Disruption of the HPT axis decreases spermatogenesis, Leydig cell number, and plasma testosterone (43). PCBs specifically also produce free radicals in testicular cells that decrease catalase activity which is meant to combat oxidative stress (43). PCBs are known to inhibit gene expression of steroidogenic enzymes by binding to AhR and androgen receptors, thus resulting in oxidative stress and ultimately apoptosis (43,44) (Figure 1).
Against argument
Though there is much evidence to support the assertion that EDCs are detrimental to semen quality and overall male reproductive health, there are some who claim that current studies on the effects of EDCs are inaccurate or unrealistic in their findings. One such concern is that current studies do not report the body mass indexes (BMIs) of their subjects, which could be problematic because BMI and body fat percentages have been correlated with variations in both semen quality and levels of reproductive hormones (12). Past work, however, has addressed this concern in different ways. For example, one study compensated for differences among subjects by normalizing weight in terms of AGD so that individuals could be compared to each other on the basis of an anogenital index (AGI), and results showed that AGD was shorter in boys whose mothers had experienced heightened exposure to phthalates (19). It was acknowledged in this study that humans have greater potential sensitivity to toxins because of their slower metabolic rates as compared to rats and other rodents that are often used as test subjects in similar studies (19). It follows that the subject of varying metabolic rates among humans must account for some of the different responses to EDC exposure, and this is an especially important point considering that metabolic rates can vary greatly between individuals with different weights and body fat percentages. To address this issue, one study determined the ratio of metabolized EDC as compared to the amount of un-metabolized chemical (45). In this work, it was found that overweight subjects had lower levels of the metabolized form of di-2-ethylhexyl (DEHP)—MEHP—as compared to normal and underweight individuals (45). These results are important not only because they call attention to the higher health risks associated with overweight exposure to EDCs, but because they also compensate for the confounding effects of differing weights among test subjects. Another concern regarding these studies is that they do not report on the confounding effects of smoking (12). Though past studies, such as the one regarding MEHP, have taken note of smoking versus non-smoking test subjects, the results of these studies are still limited because they fail to take into account the fact that many of these same test subjects are also on prescription medications that could interfere with the results of the EDC exposure (45). Such concerns limit the findings of studies that report on the effects of EDCs, but the mounting amount of evidence still seems to point toward the direction of these chemicals as harmful agents against human hormonal systems.
Conclusions
Overall, the evidence on EDC exposure points toward the negative effects they have on humans. The rise of EDCs in the environment as well as the simultaneous rise of male reproductive disorders and infertility calls attention to the idea that EDCs may be responsible for the decline in male reproductive health (2,5,6). EDCs have been incorporated into a wide array of consumables and products (foods, water, plastics, shampoos, clothes, toothpastes, soaps, fertilizers, paper, textiles, carpets, utensils, bedding, toy, cosmetics, deodorant, etc.), which puts all people at greater risk for exposure in daily life (2-4). Additionally, there is an increasing amount of research to suggest that male children are more likely to develop reproductive disorders in response to neonatal and especially prenatal exposure; such exposures are even more likely to occur now with the increasing prevalence of EDCs in general consumer goods (1,7,17-19). In order to tackle this issue, some countries have already established regulations to remove EDCs from everyday products. For example, as of January 2015, the French parliament outlawed the use of BPA in any containers that come into contact with food. France has also outlawed the use of phthalate DEHP in toys and products related to childcare. In the United States, legislation such as the Toxic Substances Control Act, the Food Quality Protection Act of 1996, and the Safe Drinking Water Act of 1996 gives the Environmental Protection Agency (EPA) the power to evaluate and place regulations upon substances that may act as endocrine disruptors. Similarly, the Endocrine Disruptor Screening Program introduced by the EPA in 1998 has allowed for the testing of over 85,000 chemicals. A comprehensive list of EDCs and their potential effects on male reproductive health in relevance to the published studies are listed in Table 2. Clearly, the EDC issue has inspired much legal change, but it is still necessary that more countries adopt these regulations and that a wider range of chemicals are screened for the potential enactment of new legislation. Unless more laws are put in place to control the increase of EDCs in the environment, humans and animals alike could be facing the consequences of decreased health and fertility. The way in which EDCs have been shown to negatively affect semen parameters and reproductive health in male subjects is just one of the many ways in which EDCs have been shown to be a detriment to humans. These facts warrant the use of alternatives to EDCs.
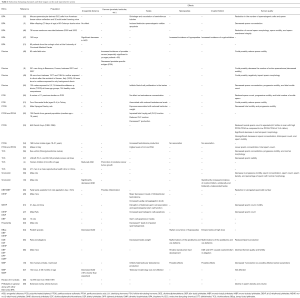
Full table
Acknowledgements
None.
Footnote
Conflicts of Interest: The authors have no conflicts of interest to declare.
References
- Marcoccia D, Pellegrini M, Fiocchetti M, et al. Food components and contaminants as (anti)androgenic molecules. Genes Nutr 2017;12:6. [Crossref] [PubMed]
- Joensen UN, Bossi R, Leffers H, et al. Do perfluoroalkyl compounds impair human semen quality? Environ Health Perspect 2009;117:923-7. [Crossref] [PubMed]
- Vilela J, Hartmann A, Silva EF, et al. Sperm impairments in adult vesper mice (Calomys laucha) caused by in utero exposure to bisphenol A. Andrologia 2014;46:971-8. [Crossref] [PubMed]
- Zhu W, Zhang H, Tong C, et al. Environmental Exposure to Triclosan and Semen Quality. Int J Environ Res Public Health 2016;13:224. [Crossref] [PubMed]
- Nordkap L, Joensen UN, Blomberg Jensen M, et al. Regional differences and temporal trends in male reproductive health disorders: semen quality may be a sensitive marker of environmental exposures. Mol Cell Endocrinol 2012;355:221-30. [Crossref] [PubMed]
- Mendiola J, Stahlhut RW, Jørgensen N, et al. Shorter anogenital distance predicts poorer semen quality in young men in Rochester, New York. Environ Health Perspect 2011;119:958-63. [Crossref] [PubMed]
- Fisher JS. Environmental anti-androgens and male reproductive health: focus on phthalates and testicular dysgenesis syndrome. Reproduction 2004;127:305-15. [Crossref] [PubMed]
- Lafuente R, García-Blàquez N, Jacquemin B, et al. Outdoor air pollution and sperm quality. Fertil Steril 2016;106:880-96. [Crossref] [PubMed]
- Faure AC, Viel JF, Bailly A, et al. Evolution of sperm quality in men living in the vicinity of a municipal solid waste incinerator possibly correlated with decreasing dioxins emission levels. Andrologia 2014;46:744-52. [Crossref] [PubMed]
- Toshima H, Suzuki Y, Imai K, et al. Endocrine disrupting chemicals in urine of Japanese male partners of subfertile couples: a pilot study on exposure and semen quality. Int J Hyg Environ Health 2012;215:502-6. [Crossref] [PubMed]
- Auger J, Kunstmann JM, Czyglik F, et al. Decline in semen quality among fertile men in Paris during the past 20 years. N Engl J Med 1995;332:281-5. [Crossref] [PubMed]
- Swan SH, Elkin EP, Fenster L. The question of declining sperm density revisited: an analysis of 101 studies published 1934-1996. Environ Health Perspect 2000;108:961-6. [Crossref] [PubMed]
- Rolland M, Le Moal J, Wagner V, et al. Decline in semen concentration and morphology in a sample of 26,609 men close to general population between 1989 and 2005 in France. Hum Reprod 2013;28:462-70. [Crossref] [PubMed]
- Abdelouahab N, Ainmelk Y, Takser L. Polybrominated diphenyl ethers and sperm quality. Reprod Toxicol 2011;31:546-50. [Crossref] [PubMed]
- Aneck-Hahn NH, Schulenburg GW, Bornman MS, et al. Impaired semen quality associated with environmental DDT exposure in young men living in a malaria area in the Limpopo Province, South Africa. J Androl 2007;28:423-34. [Crossref] [PubMed]
- Chen M, Tang R, Fu G, et al. Association of exposure to phenols and idiopathic male infertility. J Hazard Mater 2013;250-251:115-21. [Crossref] [PubMed]
- Doyle TJ, Bowman JL, Windell VL, et al. Transgenerational effects of di-(2-ethylhexyl) phthalate on testicular germ cell associations and spermatogonial stem cells in mice. Biol Reprod 2013;88:112. [Crossref] [PubMed]
- Hallmark N, Walker M, McKinnell C, et al. Effects of monobutyl and di(n-butyl) phthalate in vitro on steroidogenesis and Leydig cell aggregation in fetal testis explants from the rat: comparison with effects in vivo in the fetal rat and neonatal marmoset and in vitro in the human. Environ Health Perspect 2007;115:390-6. [Crossref] [PubMed]
- Swan SH, Main KM, Liu F, et al. Decrease in anogenital distance among male infants with prenatal phthalate exposure. Environ Health Perspect 2005;113:1056-61. Erratum in: Environ Health Perspect 2005;113:A583. [Crossref] [PubMed]
- Virtanen HE, Bjerknes R, Cortes D, et al. Cryptorchidism: classification, prevalence and long-term consequences. Acta Paediatr 2007;96:611-6. [Crossref] [PubMed]
- Tarapore P, Ying J, Ouyang B, et al. Exposure to bisphenol A correlates with early-onset prostate cancer and promotes centrosome amplification and anchorage-independent growth in vitro. PLoS One 2014;9. [Crossref] [PubMed]
- Jurewicz J, Hanke W, Radwan M, et al. Environmental factors and semen quality. Int J Occup Med Environ Health 2009;22:305-29. [Crossref] [PubMed]
- Lovekamp-Swan T, Jetten AM, Davis BJ. Dual activation of PPARalpha and PPARgamma by mono-(2-ethylhexyl) phthalate in rat ovarian granulosa cells. Mol Cell Endocrinol 2003;201:133-41. [Crossref] [PubMed]
- Gunnarsson D, Leffler P, Ekwurtzel E, et al. Mono-(2-ethylhexyl) phthalate stimulates basal steroidogenesis by a cAMP-independent mechanism in mouse gonadal cells of both sexes. Reproduction 2008;135:693-703. [Crossref] [PubMed]
- Akingbemi BT, Ge R, Klinefelter GR, et al. Phthalate-induced Leydig cell hyperplasia is associated with multiple endocrine disturbances. Proc Natl Acad Sci U S A 2004;101:775-80. [Crossref] [PubMed]
- Zhao Y, Ao H, Chen L, et al. Mono-(2-ethylhexyl) phthalate affects the steroidogenesis in rat Leydig cells through provoking ROS perturbation. Toxicol In Vitro 2012;26:950-5. [Crossref] [PubMed]
- Barlow NJ, Phillips SL, Wallace DG, et al. Quantitative changes in gene expression in fetal rat testes following exposure to di(n-butyl) phthalate. Toxicol Sci 2003;73:431-41. [Crossref] [PubMed]
- Li LH, Jester WF Jr, Laslett AL, et al. A single dose of Di-(2-ethylhexyl) phthalate in neonatal rats alters gonocytes, reduces sertoli cell proliferation, and decreases cyclin D2 expression. Toxicol Appl Pharmacol 2000;166:222-9. [Crossref] [PubMed]
- Tay TW, Andriana BB, Ishii M, et al. Disappearance of vimentin in Sertoli cells: a mono(2-ethylhexyl) phthalate effect. Int J Toxicol 2007;26:289-95. [Crossref] [PubMed]
- Park JD, Habeebu SS, Klaassen CD. Testicular toxicity of di-(2-ethylhexyl)phthalate in young Sprague-Dawley rats. Toxicology 2002;171:105-15. [Crossref] [PubMed]
- Chiba K, Kondo Y, Yamaguchi K, et al. Inhibition of claudin-11 and occludin expression in rat Sertoli cells by mono-(2-ethylhexyl) phthalate through p44/42 mitogen-activated protein kinase pathway. J Androl 2012;33:368-74. [Crossref] [PubMed]
- Yin L, Dai Y, Cui Z, et al. The regulation of cellular apoptosis by the ROS-triggered PERK/EIF2α/chop pathway plays a vital role in bisphenol A-induced male reproductive toxicity. Toxicol Appl Pharmacol 2017;314:98-108. [Crossref] [PubMed]
- Wetherill YB, Akingbemi BT, Kanno J, et al. In vitro molecular mechanisms of bisphenol A action. Reprod Toxicol 2007;24:178-98. [Crossref] [PubMed]
- Kitamura S, Suzuki T, Sanoh S, et al. Comparative study of the endocrine-disrupting activity of bisphenol A and 19 related compounds. Toxicol Sci 2005;84:249-59. [Crossref] [PubMed]
- Lee HJ, Chattopadhyay S, Gong EY, et al. Antiandrogenic effects of bisphenol A and nonylphenol on the function of androgen receptor. Toxicol Sci 2003;75:40-6. [Crossref] [PubMed]
- Kuiper GG, Carlsson B, Grandien K, et al. Comparison of the ligand binding specificity and transcript tissue distribution of estrogen receptors alpha and beta. Endocrinology 1997;138:863-70. [Crossref] [PubMed]
- Bonefeld-Jørgensen EC, Long M, Hofmeister MV, et al. Endocrine-disrupting potential of bisphenol A, bisphenol A dimethacrylate, 4-n-nonylphenol, and 4-n-octylphenol in vitro: new data and a brief review. Environ Health Perspect 2007;115 Suppl 1:69-76. [Crossref] [PubMed]
- Li Y, Burns KA, Arao Y, et al. Differential estrogenic actions of endocrine-disrupting chemicals bisphenol A, bisphenol AF, and zearalenone through estrogen receptor α and β in vitro. Environ Health Perspect 2012;120:1029-35. [Crossref] [PubMed]
- Liu ZW, Zhu HT, Chen KL, et al. Protein kinase RNA-like endoplasmic reticulum kinase (PERK) signaling pathway plays a major role in reactive oxygen species (ROS)-mediated endoplasmic reticulum stress-induced apoptosis in diabetic cardiomyopathy. Cardiovasc Diabetol 2013;12:158. [Crossref] [PubMed]
- Quan C, Wang C, Duan P, et al. Prenatal bisphenol a exposure leads to reproductive hazards on male offspring via the Akt/mTOR and mitochondrial apoptosis pathways. Environ Toxicol 2017;32:1007-23. [Crossref] [PubMed]
- Mocarelli P, Gerthoux PM, Needham LL, et al. Perinatal exposure to low doses of dioxin can permanently impair human semen quality. Environ Health Perspect 2011;119:713-8. [Crossref] [PubMed]
- Mínguez-Alarcón L, Sergeyev O, Burns JS, et al. A Longitudinal Study of Peripubertal Serum Organochlorine Concentrations and Semen Parameters in Young Men: The Russian Children's Study. Environ Health Perspect 2017;125:460-6. [PubMed]
- Phillips KP, Tanphaichitr N. Human exposure to endocrine disrupters and semen quality. J Toxicol Environ Health B Crit Rev 2008;11:188-220. [Crossref] [PubMed]
- Yurdakok B, Tekin K, Daskin A, et al. Effects of polychlorinated biphenyls 28, 30 and 118 on bovine spermatozoa in vitro. Reprod Domest Anim 2015;50:41-7. [Crossref] [PubMed]
- Yaghjyan L, Carlsson NP, Ghita GL, et al. Associations of individual characteristics and lifestyle factors with metabolism of di-2-ethylhexyl phthalate in NHANES 2001-2012. Environ Res 2016;149:23-31. [Crossref] [PubMed]
- Fernández MF, Arrebola JP, Jiménez-Díaz I, et al. Bisphenol A and other phenols in human placenta from children with cryptorchidism or hypospadias. Reprod Toxicol 2016;59:89-95. [Crossref] [PubMed]
- Miao M, Yuan W, He Y, et al. In utero exposure to bisphenol-A and anogenital distance of male offspring. Birth Defects Res A Clin Mol Teratol 2011;91:867-72. [Crossref] [PubMed]
- Ishiniwa H, Sakai M, Tohma S, et al. Dioxin pollution disrupts reproduction in male Japanese field mice. Ecotoxicology 2013;22:1335-47. [Crossref] [PubMed]
- Mocarelli P, Gerthoux PM, Patterson DG Jr, et al. Dioxin exposure, from infancy through puberty, produces endocrine disruption and affects human semen quality. Environ Health Perspect 2008;116:70-7. [Crossref] [PubMed]
- Meeker JD, Hauser R. Exposure to polychlorinated biphenyls (PCBs) and male reproduction. Syst Biol Reprod Med 2010;56:122-31. [Crossref] [PubMed]
- Zhao B, Li L, Liu J, et al. Exposure to perfluorooctane sulfonate in utero reduces testosterone production in rat fetal Leydig cells. PLoS One 2014;9. [Crossref] [PubMed]
- Toft G, Jönsson BA, Bonde JP, et al. Perfluorooctane Sulfonate Concentrations in Amniotic Fluid, Biomarkers of Fetal Leydig Cell Function, and Cryptorchidism and Hypospadias in Danish Boys (1980-1996). Environ Health Perspect 2016;124:151-6. [PubMed]
- Vested A, Ramlau-Hansen CH, Olsen SF, et al. Associations of in utero exposure to perfluorinated alkyl acids with human semen quality and reproductive hormones in adult men. Environ Health Perspect 2013;121:453-8. [PubMed]
- Governini L, Guerranti C, De Leo V, et al. Chromosomal aneuploidies and DNA fragmentation of human spermatozoa from patients exposed to perfluorinated compounds. Andrologia 2015;47:1012-9. [Crossref] [PubMed]
- Hwang J, Suh SS, Chang M, et al. Effects of triclosan on reproductive prarmeters and embryonic development of sea urchin, Strongylocentrotus nudus. Ecotoxicol Environ Saf 2014;100:148-52. [Crossref] [PubMed]
- Derouiche S, Mariot P, Warnier M, et al. Activation of TRPA1 Channel by Antibacterial Agent Triclosan Induces VEGF Secretion in Human Prostate Cancer Stromal Cells. Cancer Prev Res (Phila) 2017;10:177-87. [Crossref] [PubMed]
- Lassen TH, Frederiksen H, Kyhl HB, et al. Prenatal Triclosan Exposure and Anthropometric Measures Including Anogenital Distance in Danish Infants. Environ Health Perspect 2016;124:1261-8. [Crossref] [PubMed]
- Shono T, Suita S, Kai H, et al. Short-time exposure to vinclozolin in utero induces testicular maldescent associated with a spinal nucleus alteration of the genitofemoral nerve in rats. J Pediatr Surg 2004;39:217-9; discussion 217-9. [Crossref] [PubMed]
- Cowin PA, Gold E, Aleksova J, et al. Vinclozolin exposure in utero induces postpubertal prostatitis and reduces sperm production via a reversible hormone-regulated mechanism. Endocrinology 2010;151:783-92. [Crossref] [PubMed]
- Doyle TJ, Bowman JL, Windell VL, et al. Transgenerational effects of di-(2-ethylhexyl) phthalate on testicular germ cell associations and spermatogonial stem cells in mice. Biol Reprod 2013;88:112. [Crossref] [PubMed]
- Bhattacharya N, Dufour JM, Vo MN, et al. Differential effects of phthalates on the testis and the liver. Biol Reprod 2005;72:745-54. [Crossref] [PubMed]
- Bowman CJ, Barlow NJ, Turner KJ, et al. Effects of in utero exposure to finasteride on androgen-dependent reproductive development in the male rat. Toxicol Sci 2003;74:393-406. [Crossref] [PubMed]
- Wang YX, Zeng Q, Sun Y, et al. Phthalate exposure in association with serum hormone levels, sperm DNA damage and spermatozoa apoptosis: A cross-sectional study in China. Environ Res 2016;150:557-65. [Crossref] [PubMed]
- Annamalai J, Namasivayam V. Endocrine disrupting chemicals in the atmosphere: Their effects on humans and wildlife. Environ Int 2015;76:78-97. [Crossref] [PubMed]
- Kumar N, Srivastava S, Roy P. Impact of low molecular weight phthalates in inducing reproductive malfunctions in male mice: Special emphasis on Sertoli cell functions. Gen Comp Endocrinol 2015;215:36-50. [Crossref] [PubMed]
- McKinnell C, Mitchell RT, Morris K, et al. Perinatal germ cell development and differentiation in the male marmoset (Callithrix jacchus): similarities with the human and differences from the rat. Hum Reprod 2013;28:886-96. [Crossref] [PubMed]
- Carlsen E, Giwercman AJ, Keiding N, et al. Decline in semen quality from 1930 to 1991. Ugeskr Laeger 1993;155:2530-5. [PubMed]
- Jenardhanan P, Panneerselvam M, Mathur PP. Effect of environmental contaminants on spermatogenesis. Semin Cell Dev Biol 2016;59:126-40. [Crossref] [PubMed]
- Jurewicz J, Hanke W. Exposure to phthalates: reproductive outcome and children health. A review of epidemiological studies. Int J Occup Med Environ Health 2011;24:115-41. [Crossref] [PubMed]