Lymph node imaging of pediatric renal and suprarenal malignancies
Introduction
Abdominal masses in children can result from a number of etiologies ranging from benign conditions to malignant tumors. Most pediatric abdominal masses arise from the kidney and are benign (1). In fact, a pediatric renal mass is more likely to be hydronephrosis, or if solid, congenital mesoblastic nephroma (1,2). Nevertheless, abdominal masses, which are often detected incidentally by the child’s caretaker, may be malignant neoplasms. Neuroblastomas are the most common extracranial malignancy in children presenting most frequently in the adrenal glands. While relatively more rare, pediatric renal cancer makes up approximately 6% to 7% of all pediatric cancers (1,3,4) including Wilms’ tumor (WT), renal cell carcinoma (RCC), rhabdoid tumor, clear cell sarcoma, and other less frequent pathologies.
Many of these conditions can present as locally-advanced or with metastases to lymph nodes, lungs, brain and bones. Advanced stage disease often requires multimodal treatments including nephrectomy, lymph node dissection, chemotherapy, and radiotherapy (2). Therefore, imaging to effectively gauge the extent of disease is vital to assessing patient prognosis and planning treatment.
There are different considerations for which imaging modality to choose for a pediatric patient as compared to an adult. For abdominal imaging, abdominal ultrasound is generally the first imaging investigation pursued, but most guidelines recommend additional imaging such as computed tomography (CT) or magnetic resonance imaging (MRI) for additional detail (5). When working with pediatric patients, however, patient cooperativity during exam must be addressed. Pediatric patients often require assistance or sedation to remain motionless during extending imaging studies (i.e., MRI) and their cooperation can vary widely depending on the age of the child (5). CT imaging utilizes ionizing radiation, which is more concerning for development of radiation-associated cancers in pediatric patients (6). MRI is attractive because it does not expose the patient to ionization radiation. Moreover, the relatively smaller size of pediatric patients allows for use of whole body MRI (WBMRI). Given the risk of widespread metastases in pediatric renal and suprarenal cancer, WBMRI offers an attractive option for complete imaging assessment of pediatric patients with these malignancies (4).
Imaging of lymph nodes in pediatric patients poses additional challenges. There is lacking standardization for what constitutes abnormally enlarged lymph nodes in children of different ages and radiologists frequently employ their personal interpretations when classifying nodes as suspicious or benign (7).
In this review, we will examine the role of lymph node involvement (LNI) in prognosis and treatment for pediatric renal and suprarenal cancers, specifically WT, neuroblastoma, and RCC. Through this lens, we will report on research regarding imaging detection of LNI in these cancers how these imaging studies impact treatment decisions.
WT
WT is the most common primary renal malignancy in pediatric patients (5,8). There are an estimated 650 cases of WT in North America each year (3,9). This tumor arises unilaterally in most cases or, rarely, bilaterally (approximately 5% of cases). These children present at a mean age of 3.5–4.0 years (2,8). The WT1 gene is a tumor suppressor gene located on chromosome 11p (2). Alterations affecting this gene can occur in multiple hereditary syndromes such as WAGR (WT, aniridia, and growth retardation) syndrome, Denys-Drash (gonadal dysgenesis, nephropathy, and WT) syndrome, or Beckwith-Wiedemann syndrome (macroglossia, macrosomia, microcephaly, midline abdominal wall defects, hemihypertrophy, neonatal hypoglycemia, and WT) (2). More often, WTs are first detected incidentally by a parent as an abdominal mass or can present with hypertension in up to 65% of cases (2,10,11).
Over the last 20–30 years, prognosis of patients with WT has dramatically improved to 5-year survival rates of greater than 90% due to improvements in multimodal treatment regimens and more sensitive methods of detection (12,13). Despite these developments, patients with advanced stage disease have diminished 5-year survival rates (approximately 75%) and undergo more rigorous treatment regimens that carry significant morbidity (14). In long-term follow-up, nearly one quarter of patients suffer from chronic health problems by 25 years after initial WT treatment (12).
Treatment algorithms published by consensus groups such as the Society of Paediatric Oncology (SIOP) and the National Wilms Tumor Study (NWTS) detail use of multimodal therapy to bolster efficacy of surgical excision. Chemotherapy and radiotherapy regimens intensify as WT stage increases (15). In fact, SIOP recommends neoadjuvant chemotherapy for advanced stage WT. This has shown to reduce surgical morbidity via tumor shrinkage but has produced equivocal effects on survival as compared to adjuvant chemotherapy (16).
Involvement of lymph nodes in WT is a marker for advanced stage disease (Table 1) whether they are regional (stage III) or non-regional (stage IV) nodal metastases (14). Approximately 41% of stage III patients are characterized as such based solely on nodal involvement. Due to the associated risk for postsurgical recurrence and mortality, retroperitoneal lymph node dissection is strongly recommended for all WT resections (17). Raval et al. (9) demonstrated that lymph node dissection was associated with improved overall survival (OS) as compared to patients who did not undergo lymph node dissection. In a study of 3,409 patients in the NWTS, Kieran et al. (18) found that stage II WT patients especially benefited from nodal dissection due to the potential for under-sampling and missed disease. For stage I patients who often do not receive adjuvant therapy, Shamberger et al. determined that retroperitoneal nodal dissection also reduced the risk of recurrence (19). While there is no standard number of nodes to sample during WT surgeries, some studies have shown that increasing the number of nodes (many have used >7 nodes as a threshold value) improves detection of nodal involvement (18,20), although nodal positivity was shown to negatively impact survival (9,21).
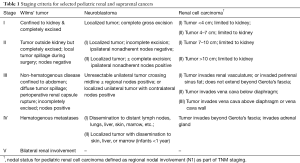
Full table
Despite the improved staging accuracy and potential survival benefit associated with lymph node dissection in WT surgeries, patients are routinely under-sampled. In the series presented by Nanda et al. (20), 75% of patients had fewer than seven nodes sampled and other trials have reported no lymph node sampling in 9% of patients (9,17,19). When lymph node dissection is performed, intraoperative discrimination between benign and malignant lymph nodes is relatively poor. One surgical series reported a false-negative rate of 31.3%, a false-positive rate of 18.1%, and a positive predictive value of 56.4% (22). This would support a need for increased numbers of nodes sampled during dissection (as detailed above). However, with increased nodal sampling there is increased risk of surgical morbidity and complications. Given these challenges and treatment implications of detecting positive lymph nodes in WT cases, there is certainly a need to improve detection of LNI and if possible, do so in anticipation of surgery.
Current preoperative imaging protocols for WT are well-established. When an intraabdominal mass is detected in a pediatric patient, abdominal ultrasound is first utilized in an attempt to determine the origin of the mass, gauge whether it is cystic or solid, and visualize possible tumor thrombi (23). If ultrasound findings are suspicious for a solid renal mass, it is advised to add a cross-sectional imaging of the abdomen for improved detection of tumor size, invasion, contralateral kidney assessment, metastases, and LNI (5). If there is concern for metastasis, chest X-ray or bone scan should be ordered, as well.
To quantify the added value of cross-sectional imaging after US, McDonald et al. performed a retrospective review of 52 patients with histologically-proven WT, of the cases reviewed, 45% had additional WT findings on CT or MRI including two patients with bilateral tumors (stage V disease), three patients with abdominal LNI, and two with tumor rupture/hemorrhage. When correlated with histology, five patients were determined to have false-negative findings on added cross-sectional imaging that included two cases of inferior vena cava (IVC) thrombi and three cases of enlarged abdominal lymph nodes. Six patients were determined to have false-positive findings that included one case of abdominal lymph node enlargement, one case of IVC thrombus, two cases of adjacent invasion, and one case of liver metastasis. While the study authors contend that the cross-sectional imaging does provide valuable additional diagnostic information, the lack of diagnostic accuracy for detection of lymph node invasion is problematic. Furthermore, despite widespread use of cross-sectional imaging for work-up of WT cases, there is a lack of established imaging criteria by which diagnosis of lymph node invasion in WT cases is made.
In a large, 25-year retrospective review of WT patients, Lubahn et al. (7) sought to determine the association between enlarged lymph nodes on abdominal CT and final pathology. They identified 52 patients who underwent preoperative abdominal CT without neoadjuvant chemotherapy prior to surgery. All CT scans were performed with slice thickness ≤5 mm. Ten patients (19.2%) had pathologically-confirmed positive lymph nodes. When correlated back to preoperative CT imaging, the median diameter of the largest regional lymph node in those cases was 8.5 mm (range, 3–11 mm), but median diameter of largest regional lymph node in patients without LNI was 6.0 mm (range, 2–15 mm), P=0.089. A median of five lymph nodes were surgically resected and the authors found no statistically significant correlation between patient age and lymph node size. Using a cut-off of 7.0 mm for enlarged lymph nodes measured on CT imaging, the authors determined the sensitivity and specificity for detecting lymph node invasion to be 70.0% and 57.1%, respectively, and a negative predictive value of 89.0%. Overall, CT imaging was found to have moderate-to-poor diagnostic ability for lymph node invasion with an area under the curve of 0.67 (95% CI, 0.48–0.87, P=0.09). Other studies also call into question the ability of CT to stage WT cases by finding high rates of incorrect staging on final pathology due to missed preoperative detection of capsular penetration or reported lymph node enlargement (24). Furthermore, without specific definitions for lymph node enlargement in these cases, it is difficult to assess the true ability of CT imaging to diagnose lymph node invasion.
There is clearly a need for standardized criteria for diagnosing nodal invasion in WT cases and exploration of contemporary imaging modalities to improve overall nodal visualization. As noted above, MRI is an attractive option because it offers improved spatial resolution and lack of ionizing radiation exposure (25). When examining multiparametric MRI data from pediatric patients with abdominal lesions, Meeus et al. (26) were able to use apparent diffusion coefficient (ADC) mapping and the intravoxel incoherent motion (IVIM) model to distinguish between different abdominal tumor histologic types. Not only could MRI discriminate between benign and malignant tumors, it also was able to specifically identify WT apart from neuroblastomas based on diffusion and perfusion characteristics. When examining only WT cases, Hales et al. (25) were able to use ADC mapping to differentiate between WT subtypes (i.e., blastemal, epithelial, stromal, and regressive) and track changes in viable tumor tissue following chemotherapy. Similar to preoperative staging, preoperative assessment of WT subtype has important implications in prognostic factors such as recurrence and survival, and potentially allows for necessary adjustments to neoadjuvant therapy.
While MRI shows promise in the preoperative characterization of WT, there are no studies that look specifically at detection of lymph node invasion with MRI. Moreover, investigations of positron emission tomography (PET)/CT or MRI have focused on characterizing primary WT sites and response to treatment, but not nodal invasion (27,28). As advanced imaging modalities continue to evolve and neoadjuvant chemotherapies are further developed, there is opportunity to investigate image-based staging of WT, especially regarding lymph node invasion.
Neuroblastoma (NB)
NB is the most common extracranial malignancy in children and in general, NB is the third most common pediatric malignancy behind leukemia and central nervous system tumors (29). NBs have an incidence of ten per one million live births (2).
NB arises from neural crest cells that compose the adrenal medulla as well as sympathetic ganglia. The vast majority of NBs arise in the retroperitoneum within the adrenal gland and sympathetic ganglia (30). There is a wide spectrum of clinical presentations and clinical courses in regard to NBs due to varied locations and tumor biology (31). Of note, amplification of the MYCN gene confers a worse prognosis in NB (32).
Appropriate staging in NB is critical to determining treatment and prognosis (Table 1). The International Neuroblastoma Staging System (INSS) was developed to ascertain risk and assist in clinical decision making as specific treatment protocols exist depending on NB stage. According to the INSS, lymph node invasion can occur at any stage of disease, but their position relative to the primary site determines more advanced stage disease. Whereas stage I and II disease may have LNI ipsilateral to the tumor, stage III disease may be defined by contralateral LNI. Stage IV disease is defined as any primary tumor with dissemination to distant lymph nodes, bone, bone marrow, liver, skin and/or other organs. Approximately 60% of patients present with metastatic NB including invasion of lymph nodes while hematogenous spread to the lungs or brain is far less common (33). In a meta-analysis, Morgenstern et al. (34) analyzed the International Neuroblastoma Risk Group Database and found 2,250 patients with stage IV disease and complete data from 1990–2002. One hundred fourty-six (6.5%) were found to have 4N disease (confined to distant lymph nodes) and more than half of these patents were less than 18 months of age. The authors discovered an inverse correlation between MYCN-amplification status and stage 4N NB disease. Importantly, stage 4N disease was found to be independently associated with improved outcomes vs. solid organ metastasis after adjusting for age and MYCN status (34). This is in congruence with additional case series and reports that have found that metastatic disease limited to lymph nodes or 4N disease may potentially have superior outcomes compared to others with extra-nodal NB (34). Considerations for a diagnosis of stage 4N NB may be that these patients may necessitate less intense treatment regimens.
Given the propensity for NBs to spread beyond a primary site and the importance of precise localization of LNI, multimodal imaging studies are generally employed for accurate staging and diagnosis. Identification of LNI in NB not only informs selection of multimodal treatments, but also guides surgical approach to maximize removal of malignant lesions. This can prove beneficial for patient outcomes as Mullassery et al. (35) found a significant advantage in those stage IV patients who have gross total resection versus those who have subtotal resection. It can be postulated that preoperative localization of clinically positive lymph nodes could provide a more complete resection that would yield a superior prognosis.
Treatment regimens for NB are dependent on risk stratification, which is based on a combination of patient age, stage, MYCN mutational status, DNA index, and histopathologic findings (30). Patients with low-risk disease may avoid chemotherapy altogether staving off the significant morbidities and long-term toxicities associated with systemic treatments in children. Survival is >90% with surgical intervention for low-risk NB, >90% with combined surgical and chemotherapeutic intervention for intermediate-risk NB, but can fall to <30% despite intense multimodal therapy in high-risk NB, especially those with amplification of MYCN (30). Younger patients (age one or less) typically have better outcomes in terms of survival compared to older patients likely due to improved tumor biology at that time point. Moreover, improved survival is seen with non-adrenal primary NB (2).
Imaging work-up for NB, which often presents as an incidentally-found abdominal mass, generally begins with abdominal ultrasound and cross-sectional CT. More recently, MRI has demonstrated equivalence and even indications of superiority to CT for delineation of primary tumor, metastatic involvement, and post-treatment NB surveillance (36,37). However, MRI may lack sensitivity for detection of smaller involved lymph nodes (30). In general, both CT and MRI have low positive predictive value for LNI, but the inclusion of additional pulse sequences, such as diffusion weighted imaging, as part of a multiparametric MRI may improve positive lymph node detection (4). Use of ADC mapping values calculated from diffusion-weighted imaging with background body signal suppression (DWIBS) has been shown to differentiate NB from other abdominal lesions, both benign and malignant, and gauge response to chemotherapy (38,39).
Unlike WT or pediatric RCC, NB is identifiable by its associated hypersecretion of urinary metabolites: vanillylmandelic acid, homovanillic acid, and dopamine (40). Scintigraphic studies make use of this metabolic activity for imaging. Metaiodobenzylguanidine (MIBG) is taken up by neuroblastic cells and when combined with a radiotracer (such as 123I), it can concentrate allowing for scintigraphic visualization in neuroblastoma primary sites and metastases (37,41). Pfluger et al. (37) combined 123I MIBG and MRI to improve sensitivity and specificity of primary NB detection to 99% and 95%, respectively. Regarding detection of LNI, MRI was falsely-positive in two cases that were subsequently ruled out on 123I MIBG scintigraphy.
131I MIBG is not only utilized for imaging, but also has a likely therapeutic benefit due to its toxic uptake in neuroblastoma cells (42). It has been shown to be particularly effective for treatment of relapsed disease (41). A comparative study between 131I MIBG and 123I MIBG radiotracers suggest that 123I MIBG is preferable for imaging studies due to greater sensitivity, image quality, and decreased toxicity (43).
PET imaging also makes use of the significant metabolic activity exhibited by NB tumors and can be useful for NB imaging in cases in which NB does not express sufficient norepinephrine transporter for MIBG uptake or when cost of and/or access to MIBG radiotracer is prohibitive (44). In a comparative study of fluorine-18 fluorodeoxyglucose (18F-FDG) PET/CT versus 131I MIBG scintigraphy by Dhull et al. (44), PET/CT was far more sensitive for detection of lymph node metastases. PET/CT detected 22 nodal lesions versus only five seen on 131I MIBG scintigraphy, P=0.001. In a case study by Colavolpe et al. (45), FDG PET/CT demonstrated utility in monitoring NB following treatment because these tumors can lose MIBG avidity resulting in falsely negative findings. These findings were also seen in the study by Dhull et al. (44).
Alternative PET tracers, such as 18F-fluoro-dihydroxyphenlalanine (18F-DOPA) have been investigated with FDG PET/CT for NB staging. Liu et al. (46) measured pre-treatment volumetric parameters on FDG PET/CT and F-DOPA PET/CT to determine associations with negative prognostic features. For patients with lymph node metastases, F-DOPA PET/CT demonstrated higher uptake values in primary tumors while FDG PET/CT did not successfully differentiate between patients with or without LNI. The study did not address uptake at the lymph nodes specifically.
Developments in MRI, scintigraphy, and PET imaging are improving NB detection and staging, while informing treatment decisions to impact patient survival and minimize unnecessary morbidity. The unique metabolic characteristics of NB provide strong opportunity to continue improving non-invasive biomarkers of lymph node invasion and other metastatic sites via advanced imaging modalities.
Pediatric RCC
RCC is rare in the pediatric population, accounting for <5% of all pediatric renal tumors (47). Presenting symptoms include frank hematuria, flank pain, and/or a palpable abdominal mass. These tumors usually occur in the second decade of life with age being strongly associated with risk of RCC diagnosis (48). It is important to differentiate RCC from other tumors; hematuria is uncommon in WT while older children and teenagers tend to have RCC. Additionally, calcification seen on imaging is suggestive of RCC, noted in up to 53% of patients (47). In contrast to adults where up to 50% of RCC are found incidentally, up to 88% are diagnosed after evaluation of the aforementioned symptoms in the pediatric population (49).
RCC originates from the epithelial cells of the renal tubules and includes a subset of morphologies including papillary, clear cell, chromophobe, and oncocytoma (4). The papillary subtype is most common (20–50%) in the pediatric population (47). RCC is often associated with a chromosome translocation involving the TFE gene located on Xp11.2 and 6p21. RCC is also associated with multiple genetic disorders including von Hippel-Lindau (VHL), tuberous sclerosis, Birt-Hogg-Dube, hereditary leiomyomatosis renal cell cancer, hereditary papillary renal carcinoma, and succinate dehydrogenase RCC and therefore it is important to know any family history of disease in order to make a diagnosis and as the patient ages watch for the manifestations of these syndromes (47). Five-year OS is >90% for patients with stage I, >80% for stage II, 70% for stage III, and <15% for stage IV disease (Table 1) (50).
Ultrasound is often the first modality utilized to detect the lesion due to low cost and no exposure to radiation. CT/MRI is then used to assess tumor size, local extension, enhancement pattern, presence of calcification, LNI, vascular invasion, contralateral lesions, and metastatic lesions. Geller et al. found radiologic studies have poor sensitivity (missing >42% of positive lymph nodes) when assessing for positive LNI (51).
RCC is notorious for its resistance to chemotherapy and radiation, therefore the mainstay of treatment is surgical resection (52). The preferred treatment option is surgical removal with partial or radical nephrectomy and lymph node dissection (47,50). Lymph node dissection is the standard of care for WT but given a paucity of a data, its utility as a prophylactic measure in RCC has not proven sufficiently beneficial (48).
A retrospective review done by Geller and Dome at St. Jude Children’s Research Hospital (53) found LNI in the pediatric population in the absence of metastatic disease has a relatively favorable long-term prognosis, with 72.4% disease-free survival at the last follow (follow up was 2–9 years). They also found that in those with node positive disease who underwent adjuvant therapy (radiotherapy, immunotherapy, and/or chemotherapy; specific treatment regimens were not discussed), there was no improvement in disease-free or OS (52). Although Geller et al. showed favorable long-term prognosis with no significant effect of adjuvant therapy, some argue lymph node dissection during time of nephrectomy contributes to the higher survival rates.
Lymph node positivity is high in the pediatric population; the AREN03B2 study found positive lymph nodes in 47.5% of patients with primary tumors less than 7 cm (53). Given the higher prevalence of positive lymph nodes in pediatric patients and poor accuracy, Geller et al. advocate for a more comprehensive approach to LN sampling and dissection (53). Additional studies are required to further elucidate the role of lymph node dissection in RCC. Lastly, it should be noted that nephron sparing surgery has been employed in the pediatric population in select populations including children with genetic disorders predisposing them to recurrent renal tumors (47).
Conclusions
Accurate lymph node imaging in pediatric renal and suprarenal cancers is critical because disease staging, treatment regimens, and clinical prognosis are highly dependent on lymph node invasion status and location. Surgical experience in WT and pRCC cases demonstrates additional importance of preoperatively localizing suspicious lymph nodes as extensive dissection has prognostic and therapeutic value, but also potentially increases morbidity. Despite this need, current standard-of-care imaging protocols, such as CT or MRI, have limited predictive ability. Strict imaging criteria for LNI in pediatric renal tumors has not been established. However, studies in both WT and pRCC have suggested size criteria to increase sensitivity and specificity, and while their results are encouraging, sample sizes are limited and large-scale studies are lacking (7,53). Research in NB staging has utilized advanced imaging modalities such as scintigraphy and PET to identify regional and distant spread of disease that is often undetected on conventional imaging. While the metabolic activity in NB is conducive to scintigraphy and PET, these modalities have been less intensely and successfully studied in WT and pRCC. Future research may show the benefit of incorporating advanced imaging modalities into dissection schema in future research.
Ultimately, pediatric renal and suprarenal tumors are relatively rare pathologies, but have high metastatic potential, and dramatically influence a young child’s life. As imaging modalities continue to evolve, expanded use for lymph node imaging in these pediatric cancers should be investigated.
Acknowledgements
This research was also made possible through the National Institutes of Health (NIH) Medical Research Scholars Program, a public-private partnership supported jointly by the NIH and generous contributions to the Foundation for the NIH from the Doris Duke Charitable Foundation, Genentech, the American Association for Dental Research, the Colgate-Palmolive Company, Elsevier, alumni of student research programs, and other individual supporters via contributions to the Foundation for the National Institutes of Health.
Footnote
Conflicts of Interest: The authors have no conflicts of interest to declare.
References
- Potisek NM, Antoon JW. Abdominal Masses. Pediatr Rev 2017;38:101-3. [Crossref] [PubMed]
- Wein AJ, Kavoussi LR, Partin AW, et al. Campbell-Walsh urology. Eleventh edition. Philadelphia, PA: Elsevier, 2016.
- Noone AM, Cronin KA, Altekruse SF, et al. Cancer Incidence and Survival Trends by Subtype Using Data from the Surveillance Epidemiology and End Results Program, 1992-2013. Cancer Epidemiol Biomarkers Prev 2017;26:632-41. [Crossref] [PubMed]
- Goo HW. Whole-body MRI of neuroblastoma. Eur J Radiol 2010;75:306-14. [Crossref] [PubMed]
- McDonald K, Duffy P, Chowdhury T, et al. Added value of abdominal cross-sectional imaging (CT or MRI) in staging of Wilms' tumours. Clin Radiol 2013;68:16-20. [Crossref] [PubMed]
- Miglioretti DL, Johnson E, Williams A, et al. The use of computed tomography in pediatrics and the associated radiation exposure and estimated cancer risk. JAMA Pediatr 2013;167:700-7. [Crossref] [PubMed]
- Lubahn JD, Cost NG, Kwon J, et al. Correlation between preoperative staging computerized tomography and pathological findings after nodal sampling in children with Wilms tumor. J Urol 2012;188:1500-4. [Crossref] [PubMed]
- Breslow N, Olshan A, Beckwith JB, et al. Epidemiology of Wilms tumor. Med Pediatr Oncol 1993;21:172-81. [Crossref] [PubMed]
- Raval MV, Bilimoria KY, Bentrem DJ, et al. Nodal evaluation in Wilms' tumors: analysis of the national cancer data base. Ann Surg 2010;251:559-65. [Crossref] [PubMed]
- Maas MH, Cransberg K, van Grotel M, et al. Renin-induced hypertension in Wilms tumor patients. Pediatr Blood Cancer 2007;48:500-3. [Crossref] [PubMed]
- Steinbrecher HA, Malone PS. Wilms' tumour and hypertension: incidence and outcome. Br J Urol 1995;76:241-3. [Crossref] [PubMed]
- Termuhlen AM, Tersak JM, Liu Q, et al. Twenty-five year follow-up of childhood Wilms tumor: a report from the Childhood Cancer Survivor Study. Pediatr Blood Cancer 2011;57:1210-6. [Crossref] [PubMed]
- Metzger ML, Dome JS. Current therapy for Wilms' tumor. Oncologist 2005;10:815-26. [Crossref] [PubMed]
- Dome JS, Perlman EJ, Graf N. Risk stratification for wilms tumor: current approach and future directions. Am Soc Clin Oncol Educ Book 2014.215-23; discussion 212-3. [Crossref] [PubMed]
- Green DM, Breslow NE, Beckwith JB, et al. Effect of duration of treatment on treatment outcome and cost of treatment for Wilms' tumor: a report from the National Wilms' Tumor Study Group. J Clin Oncol 1998;16:3744-51. [Crossref] [PubMed]
- Breslow NE, Ou SS, Beckwith JB, et al. Doxorubicin for favorable histology, Stage II-III Wilms tumor: results from the National Wilms Tumor Studies. Cancer 2004;101:1072-80. [Crossref] [PubMed]
- Ehrlich PF, Ritchey ML, Hamilton TE, et al. Quality assessment for Wilms' tumor: a report from the National Wilms' Tumor Study-5. J Pediatr Surg 2005;40:208-12. [Crossref] [PubMed]
- Kieran K, Anderson JR, Dome JS, et al. Lymph node involvement in Wilms tumor: results from National Wilms Tumor Studies 4 and 5. J Pediatr Surg 2012;47:700-6. [Crossref] [PubMed]
- Shamberger RC, Guthrie KA, Ritchey ML, et al. Surgery-related factors and local recurrence of Wilms tumor in National Wilms Tumor Study 4. Ann Surg 1999;229:292-7. [Crossref] [PubMed]
- Nanda RH, Shehata BM, Khoshnam N, et al. Impact of lymph node evaluation in adjuvant and neoadjuvant chemotherapy settings on survival outcomes in Wilms tumour: a review of 185 cases from a single institution. Pathology 2017;49:19-23. [Crossref] [PubMed]
- Ehrlich PF, Anderson JR, Ritchey ML, et al. Clinicopathologic findings predictive of relapse in children with stage III favorable-histology Wilms tumor. J Clin Oncol 2013;31:1196-201. [Crossref] [PubMed]
- Othersen HB Jr, DeLorimer A, Hrabovsky E, et al. Surgical evaluation of lymph node metastases in Wilms' tumor. J Pediatr Surg 1990;25:330-1. [Crossref] [PubMed]
- Brisse HJ, Smets AM, Kaste SC, et al. Imaging in unilateral Wilms tumour. Pediatr Radiol 2008;38:18-29. [Crossref] [PubMed]
- Gow KW, Roberts IF, Jamieson DH, et al. Local staging of Wilms' tumor--computerized tomography correlation with histological findings. J Pediatr Surg 2000;35:677-9. [Crossref] [PubMed]
- Hales PW, Olsen OE, Sebire NJ, et al. A multi-Gaussian model for apparent diffusion coefficient histogram analysis of Wilms' tumour subtype and response to chemotherapy. NMR Biomed 2015;28:948-57. [Crossref] [PubMed]
- Meeus EM, Zarinabad N, Manias KA, et al. Diffusion-weighted MRI and intravoxel incoherent motion model for diagnosis of pediatric solid abdominal tumors. J Magn Reson Imaging 2018;47:1475-86. [Crossref] [PubMed]
- Begent J, Sebire NJ, Levitt G, et al. Pilot study of F(18)-Fluorodeoxyglucose Positron Emission Tomography/computerised tomography in Wilms' tumour: correlation with conventional imaging, pathology and immunohistochemistry. Eur J Cancer 2011;47:389-96. [Crossref] [PubMed]
- Provenzi M, Saettini F, Conter V, et al. Is there a role for FDG-PET for the assessment of treatment efficacy in Wilms' tumor? A case report and literature review. Pediatr Hematol Oncol 2013;30:633-9. [Crossref] [PubMed]
- Kliegman R, Stanton B, St. Geme JW, et al. Nelson textbook of pediatrics. Edition 20. Philadelphia, PA: Elsevier, 2016.
- Kushner BH. Neuroblastoma: a disease requiring a multitude of imaging studies. J Nucl Med 2004;45:1172-88. [PubMed]
- Heczey A, Louis CU. Advances in chimeric antigen receptor immunotherapy for neuroblastoma. Discov Med 2013;16:287-94. [PubMed]
- Huang M, Weiss WA. Neuroblastoma and MYCN. Cold Spring Harb Perspect Med 2013;3. [Crossref] [PubMed]
- DuBois SG, Kalika Y, Lukens JN, et al. Metastatic sites in stage IV and IVS neuroblastoma correlate with age, tumor biology, and survival. J Pediatr Hematol Oncol 1999;21:181-9. [Crossref] [PubMed]
- Morgenstern DA, London WB, Stephens D, et al. Metastatic neuroblastoma confined to distant lymph nodes (stage 4N) predicts outcome in patients with stage 4 disease: A study from the International Neuroblastoma Risk Group Database. J Clin Oncol 2014;32:1228-35. [Crossref] [PubMed]
- Mullassery D, Farrelly P, Losty PD. Does aggressive surgical resection improve survival in advanced stage 3 and 4 neuroblastoma? A systematic review and meta-analysis. Pediatr Hematol Oncol 2014;31:703-16. [Crossref] [PubMed]
- Siegel MJ, Ishwaran H, Fletcher BD, et al. Staging of neuroblastoma at imaging: report of the radiology diagnostic oncology group. Radiology 2002;223:168-75. [Crossref] [PubMed]
- Pfluger T, Schmied C, Porn U, et al. Integrated imaging using MRI and 123I metaiodobenzylguanidine scintigraphy to improve sensitivity and specificity in the diagnosis of pediatric neuroblastoma. AJR Am J Roentgenol 2003;181:1115-24. [Crossref] [PubMed]
- Kocaoglu M, Bulakbasi N, Sanal HT, et al. Pediatric abdominal masses: diagnostic accuracy of diffusion weighted MRI. Magn Reson Imaging 2010;28:629-36. [Crossref] [PubMed]
- Demir S, Altinkaya N, Kocer NE, et al. Variations in apparent diffusion coefficient values following chemotherapy in pediatric neuroblastoma. Diagn Interv Radiol 2015;21:184-8. [Crossref] [PubMed]
- Candito M, Thyss A, Albertini M, et al. Methylated catecholamine metabolites for diagnosis of neuroblastoma. Med Pediatr Oncol 1992;20:215-20. [Crossref] [PubMed]
- Matthay KK, Yanik G, Messina J, et al. Phase II study on the effect of disease sites, age, and prior therapy on response to iodine-131-metaiodobenzylguanidine therapy in refractory neuroblastoma. J Clin Oncol 2007;25:1054-60. [Crossref] [PubMed]
- Matthay KK, George RE, Yu AL. Promising therapeutic targets in neuroblastoma. Clin Cancer Res 2012;18:2740-53. [Crossref] [PubMed]
- Shapiro B, Gross MD. Radiochemistry, biochemistry, and kinetics of 131I-metaiodobenzylguanidine (MIBG) and 123I-MIBG: clinical implications of the use of 123I-MIBG. Med Pediatr Oncol 1987;15:170-7. [Crossref] [PubMed]
- Dhull VS, Sharma P, Patel C, et al. Diagnostic value of 18F-FDG PET/CT in paediatric neuroblastoma: comparison with 131I-MIBG scintigraphy. Nucl Med Commun 2015;36:1007-13. [Crossref] [PubMed]
- Colavolpe C, Guedj E, Cammilleri S, et al. Utility of FDG-PET/CT in the follow-up of neuroblastoma which became MIBG-negative. Pediatr Blood Cancer 2008;51:828-31. [Crossref] [PubMed]
- Liu CJ, Lu MY, Liu YL, et al. Risk Stratification of Pediatric Patients With Neuroblastoma Using Volumetric Parameters of 18F-FDG and 18F-DOPA PET/CT. Clin Nucl Med 2017;42:e142-8. [Crossref] [PubMed]
- Young EE, Brown CT, Merguerian PA, et al. Pediatric and adolescent renal cell carcinoma. Urol Oncol 2016;34:42-9. [Crossref] [PubMed]
- Syed JS, Nguyen KA, Wu CQ, et al. Distinguishing pediatric and adolescent renal cell carcinoma from other renal malignancies. Pediatr Blood Cancer 2017.64. [PubMed]
- Akhavan A, Richards M, Shnorhavorian M, et al. Renal cell carcinoma in children, adolescents and young adults: a National Cancer Database study. J Urol 2015;193:1336-41. [Crossref] [PubMed]
- Wilms Tumor and Other Childhood Kidney Tumors Treatment (PDQ(R)): Health Professional Version. PDQ Cancer Information Summaries. Bethesda (MD) 2002.
- Geller JI, Ehrlich PF, Cost NG, et al. Characterization of adolescent and pediatric renal cell carcinoma: A report from the Children's Oncology Group study AREN03B2. Cancer 2015;121:2457-64. [Crossref] [PubMed]
- Sausville JE, Hernandez DJ, Argani P, et al. Pediatric renal cell carcinoma. J Pediatr Urol 2009;5:308-14. [Crossref] [PubMed]
- Geller JI, Dome JS. Local lymph node involvement does not predict poor outcome in pediatric renal cell carcinoma. Cancer 2004;101:1575-83. [Crossref] [PubMed]