Coping with chemoresistance in prostate cancer—co-targeting of adipose stromal cells?
“Obesity begets cancer” is an apt adage considering increasing research pointing to obesity as an important causal factor for increased risk and aggressiveness of many cancer types (1-3). In prostate cancer (PCa), obesity plays a complex role with obesity reported to reduce the risk of localized, low grade, indolent PCa (4) while increasing the risk of aggressive, high grade disease (5). Obesity has been associated with increased PCa mortality as a result of aggressive disease (1,3). In addition, obesity leads to increased risk of biochemical recurrence (BCR) after radical prostatectomy (RP) (4). Considering the increasing prevalence of obesity across populations and increasing incidences and mortality of aggressive PCa, understanding the underlying mechanisms are vital and is currently an area of active investigations.
Prostate gland is surrounded by periprostatic white adipose tissue (PPAT). PPAT is composed of predominantly adipocytes and other cell types such as macrophages, lymphocytes, fibroblasts, vascular endothelial cells and adipose stromal cells (ASCs). An admixture of PPAT with prostate tumor cells lead to extraprostatic extension (6), a frequent finding in aggressive PCa, that is associated with poor prognosis. Obesity increases extraprostatic extension as the abundance of PPAT is increased in obesity, promoting the admixture of these fat cells with tumor cells, leading to increased PCa aggressiveness (7). Burgeoning evidence indicates that there is a cross talk between fat cells and cancer cells that leads to aggressive PCa. Paracrine mitogenic, anti-apoptotic, angiogenic and immune suppressive signaling from adipose cells is involved in this cross-talk leading to enhanced tumorigenesis (2,7). Mature adipocytes, the lipid storing cells of fat tissue, are highly ‘endocrine’ cells that secrete a plethora of hormones, growth factors, chemokines or pro-inflammatory adipokines (8,9). Laurent et al. demonstrated that adipocytes secrete a chemokine, CCL7 that diffuses from PPAT to the peripheral zone of the human prostate gland. CCL7 binds to chemokine receptor CCR3 expressed on the surface of PCa cells, thereby stimulating the migration of these cancer cells leading to extraprostatic extension (8). Similarly, fatty acid-binding protein 4 (FABP4) was shown to be released by adipocytes that is taken up by PCa cells to promote invasion (3,10).
Adipocytes are derived by differentiation from mesenchymal, fibroblastic progenitor cells termed ASCs. Like mature adipocytes, ASCs also secrete tumor-trophic adipokines, that cause ASCs to migrate from adipose tissue to tumor (Figure 1). Tumor migrant ASCs are thought to contribute to tumor stroma like adipocytes and obesity promotes ASC trafficking from adipose tissue to tumors, leading to cancer progression and poor survival. In a recent study, Su et al. (in 2018) demonstrate that ASCs induce epithelial-to-mesenchymal transition (EMT) in prostate carcinoma cells, leading to increased aggressiveness of PCa cells, that underlies chemoresistance (11) (Figure 1). Epithelial-mesenchymal transition (EMT) is considered as the initiating and most critical step in tumor invasion and metastasis that is characterized by changes in gene expression, including decreases in epithelial genes (such as E-cadherin) and increases in mesenchymal genes (such as N-cadherin and vimentin) (12-14). Following EMT, cells become motile, detach from the primary tumor and colonize distant sites, seeding the development of secondary tumors (13,15). Su et al. (11) employed androgen-dependent PC3 or androgen-independent LNCaP cells transduced with GFP and co-cultured these cells with non-fluorescent human primary ASCs, derived from periprostatic adipose tissue. GFP labelling of PCa cell lines enabled tracking of these cells in mixed culture with non-GFP ASCs. After 24 hours of co-culture, cells were fixed and immunofluorescence staining was performed for epithelial marker E-cadherin and mesenchymal marker N-cadherin. While control cells showed membranous E-cadherin staining consistent with their epithelial phenotype, PCa cells co-cultured with ASCs lost this epithelial marker and gained mesenchymal N-cadherin staining, suggesting an induction of EMT in these PCa cell lines. To validate this EMT phenotype, they FACS-sorted PCa cells from co-cultured ASCs by GFP fluorescence followed by RT-PCR analyses. Epithelial marker Cdh1 (encodes E-cadherin) expression was reduced concomitant with induction of mesenchymal markers Cdh2 (encodes N-cadherin), fibronectin, vimentin, Zeb2, Slug1 confirming EMT induction upon co-culture with ASCs. To examine the consequences of these EMT alterations on cellular motility, the authors performed ‘wound healing assay’ and ‘trans-well Boyden chamber assay’. These assays showed that co-culture with ASCs induced the motility of PCa cell lines owing to EMT induction. Since EMT has been implicated in cancer chemoresistance (16), the authors also evaluated the effects of ASCs on sensitivity of PCa cells to chemotherapeutic drugs. PCa cells survived higher doses of chemotherapeutic drugs cabazitaxel, docetaxel and cisplatin when grown in the presence of ASCs than those grown without ASCs. Further, the cells surviving drug treatment were found to be the ones with mesenchymal characteristics, indicating that mesenchymal cells are less drug sensitive that their epithelial counterparts.
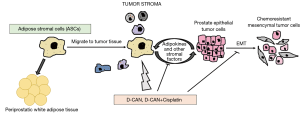
Further, authors examined the validity of these in vitro findings in a mouse tumor model (11). Experimental male mice were fed a high fat diet for 12 weeks that led to diet-induced obesity. Control mice were age-matched males who received low fat diet for this period. These two groups of mice underwent subcutaneous grafting of spheroids of HMVP2 cell line. HMVP2 cell line was previously established by this group from tumors of a PCa mouse Hi-Myc model that shows basal-epithelial characteristics (17). Obese mice developed tumors at a faster rate that matched lean mice. Further, the tumors were resected and histologically assessed. Tumors in obese mice showed tumor invasion into the surrounding adipose tissue with higher lymphocyte infiltration and a higher cellular proliferation than lean mice. Consistent with the observed in vitro effects of ASCs on inducing EMT in PCa cells, tumors from obese mice contained less epithelial E-cadherin positive cells and more mesenchymal N-cadherin staining cells as compared to controls.
This group has previously designed peptides to specifically target ASC populations, called D-Wat and D-CAN (18-20). These ‘hunter-killer peptides’ are composed of an ASC binding domain and a pro-apoptotic domain that show a dose-dependent ASC cytotoxicity and have the potential to suppress tumor growth in mice (19). Treatment of co-culture of ASCs and LNCaP cells with these peptides rendered PCa cells chemosensitive to the drugs cabazitaxel, docetaxel and cisplatin (11). Surviving LNCaP cells were found to be less migratory upon ASC depletion pointing to reduction in mesenchymal features. Importantly, D-CAN treatment of obese mice resulted in reversal of the obesity effects including reduction in overall tumor growth, cellular proliferation, tumor invasion into surrounding PPAT and lymphocyte infiltration. Observed effects on EMT were also reversed upon DCAN treatment.
Next, the authors sought to examine the effects of ASC depletion in vivo on chemosensitivity of PCa cells (11). Mice subcutaneously grafted with HMVP2 spheroids were treated either with cisplatin alone (at two doses 0.5 or 2.4 mg/kg) or with D-CAN or a combination of 0.5 mg/kg cisplatin and D-CAN. Cisplatin was not effective alone at low dose of 0.5 mg/kg. However, when combined with D-CAN, this low dose of cisplatin was sufficient to suppress tumor growth without any signs of toxicity. These data suggest that the effects of chemotherapeutic drugs can be enhanced by co-targeting ASCs. Collectively, these findings imply that cross-talk of tumor cells with ASCs drive EMT, leading to protection of carcinoma cells from chemotherapy (Figure 1). Similar to ASCs, the authors found that mature adipocytes also induced EMT and chemoresistance (11). It remains to be determined if ASCs and adipocytes converge on similar pathways in impacting EMT or if these cell types act via distinct mechanisms. Nonetheless, in view of the findings of Su et al. (11), dosing of chemotherapeutic drugs for cancer treatment need to factor in obesity. This study in conjunction with other similar studies in this direction (7,21,22) emphasize a need for specifically tailored therapies for obese patients to improve survival outcomes.
Acknowledgments
Funding: Authors’ research is supported by the National Institutes of Health (NIH) (Award No. R01CA177984) and Department of Defense (DoD) Prostate Cancer Research Program (Award No. W81XWH1810303). Opinions, interpretations, conclusions and recommendations are those of the author and are not necessarily endorsed by the NIH/DoD.
Footnote
Conflicts of Interest: The authors have no conflicts of interest to declare.
References
- Buschemeyer WC 3rd, Freedland SJ. Obesity and prostate cancer: epidemiology and clinical implications. Eur Urol 2007;52:331-43. [Crossref] [PubMed]
- Lengyel E, Makowski L, DiGiovanni J, et al. Cancer as a Matter of Fat: The Crosstalk between Adipose Tissue and Tumors. Trends Cancer 2018;4:374-84. [Crossref] [PubMed]
- Uehara H, Kobayashi T, Matsumoto M, et al. Adipose tissue: Critical contributor to the development of prostate cancer. J Med Invest 2018;65:9-17. [Crossref] [PubMed]
- Asmar R, Beebe-Dimmer JL, Korgavkar K, et al. Hypertension, obesity and prostate cancer biochemical recurrence after radical prostatectomy. Prostate Cancer Prostatic Dis 2013;16:62-6. [Crossref] [PubMed]
- Vidal AC, Howard LE, Moreira DM, et al. Obesity increases the risk for high-grade prostate cancer: results from the REDUCE study. Cancer Epidemiol Biomarkers Prev 2014;23:2936-42. [Crossref] [PubMed]
- Sung MT, Eble JN, Cheng L. Invasion of fat justifies assignment of stage pT3a in prostatic adenocarcinoma. Pathology 2006;38:309-11. [Crossref] [PubMed]
- van Roermund JG, Hinnen KA, Tolman CJ, et al. Periprostatic fat correlates with tumour aggressiveness in prostate cancer patients. BJU Int 2011;107:1775-9. [Crossref] [PubMed]
- Laurent V, Guerard A, Mazerolles C, et al. Periprostatic adipocytes act as a driving force for prostate cancer progression in obesity. Nat Commun 2016;7:10230. [Crossref] [PubMed]
- Ouchi N, Parker JL, Lugus JJ, et al. Adipokines in inflammation and metabolic disease. Nat Rev Immunol 2011;11:85-97. [Crossref] [PubMed]
- Uehara H, Takahashi T, Oha M, et al. Exogenous fatty acid binding protein 4 promotes human prostate cancer cell progression. Int J Cancer 2014;135:2558-68. [Crossref] [PubMed]
- Su F, Ahn S, Saha A, et al. Adipose stromal cell targeting suppresses prostate cancer epithelial-mesenchymal transition and chemoresistance. Oncogene 2019;38:1979-88. [Crossref] [PubMed]
- Kalluri R. EMT: when epithelial cells decide to become mesenchymal-like cells. J Clin Invest 2009;119:1417-9. [Crossref] [PubMed]
- Kalluri R, Weinberg RA. The basics of epithelial-mesenchymal transition. J Clin Invest 2009;119:1420-8. [Crossref] [PubMed]
- Sekhon K, Bucay N, Majid S, et al. MicroRNAs and epithelial-mesenchymal transition in prostate cancer. Oncotarget 2016;7:67597-611. [Crossref] [PubMed]
- Yang J, Weinberg RA. Epithelial-mesenchymal transition: at the crossroads of development and tumor metastasis. Dev Cell 2008;14:818-29. [Crossref] [PubMed]
- Wang J, Wei Q, Wang X, et al. Transition to resistance: An unexpected role of the EMT in cancer chemoresistance. Genes Dis 2016;3:3-6. [Crossref] [PubMed]
- Saha A, Blando J, Fernandez I, et al. Linneg Sca-1high CD49fhigh prostate cancer cells derived from the Hi-Myc mouse model are tumor-initiating cells with basal-epithelial characteristics and differentiation potential in vitro and in vivo. Oncotarget 2016;7:25194-207. [Crossref] [PubMed]
- Daquinag AC, Dadbin A, Snyder B, et al. Non-glycanated Decorin Is a Drug Target on Human Adipose Stromal Cells. Mol Ther Oncolytics 2017;6:1-9. [Crossref] [PubMed]
- Daquinag AC, Tseng C, Salameh A, et al. Depletion of white adipocyte progenitors induces beige adipocyte differentiation and suppresses obesity development. Cell Death Differ 2015;22:351-63. [Crossref] [PubMed]
- Daquinag AC, Tseng C, Zhang Y, et al. Targeted Proapoptotic Peptides Depleting Adipose Stromal Cells Inhibit Tumor Growth. Mol Ther 2016;24:34-40. [Crossref] [PubMed]
- Duong MN, Cleret A, Matera EL, et al. Adipose cells promote resistance of breast cancer cells to trastuzumab-mediated antibody-dependent cellular cytotoxicity. Breast Cancer Res 2015;17:57. [Crossref] [PubMed]
- Nowicka A, Marini FC, Solley TN, et al. Human omental-derived adipose stem cells increase ovarian cancer proliferation, migration, and chemoresistance. PLoS One 2013;8:e81859. [Crossref] [PubMed]