Adipose tissue: enabler of prostate cancer aggressive behavior
Prostate tumor progression to lethal disease
Prostate cancer is an important contributor to cancer morbidity and mortality worldwide. In 2018, there was an estimated 164,690 new cases of prostate cancer and 29,430 deaths due to prostate cancer among American patients, making it the most common cause of cancer diagnosis in males (1). Prostate cancer is the second most common cause of cancer death among males, following respiratory tract malignancies (1). The historic discovery of androgen deprivation therapy (ADT) efficacy against prostate cancer by Huggins et al. in 1941 set the first line therapy for treatment that persists to this day (2,3). Since then, advances in therapy have improved the 5-year survival rate of non-metastatic prostate cancer to 98.9% but patients with metastatic disease upon diagnosis experienced only a modest 28.2% 5-year survival (4).
The process of epithelial-to-mesenchymal transition (EMT) has been phenotypically held responsible as the driving force for malignant prostate epithelium to circumvent ADT, resulting in castration resistant prostate cancer (CRPC), as well as resistance to chemotherapeutics (docetaxel, cabazitaxel, and cisplatin) used for the treatment of metastatic CRPC. In addition to therapeutic resistance, the EMT phenotype results in the loss of the polarized structure of normal prostate epithelium in favor of a de-differentiated, highly migratory, and invasive phenotype (Figure 1) (5). The contribution of adipose (fat) tissue that characterizes obesity to prostate tumorigenesis has been previously considered as a risk factor of prostate cancer progression, but the association remained unclear at the functional level or in therapeutic resistance. In a recent manuscript by Su and colleagues published in Oncogene, the authors provide intriguing new insights into the influence of excess adipose tissue on EMT-driven prostate cancer progression and therapeutic resistance (6). These findings are particularly intriguing in the realm of prostate cancer risk factors as there is evidence to suggest that obese individuals are more likely to suffer from advanced, more fatal forms of the disease (7). Using robust in vitro and in vivo experimental models the authors establish with confidence a functional link between obesity and the EMT induction under the control of fatty tissues. They also introduce potential for anti-adipose stromal cell (ASC) peptide therapies to potentiate the anti-cancer effects of cisplatin against prostate cancer.
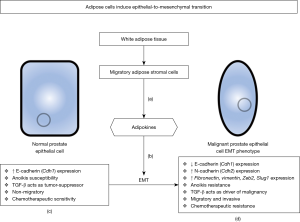
Obesity dictates phenotypic EMT outcomes
The hallmark characteristic of an EMT phenotype in any epithelial-derived cancer is the loss of the surface marker E-cadherin (Cdh1) in favor of the normally mesenchymal N-cadherin (Cdh2), a change that is a major contributor to the tumor ability to migrate from the glandular epithelial network and metastasize to distant sites (8). A multilayered interplay of adipose stromal cells and tumor epithelial cells in the tumor microenvironment confer EMT phenotypic changes in the epithelium (Figure 1). Inflammatory cells contribute to changes in the tumor microenvironment by engaging the conversion of fibroblasts into myofibroblasts, recruitment of cancer-associated fibroblasts and myeloid derived suppressor cells, and introducing migrating adipose progenitors (9-11). The inflammatory effects of white adipose tissue (WAT), the tissue type that is overgrown in obesity, enhance the malignant characteristic of prostate cancer by driving this EMT phenotype (6,10). There is compelling previous evidence that suggests that the effects of obesity on prostate cancer are mediated by ASC migration to the tumor microenvironment (11). ASCs are the fibroblastic mesenchymal cells that differentiate into adipocytes in normal WAT fat storage. In the setting of obesity, ASCs mobilize from abdominal and peritumoral adipose tissue to the tumor stroma where the cells release tumor-trophic signaling factors termed adipokines (11,12). A well characterized inducer of EMT is transforming growth factor-β (TGF-β), a signaling molecule with opposing roles in the malignant prostate epithelium (13). In normal or pre-malignant prostate epithelium TGF-β induces apoptosis via canonical SMAD signaling when separated from the glandular extracellular matrix (ECM), a process termed anoikis (14). In malignant prostate tumors TGF-β drives cell survival and migration via non-canonical SMAD signaling and cell-matrix remodeling, respectively, both phenotypically characterized by EMT (13).
In this study, the authors cleverly used an in vitro model to demonstrate the ability of ASCs to induce EMT in human prostate cancer cells by co-culturing with ASCs derived from peri-prostatic WAT, resulting in the induction of EMT changes as outlined in Figure 1 (6). Co-cultured cell lines were then treated with one the clinically used chemotherapeutics for CRPC, docetaxel, cabazitaxel, or cisplatin. The observed result was markedly increased cell survival in co-cultured EMT-induced cell lines when compared to cells grown in the absence of ASCs. As expected, the chemotherapeutics induced selective death of cells with preserved epithelial phenotype, as opposed to the cells demonstrating the EMT phenotype (6). To revert the effects of ASC-induced EMT, the authors used a hunter-killer peptide directed against ASCs entitled D-CAN resulting in renewed chemotherapeutic sensitivity amongst the ASC co-cultured prostate cancer cell lines, with decreased migratory activity (6). Interestingly, prostate cancer cells co-cultured with only soluble factors from ASCs exhibited enhanced migration properties, implicating the importance of the microenvironment, not isolated signaling effectors (6).
Previous experimental efforts targeting the EMT phenotype to overcome therapeutic resistance in prostate tumors have centered around fibroblasts and T-cells and were met with limited success (5). The clinical impact of the present pre-clinical work stems from the identification of adipose tissue and the migratory fibroblasts that it releases as a potential specific target against advanced prostate cancer. Building on the in vitro studies, the in vivo evidence from this work indicates that anti-ASC therapy disrupts prostate cancer progression and increases chemotherapeutic sensitivity. Using a tumor-graft mice model, researchers provided supporting information that obese mice experienced faster growth of Hi-Myc prostate cancer with a higher degree of prostate cancer invasion into surrounding WAT, lymphocytic infiltration, and cell proliferation that correlated with EMT phenotypic changes (6). Treatment of tumor-grafted mice with D-CAN resulted in suppression of tumor growth and concomitant reversion of the EMT phenotype to MET (mesenchymal-epithelial-transition) in both obese and non-obese mice (6). These results indicate that the influence of ASCs on progression to an aggressive EMT phenotype in prostate cancer is not only limited to obese individuals. However, the relatively low specificity in the recruitment of adipose tissues as the absolute enabler of therapeutic resistance in advanced prostate tumors certainly appears as a limitation of the study. Of major translational relevance is the observation that tumor-grafted mice treated with a combination cisplatin and D-CAN therapy benefited from prolonged survival and decreased tumor burden (potentially driven by increased tumor apoptosis), compared to either treatment alone (6). Scrutinizing these results from a wider angle, one must recognize the increasing promise of combination strategies tailored to target various components of the tumor microenvironment. Recent work from our group and others has demonstrated that effective blockade of TGF-β signaling, a mediator of EMT phenotypic landscape in prostate cancer, in combination with the antiandrogen enzalutamide impairs aggressive prostate tumor growth (via apoptosis induction) and inhibits tumor cell invasion (via reversing EMT to MET) in pre-clinical prostate tumor models by reducing motility effectors found in EMT (15,16).
In summary, this work by Su et al. provides a new provocative platform for personalized therapies based on the adipose layers characterizing individual prostate tumors. Making a most compelling case, the investigators provide solid evidence to support the epidemiologic data presented by the American Cancer Society on the relationship between obesity and high mortality advanced prostate cancer. While one may easily argue that obese patients at risk for high-grade prostate cancer may clinically benefit from novel anti-ASC therapies targeting dipose tissues to halt the EMT-dictated therapeutic resistance, recent work in lung cancer brings sharp contrast to the function of adipose tissue. Elegant studies published in Nature Medicine (in 2019) demonstrated a paradoxical increase by obesity in the efficacy of anti-tumor immune checkpoint blockade in lung cancer, implicating a dynamic interplay between adiposity and inflammatory mediators contributing to cancer therapeutic response (17). This complexity needs to be dissected carefully as we advance towards personalized treatment strategies for specific tumors benefited by immunotherapy. In the meantime, we acknowledge that in an unexpected twist of phenotypic changes, fat tissue takes diverse role to shape new plans of therapeutic attack for cancer patients!
Acknowledgments
The authors would like to acknowledge the James F. Hardymon Endowment (NK) and the Center for Clinical and Translational Research PSMR Fellowship (CAW).
Footnote
Conflicts of Interest: The authors have no conflicts of interest to declare.
References
- Siegel RL, Miller KD, Jemal A. Cancer statistics, 2018. CA Cancer J Clin 2018;68:7-30. [Crossref] [PubMed]
- Huggins C, Hodges CV. Studies on prostatic cancer. I. The effect of castration, of estrogen and androgen injection on serum phosphatases in metastatic carcinoma of the prostate. CA Cancer J Clin 1972;22:232-40. [Crossref] [PubMed]
- Sanda MG, Cadeddu JA, Kirkby E, et al. Clinically Localized Prostate Cancer: AUA/ASTRO/SUO Guideline. Part I: Risk Stratification, Shared Decision Making, and Care Options. J Urol 2018;199:683-90. [Crossref] [PubMed]
- Crawford ED, Petrylak D, Sartor O. Navigating the evolving therapeutic landscape in advanced prostate cancer. Urol Oncol 2017;35S:S1-13. [Crossref] [PubMed]
- Wade CA, Kyprianou N. Profiling Prostate Cancer Therapeutic Resistance. Int J Mol Sci 2018.19. [PubMed]
- Su F, Ahn S, Saha A, et al. Adipose stromal cell targeting suppresses prostate cancer epithelial-mesenchymal transition and chemoresistance. Oncogene 2019;38:1979-88. [Crossref] [PubMed]
- Society AC. Prostate Cancer Risk Factors. American Cancer Society, 2016. Available online: https://www.cancer.org/cancer/prostate-cancer/causes-risks-prevention/risk-factors.html
- Kumar S, Park SH, Cieply B, et al. A pathway for the control of anoikis sensitivity by E-cadherin and epithelial-to-mesenchymal transition. Mol Cell Biol 2011;31:4036-51. [Crossref] [PubMed]
- Calcinotto A, Spataro C, Zagato E, et al. IL-23 secreted by myeloid cells drives castration-resistant prostate cancer. Nature 2018;559:363-9. [Crossref] [PubMed]
- Jung Y, Kim JK, Shiozawa Y, et al. Recruitment of mesenchymal stem cells into prostate tumours promotes metastasis. Nat Commun 2013;4:1795. [Crossref] [PubMed]
- Zhang Y, Daquinag AC, Amaya-Manzanares F, et al. Stromal progenitor cells from endogenous adipose tissue contribute to pericytes and adipocytes that populate the tumor microenvironment. Cancer Res 2012;72:5198-208. [Crossref] [PubMed]
- Park J, Morley TS, Kim M, et al. Obesity and cancer--mechanisms underlying tumour progression and recurrence. Nat Rev Endocrinol 2014;10:455-65. [Crossref] [PubMed]
- Tu H, Jacobs SC, Borkowski A, et al. Incidence of apoptosis and cell proliferation in prostate cancer: relationship with TGF-beta1 and bcl-2 expression. Int J Cancer 1996;69:357-63. [Crossref] [PubMed]
- Frisch SM, Francis H. Disruption of epithelial cell-matrix interactions induces apoptosis. J Cell Biol 1994;124:619-26. [Crossref] [PubMed]
- Paller C, Pu H, Begemann DE, et al. TGF-beta receptor I inhibitor enhances response to enzalutamide in a pre-clinical model of advanced prostate cancer. Prostate 2019;79:31-43. [Crossref] [PubMed]
- Song B, Park SH, Zhao JC, et al. Targeting FOXA1-mediated repression of TGF-beta signaling suppresses castration-resistant prostate cancer progression. J Clin Invest 2019;129:569-82. [Crossref] [PubMed]
- Wang Z, Aguilar EG, Luna JI, et al. Paradoxical effects of obesity on T cell function during tumor progression and PD-1 checkpoint blockade. Nat Med 2019;25:141-51. [Crossref] [PubMed]