Androgen receptor-mediated non-genomic regulation of prostate cancer cell proliferation
Introduction
Androgen receptor (AR) is a classic steroid hormone receptor that is critical for prostate cancer development and progression. In its unbound conformation, AR is located primarily in the cytoplasm in complex with heat shock proteins, cytoskeletal proteins, and other chaperones (1-5). These proteins also enable modulation of AR conformation for efficient ligand binding (6,7). When androgen binds AR, AR forms a homodimer, undergoes a conformational change, and interacts with additional proteins that facilitate its nuclear translocation (8-10). Once in the nucleus, AR binds to the androgen response elements (AREs) on promoter/enhancer regions, recruits coregulators, and forms the transcriptional machinery for AR-regulated gene expression (10). This AR-signaling pathway, known as the genomic pathway, relies on AR nuclear translocation and AR-DNA binding for cell proliferation. The genomic pathway is thought to occur over several hours and is characterized by increased expression of specific AR-regulated genes (Figure 1).
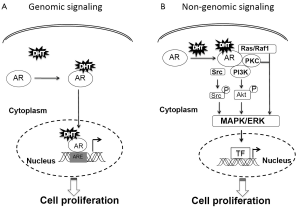
However, studies have shown a rapid and reversible AR signaling that occurs within minutes and results in regulation of prostate cancer cell proliferation (11-13). This AR-signaling pathway, known as the non-genomic pathway, requires neither AR nuclear translocation nor AR-DNA binding. Instead, cytoplasmic AR signaling may function through mitogen-activated protein kinase (MAPK) signaling cascades, converging on extracellular signal-regulated kinase (ERK) activation (14,15). Treatment of AR-positive prostate cancer cells with 5α-dihydrotestosterone (DHT) leads to increased ERK-1/2 phosphorylation within 5 minutes in a dosage-dependent manner (13) (Figure 1).
While non-genomic AR signaling has thus far been shown to primarily require MAPK/ERK activation, cell signaling can also occur without ERK activation. Non-ERK pathways involve activation of mammalian target of rapamycin (mTOR) via the phosphatidyl-inositol 3-kinase (PI3K)/Akt pathway or involvement of plasma membrane, G protein coupled receptors (GPCRs) and the sex hormone binding globulin receptor (SHBGR) that modulate intracellular Ca2+ concentration and cyclic adenosine monophosphate (cAMP) levels, respectively (16,17).
In addition, non-genomic AR signaling may be mediated by a membrane-bound AR that can regulate intracellular Ca2+ concentration and membrane ion channels (18,19). Studies with bovine serum albumin (BSA)-bound DHT, a compound that is unable to penetrate the plasma membrane, show a dose-dependent suppression of the PI3K and MAPK pathways (20). These data indicate that non-genomic AR signaling may suppress proliferation via membrane-bound AR (21) or activate proliferation via cytoplasmic AR.
Finally, recent data indicates that the non-genomic AR signaling may regulate genomic AR signaling and that the non-genomic and genomic AR signaling may work together to coordinate gene regulation in prostate cancer cells. In this manuscript, we provide a comprehensive review of non-genomic AR signaling with an emphasis on the established role of MAPK/ERK in prostate cancer cell proliferation. Clinically, understanding of these non-genomic AR signaling pathways is important, as they may represent potential mechanisms of resistance to AR antagonists. AR antagonists including casodex and flutamide have no effect on non-genomic AR signaling, as evidenced by ERK phosphorylation in the presence of these drugs (13). Thus, elucidation of non-genomic AR signaling pathways may enable development of novel agents to inhibit all forms of AR signaling in prostate cancer.
ERK-1/2 mediated non-genomic AR signaling
The MAPK/ERK signaling cascade is important in regulating diverse biological functions including cell survival, motility, and proliferation, which are essential to prostate carcinogenesis (22). Aberrant activation of kinases in this pathway is frequently reported in human cancer (23-25). Studies of DHT-responsiveness in prostate cancer cells in our lab and others show evidence of ERK-1/2 phosphorylation within 1-2 minutes of DHT treatment and peak levels of ERK-1/2 phosphorylation within 5-10 minutes. Activated ERK-1/2 then translocates to the nucleus and directly interacts with and phosphorylates transcription factors (TFs), such as nuclear ETS domain-containing Elk1 (26-28). Elk1 transcriptionally regulates immediate early genes (IEGs) such as c-fos (26,29), which coordinately regulates the expression of several genes involved in cell proliferation (26,27). This response is AR-dependent as no effect was observed in AR-negative PC-3 prostate cancer cells (13). Thus, while ERK phosphorylation occurs within minutes and serves as a measurable response of non-genomic activation, the molecular processes involved in cell proliferation occur over several hours and days.
DHT-induced ERK activation in prostate cancer cells may be mediated via multiple pathways, including the PI3K/Akt, Ras-Raf, and protein kinase C (PKC) pathways. Extensive evidence suggests AR associates with plasma membrane lipid rafts that facilitate AR activation of these pathways (30). AR activation of the PI3K/Akt pathway involves direct interaction of AR with the p85α regulatory subunit of PI3K (31), while the activation of the Ras-Raf pathway may involve the sequential activation of Ras, Raf and MEK kinases and may be dependent on the formation of an AR-Src complex (29,32). Importantly, Src or scaffolding proteins like proline-, glutamic acid-, and leucine-rich protein-1 (PELP1) may modulate the interaction of AR with Akt (31,33). PKC activation of ERK may involve modulation of intracellular Ca2+ concentration (13). Furthermore, ERK and Src are calcium-dependent kinase cascades suggesting AR could directly regulate them via mobilization of intracellular Ca2+ levels (32,34). Each of these AR signaling pathways can result in ERK activation and represent redundancy that ensures a proliferative response to DHT. Further, crosstalk between AR-mediated signaling cascades suggests a complex network of signals that converge on ERK phosphorylation. Each of these pathways is individually discussed in detail below.
PI3K/Akt/PTEN pathway
Ligand binding induces AR to directly interact with the p85α regulatory subunit of PI3K, resulting in the activation of the PI3K/Akt pathway (31). PI3K phosphorylates Akt (also known as PKB), a subfamily of serine-threonine protein kinases. Akt expression is frequently observed to be elevated in human prostate, ovarian, and breast cancers (35-37). The PI3K/Akt pathway activates the MAPK/ERK cascade and is regulated by phosphatase and tensin homolog (PTEN). PTEN, a protein phosphatase that dephosphorylates phosphatidyl-inositol (3,4,5)-triphosphate (PIP3) thereby inhibiting PI3K induced activation of Akt (38,39), is one of the most commonly lost tumor suppressors in prostate cancer (40-42). PTEN loss of function often results in constitutively active Akt and may result in chronic activation of the proliferative genes.
The PI3K/Akt pathway activates mTOR and forkhead box protein O1 (FOXO1) and the MAPK/ERK cascade. Kinase inhibitors and dominant negative mutants of PI3K disrupt DHT-mediated activation of ERK and have supported a central role for the PI3K/Akt pathway in non-genomic AR signaling (13). Further, DHT-mediated activation of PI3K/Akt is AR-dependent (31,32,43).
Src pathway
Several studies have also implied the importance of Src in AR activation of kinase signaling cascades (17,32,43). In its inactivated conformation, interaction of the Src homology 2 (SH2) and Src homology 3 (SH3) domains causes autoinihibition of Src. AR interacts with the SH3 domain of Src relieving its autoinhibition and resulting in Src activation of the adaptor protein, Shc, a known upstream regulator of the MAPK pathway (44-46). AR-Src complexes may be noted in immunoprecipitation assays resulting in activation of Shc (29,43). Inhibition of the Src/MAPK pathway decreases DHT-induced ERK-1/2 phosphorylation (47).
In addition to Src-mediated direct activation of the ERK1/2 signaling cascade, Src may also activate the expression of receptors such as the insulin-like growth factor 1 receptor (IGF-1R) (47). Activated AR can also directly regulate IGF-1 gene expression as the IGF-1 promoter contains two AREs (48). However, data from quantitative RT-PCR studies shows expression of IGF-1R may be independent of AR-DNA binding. While the exact mechanism is not clearly elucidated, induction of IGF-1R expression appears to depend on Src/MAPK activation. Inhibition of the Src/MAPK pathway decreases IGF-1R expression and decreases ERK1/2 phosphorylation (47). IGF-1 signaling has been shown to promote prostate cell proliferation, migration, and tumor angiogenesis, resulting in prostate carcinogenesis and cancer progression (49).
Further, IGF-1 signaling can subsequently activate the PI3K/Akt pathway in prostate cancer cells (49). Increased IGF-1R binding of IGF-1 results in activation of the PI3K/Akt pathway, which can then regulate the action of proteins like mTOR and FOXO1. These processes activate multiple pathways including the Src/MAPK pathway early, subsequently IGF-1 pathway and later the PI3K/Akt pathway being temporally activated, ensuring a robust proliferation response to DHT.
Ras-Raf pathway
The Ras-Raf pathway is comprised of the Ras family of small GTPases and their downstream interaction of Raf kinase proteins. The Ras-Raf pathway is part of the larger Ras-Raf-MEK-MAPK-ERK signaling cascade that ultimately results in phosphorylation of the kinases ERK-1/2 (25,50,51). Dominant negative constructs of Raf-1 abrogated the DHT-induced ERK-1/2 but also reduced basal activity, which may have been present from residual hormone in the culture medium (13).
PKC pathway
Studies with PKC inhibitors indicate that AR utilizes PKC as a mediator of MAPK/ERK pathway activation (13,17,52). PKC kinase activity is regulated by both modulation of intracellular Ca2+ concentrations and diacylglycerol (DAG) binding to PKC itself (53). Mechanisms of non-genomic AR-mediated regulation of Ca2+ concentration appear to be cell-type dependent (11,54). Ca2+ could be released via internal stores and/or through influx from extracellular space. Interestingly, these mechanisms may not be blocked by AR antagonists (54). The etiology for cell type differences may indicate a role for cell type-specific AR cofactors (54). These findings also hint at the association of cytoplasmic AR or membrane-bound AR with plasma membrane receptors such as GPCRs or ion channels that may modulate intracellular Ca2+ ion concentration resulting in PKC activation.
Plasma membrane lipid rafts
AR in the plasma membrane may mediate DHT-induced activation of the PI3K/Akt, Ras-Raf, and PKC pathways (30). Non-cytoplasmic AR may be localized to the membrane and/or specialized liquid-ordered micro-domains within the lipid bilayer of the plasma membrane that are enriched with sphingolipids, caveolins, Src family kinases, G proteins and signaling mediators called “lipid rafts” (55,56). Several observations support the existence of and role for non-cytoplasmic AR in mediating non-genomic AR signaling. First, AR has been detected in the membrane and in lipid rafts. Cell membrane binding sites for androgens have been identified in several different cell types including rat osteoblasts (57), rat vascular cells (58), murine RAW 264.7 and IC-21 macrophages (19,59), murine splenic T lymphocytes (18), human prostate cancer cells (20,60), as well as in human prostate carcinoma cells (61). In PC3-AR cells, both AR and EGFR are found within plasma membrane lipid rafts (62). Co-localization of AR with caveolin-1 was found within lipid rafts of human aortic endothelial cells in response to testosterone treatment (33). Studies, using DHT-BSA, a large plasma membrane-impenetrable compound, showed binding of DHT to the membrane (18,19,63). Secondly, AR has been detected in complexes with multiple members of the lipid rafts. In caveolin-rich lipid rafts, a direct interaction was noted between caveolin-1 and a conserved nine-amino acid motif in the ligand-binding domain (LBD) of AR (30,64). AR forms a DHT-sensitive complex with the serine-threonine kinase Akt1 in caveolin-negative lipid rafts and mediates a non-genomic signal independently of Src and PI3K to activate Akt (65). Thirdly, androgens induce a rapid rise in intracellular Ca2+ concentration that can be blocked by pertussis toxin or by phospholipase C (PLC) inhibitor indicating a membrane-binding site for androgens that may be in close association with a GPCR (57,59,66). Finally, DHT-induced signaling cascades namely the Ras-Raf (67,68), MAPK/ERK (69,70), adenylyl cyclase (71), and PI3K(72), pathways have been shown to be enriched in lipid rafts. While specific membrane receptors, GPCR30 and membrane progesterone receptor (mPR), have been identified for estrogen and progesterone, respectively (73,74), to date, a membrane AR has not yet been purified or cloned. A clear understanding of the functional importance of non-cytoplasmic AR-mediated (membrane-bound or lipid raft) non-genomic signaling is lacking.
NonERK-mediated non-genomic AR signaling
Non-genomic AR signaling may occur without ERK participation through either PI3K/Akt/mTOR pathway activation or changes in intracellular Ca2+ concentration that result in activation of kinases such as PKA. For example, AR interaction with its p85α PI3K regulatory subunit may induce Akt-mediated phosphorylation of phosphatidylinositol-4,5-bisphosphate (PIP2) to generate the second messenger PIP3 (75,76), which then activates downstream signaling pathways important in cell proliferation (77). Alternatively, AR-directed Akt activation may result in FOXO1 phosphorylation resulting in its retention in the cytoplasm and subsequent degradation (78,79). In addition, liganded AR physically interacts with FOXO1 and impairs FOXO1-DNA binding ability and its ability to mediate pro-apoptotic pathways. Finally, Increased intracellular Ca2+ concentration and increased level of cAMP induced by GPCR or SHBGR also activate protein kinase A (PKA) (67,68), which then enhances prostate cancer cell proliferation (17,69).
Critically, each of these pathways is also capable of activating ERK1/2. This suggests that a number of signaling cascades may be activated in tandem with the MAPK/ERK pathway to induce proliferation (Figure 2). Crosstalk between these pathways further amplifies the signal and ensures that the cell responds to androgenic stimulation.
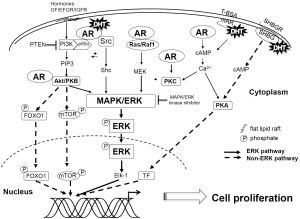
Crosstalk between genomic and non-genomic pathway
ERK has been shown to enhance AR transcriptional activity through the direct phosphorylation of AR and its coregulators (13). This autocrine loop could present a non-genomic mechanism to control AR transcriptional activity and could be important in cell adaptation to low androgen environments.
Non-genomic AR signaling mediated by induction of cAMP and PKA activation may involve SHBGR (80,81). DHT-SHBG also enhances AR transcriptional activity via phosphorylation of AR and AR coregulators facilitating their binding to AR (17). Thus, PKA can enhance prostate cancer cell proliferation and AR transcriptional activity even at very low levels of androgen (17,67,82). Thus, some of the non-genomic AR actions mediated by second messenger activation may influence the AR genomic responses (54) (Figure 3).
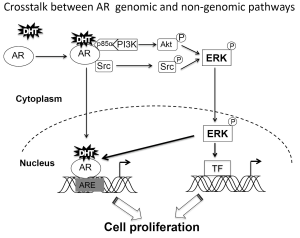
Implications in prostate cancer
Second-generation anti-androgens including MDV3100 and ARN-509 competitively target the activation of AR, its nuclear translocation and its genomic activity (68,69). However, non-genomic AR signaling that functions through cytoplasmic AR may still be active in MDV3100 treated prostate cancer cells. Prior studies have indicated that DHT-mediated non-genomic activation of ERK-1/2 in prostate cells are insensitive to anti-androgens specifically hydroxyflutamide and casodex (13). Thorough evaluation of the non-genomic AR axis is mandatory in assessing the effect of drugs targeting AR signaling in prostate cancer (13).
Conclusions
The existence of rapid, non-genomic AR signaling is incontrovertible. AR non-genomic regulation functions through the activation of intertwined complex signaling cascades resulting in expression of proliferative genes and responses. Non-genomic AR signaling may act to modulate genomic AR signaling and enable a coordinated, sustained and vigorous response to androgenic stimuli. Non-genomic AR signaling may represent a potential mechanism of resistance to anti-androgens.
Acknowledgements
None.
Footnote
Conflicts of Interest: The authors have no conflicts of interest to declare.
References
- He B, Kemppainen JA, Voegel JJ, et al. Activation function 2 in the human androgen receptor ligand binding domain mediates interdomain communication with the NH(2)-terminal domain. J Biol Chem 1999;274:37219-25. [PubMed]
- Loy CJ, Sim KS, Yong EL. Filamin-A fragment localizes to the nucleus to regulate androgen receptor and coactivator functions. Proc Natl Acad Sci U S A 2003;100:4562-7. [PubMed]
- Veldscholte J, Berrevoets CA, Brinkmann AO, et al. Anti-androgens and the mutated androgen receptor of LNCaP cells: differential effects on binding affinity, heat-shock protein interaction, and transcription activation. Biochemistry 1992;31:2393-9. [PubMed]
- Veldscholte J, Berrevoets CA, Zegers ND, et al. Hormone-induced dissociation of the androgen receptor-heat-shock protein complex: use of a new monoclonal antibody to distinguish transformed from nontransformed receptors. Biochemistry 1992;31:7422-30. [PubMed]
- Ozanne DM, Brady ME, Cook S, et al. Androgen receptor nuclear translocation is facilitated by the f-actin cross-linking protein filamin. Mol Endocrinol 2000;14:1618-26. [PubMed]
- Cardozo CP, Michaud C, Ost MC, et al. C-terminal Hsp-interacting protein slows androgen receptor synthesis and reduces its rate of degradation. Arch Biochem Biophys 2003;410:134-40. [PubMed]
- Shatkina L, Mink S, Rogatsch H, et al. The cochaperone Bag-1L enhances androgen receptor action via interaction with the NH2-terminal region of the receptor. Mol Cell Biol 2003;23:7189-97. [PubMed]
- Cato AC, Henderson D, Ponta H. The hormone response element of the mouse mammary tumour virus DNA mediates the progestin and androgen induction of transcription in the proviral long terminal repeat region. EMBO J 1987;6:363-8. [PubMed]
- Ham J, Thomson A, Needham M, et al. Characterization of response elements for androgens, glucocorticoids and progestins in mouse mammary tumour virus. Nucleic Acids Res 1988;16:5263-76. [PubMed]
- Beato M. Gene regulation by steroid hormones. Cell 1989;56:335-44. [PubMed]
- Falkenstein E, Tillmann HC, Christ M, et al. Multiple actions of steroid hormones--a focus on rapid, nongenomic effects. Pharmacol Rev 2000;52:513-56. [PubMed]
- Cato AC, Nestl A, Mink S. Rapid actions of steroid receptors in cellular signaling pathways. Sci STKE 2002;2002:re9.
- Peterziel H, Mink S, Schonert A, et al. Rapid signalling by androgen receptor in prostate cancer cells. Oncogene 1999;18:6322-9. [PubMed]
- McCubrey JA, Steelman LS, Chappell WH, et al. Roles of the Raf/MEK/ERK pathway in cell growth, malignant transformation and drug resistance. Biochim Biophys Acta 2007;1773:1263-84.
- Roberts PJ, Der CJ. Targeting the Raf-MEK-ERK mitogen-activated protein kinase cascade for the treatment of cancer. Oncogene 2007;26:3291-310. [PubMed]
- Mellström B, Naranjo JR. Mechanisms of Ca(2+)-dependent transcription. Curr Opin Neurobiol 2001;11:312-9. [PubMed]
- Heinlein CA, Chang C. The roles of androgen receptors and androgen-binding proteins in nongenomic androgen actions. Mol Endocrinol 2002;16:2181-7. [PubMed]
- Benten WP, Lieberherr M, Giese G, et al. Functional testosterone receptors in plasma membranes of T cells. FASEB J 1999;13:123-33. [PubMed]
- Benten WP, Lieberherr M, Stamm O, et al. Testosterone signaling through internalizable surface receptors in androgen receptor-free macrophages. Mol Biol Cell 1999;10:3113-23. [PubMed]
- Hatzoglou A, Kampa M, Kogia C, et al. Membrane androgen receptor activation induces apoptotic regression of human prostate cancer cells in vitro and in vivo. J Clin Endocrinol Metab 2005;90:893-903. [PubMed]
- Gatson JW, Kaur P, Singh M. Dihydrotestosterone differentially modulates the mitogen-activated protein kinase and the phosphoinositide 3-kinase/Akt pathways through the nuclear and novel membrane androgen receptor in C6 cells. Endocrinology 2006;147:2028-34. [PubMed]
- Rossomando AJ, Payne DM, Weber MJ, et al. Evidence that pp42, a major tyrosine kinase target protein, is a mitogen-activated serine/threonine protein kinase. Proc Natl Acad Sci U S A 1989;86:6940-3. [PubMed]
- De Luca A, Maiello MR, D’Alessio A, et al. The RAS/RAF/MEK/ERK and the PI3K/AKT signalling pathways: role in cancer pathogenesis and implications for therapeutic approaches. Expert Opin Ther Targets 2012;16 Suppl 2:S17-27. [PubMed]
- Dudley DT, Pang L, Decker SJ, et al. A synthetic inhibitor of the mitogen-activated protein kinase cascade. Proc Natl Acad Sci U S A 1995;92:7686-9. [PubMed]
- Santarpia L, Lippman SM, El-Naggar AK. Targeting the MAPK-RAS-RAF signaling pathway in cancer therapy. Expert Opin Ther Targets 2012;16:103-19. [PubMed]
- Gille H, Kortenjann M, Thomae O, et al. ERK phosphorylation potentiates Elk-1-mediated ternary complex formation and transactivation. EMBO J 1995;14:951-62. [PubMed]
- Marais R, Wynne J, Treisman R. The SRF accessory protein Elk-1 contains a growth factor-regulated transcriptional activation domain. Cell 1993;73:381-93. [PubMed]
- Yang SH, Yates PR, Whitmarsh AJ, et al. The Elk-1 ETS-domain transcription factor contains a mitogen-activated protein kinase targeting motif. Mol Cell Biol 1998;18:710-20. [PubMed]
- Unni E, Sun S, Nan B, et al. Changes in androgen receptor nongenotropic signaling correlate with transition of LNCaP cells to androgen independence. Cancer Res 2004;64:7156-68. [PubMed]
- Lu ML, Schneider MC, Zheng Y, et al. Caveolin-1 interacts with androgen receptor. A positive modulator of androgen receptor mediated transactivation. J Biol Chem 2001;276:13442-51. [PubMed]
- Sun M, Yang L, Feldman RI, et al. Activation of phosphatidylinositol 3-kinase/Akt pathway by androgen through interaction of p85alpha, androgen receptor, and Src. J Biol Chem 2003;278:42992-3000. [PubMed]
- Migliaccio A, Castoria G, Di Domenico M, et al. Steroid-induced androgen receptor-oestradiol receptor beta-Src complex triggers prostate cancer cell proliferation. EMBO J 2000;19:5406-17. [PubMed]
- Yu J, Akishita M, Eto M, et al. Src kinase-mediates androgen receptor-dependent non-genomic activation of signaling cascade leading to endothelial nitric oxide synthase. Biochem Biophys Res Commun 2012;424:538-43. [PubMed]
- Estrada M, Espinosa A, Müller M, et al. Testosterone stimulates intracellular calcium release and mitogen-activated protein kinases via a G protein-coupled receptor in skeletal muscle cells. Endocrinology 2003;144:3586-97. [PubMed]
- Sun M, Wang G, Paciga JE, et al. AKT1/PKBalpha kinase is frequently elevated in human cancers and its constitutive activation is required for oncogenic transformation in NIH3T3 cells. Am J Pathol 2001;159:431-7. [PubMed]
- Yuan ZQ, Sun M, Feldman RI, et al. Frequent activation of AKT2 and induction of apoptosis by inhibition of phosphoinositide-3-OH kinase/Akt pathway in human ovarian cancer. Oncogene 2000;19:2324-30. [PubMed]
- Cheng JQ, Altomare DA, Klein MA, et al. Transforming activity and mitosis-related expression of the AKT2 oncogene: evidence suggesting a link between cell cycle regulation and oncogenesis. Oncogene 1997;14:2793-801. [PubMed]
- Vivanco I, Sawyers CL. The phosphatidylinositol 3-Kinase AKT pathway in human cancer. Nat Rev Cancer 2002;2:489-501. [PubMed]
- Yuan TL, Cantley LC. PI3K pathway alterations in cancer: variations on a theme. Oncogene 2008;27:5497-510. [PubMed]
- Hollander MC, Blumenthal GM, Dennis PA. PTEN loss in the continuum of common cancers, rare syndromes and mouse models. Nat Rev Cancer 2011;11:289-301. [PubMed]
- Marsit CJ, Zheng S, Aldape K, et al. PTEN expression in non-small-cell lung cancer: evaluating its relation to tumor characteristics, allelic loss, and epigenetic alteration. Hum Pathol 2005;36:768-76. [PubMed]
- Yoshimoto M, Cunha IW, Coudry RA, et al. FISH analysis of 107 prostate cancers shows that PTEN genomic deletion is associated with poor clinical outcome. Br J Cancer 2007;97:678-85. [PubMed]
- Kousteni S, Bellido T, Plotkin LI, et al. Nongenotropic, sex-nonspecific signaling through the estrogen or androgen receptors: dissociation from transcriptional activity. Cell 2001;104:719-30. [PubMed]
- Ling Y, Maile LA, Lieskovska J, et al. Role of SHPS-1 in the regulation of insulin-like growth factor I-stimulated Shc and mitogen-activated protein kinase activation in vascular smooth muscle cells. Mol Biol Cell 2005;16:3353-64. [PubMed]
- Biedi C, Panetta D, Segat D, et al. Specificity of insulin-like growth factor I and insulin on Shc phosphorylation and Grb2 recruitment in caveolae. Endocrinology 2003;144:5497-503. [PubMed]
- Song RX, McPherson RA, Adam L, et al. Linkage of rapid estrogen action to MAPK activation by ERalpha-Shc association and Shc pathway activation. Mol Endocrinol 2002;16:116-27. [PubMed]
- Pandini G, Mineo R, Frasca F, et al. Androgens up-regulate the insulin-like growth factor-I receptor in prostate cancer cells. Cancer Res 2005;65:1849-57. [PubMed]
- Wu Y, Zhao W, Zhao J, et al. Identification of androgen response elements in the insulin-like growth factor I upstream promoter. Endocrinology 2007;148:2984-93. [PubMed]
- Grimberg A. Mechanisms by which IGF-I may promote cancer. Cancer Biol Ther 2003;2:630-5. [PubMed]
- Molina JR, Adjei AA. The Ras/Raf/MAPK pathway. J Thorac Oncol 2006;1:7-9. [PubMed]
- Chappell WH, Steelman LS, Long JM, et al. Ras/Raf/MEK/ERK and PI3K/PTEN/Akt/mTOR inhibitors: rationale and importance to inhibiting these pathways in human health. Oncotarget 2011;2:135-64. [PubMed]
- Toullec D, Pianetti P, Coste H, et al. The bisindolylmaleimide GF 109203X is a potent and selective inhibitor of protein kinase C. J Biol Chem 1991;266:15771-81. [PubMed]
- Newton AC. Protein kinase C: structure, function, and regulation. J Biol Chem 1995;270:28495-8. [PubMed]
- Foradori CD, Weiser MJ, Handa RJ. Non-genomic actions of androgens. Front Neuroendocrinol 2008;29:169-81. [PubMed]
- Simons K, Toomre D. Lipid rafts and signal transduction. Nat Rev Mol Cell Biol 2000;1:31-9. [PubMed]
- Hager MH, Solomon KR, Freeman MR. The role of cholesterol in prostate cancer. Curr Opin Clin Nutr Metab Care 2006;9:379-85. [PubMed]
- Lieberherr M, Grosse B. Androgens increase intracellular calcium concentration and inositol 1,4,5-trisphosphate and diacylglycerol formation via a pertussis toxin-sensitive G-protein. J Biol Chem 1994;269:7217-23. [PubMed]
- Figueroa-Valverde L, Luna H, Castillo-Henkel C, et al. Synthesis and evaluation of the cardiovascular effects of two, membrane impermeant, macromolecular complexes of dextran-testosterone. Steroids 2002;67:611-9. [PubMed]
- Guo Z, Benten WP, Krücken J, et al. Nongenomic testosterone calcium signaling. Genotropic actions in androgen receptor-free macrophages. J Biol Chem 2002;277:29600-7. [PubMed]
- Kampa M, Papakonstanti EA, Hatzoglou A, et al. The human prostate cancer cell line LNCaP bears functional membrane testosterone receptors that increase PSA secretion and modify actin cytoskeleton. FASEB J 2002;16:1429-31. [PubMed]
- Stathopoulos EN, Dambaki C, Kampa M, et al. Membrane androgen binding sites are preferentially expressed in human prostate carcinoma cells. BMC Clin Pathol 2003;3:1. [PubMed]
- Bonaccorsi L, Nosi D, Quercioli F, et al. Prostate cancer: a model of integration of genomic and non-genomic effects of the androgen receptor in cell lines model. Steroids 2008;73:1030-7. [PubMed]
- Konoplya EF, Popoff EH. Identification of the classical androgen receptor in male rat liver and prostate cell plasma membranes. Int J Biochem 1992;24:1979-83. [PubMed]
- Acconcia F, Bocedi A, Ascenzi P, et al. Does palmitoylation target estrogen receptors to plasma membrane caveolae? IUBMB Life 2003;55:33-5. [PubMed]
- Cinar B, Mukhopadhyay NK, Meng G, et al. Phosphoinositide 3-kinase-independent non-genomic signals transit from the androgen receptor to Akt1 in membrane raft microdomains. J Biol Chem 2007;282:29584-93. [PubMed]
- Sun YH, Gao X, Tang YJ, et al. Androgens induce increases in intracellular calcium via a G protein-coupled receptor in LNCaP prostate cancer cells. J Androl 2006;27:671-8. [PubMed]
- Nazareth LV, Weigel NL. Activation of the human androgen receptor through a protein kinase A signaling pathway. J Biol Chem 1996;271:19900-7. [PubMed]
- Clegg NJ, Wongvipat J, Joseph JD, et al. ARN-509: a novel antiandrogen for prostate cancer treatment. Cancer Res 2012;72:1494-503. [PubMed]
- Tran C, Ouk S, Clegg NJ, et al. Development of a second-generation antiandrogen for treatment of advanced prostate cancer. Science 2009;324:787-90. [PubMed]
- Liao WX, Feng L, Zhang H, et al. Compartmentalizing VEGF-induced ERK2/1 signaling in placental artery endothelial cell caveolae: a paradoxical role of caveolin-1 in placental angiogenesis in vitro. Mol Endocrinol 2009;23:1428-44. [PubMed]
- Ostrom RS, Gregorian C, Drenan RM, et al. Receptor number and caveolar co-localization determine receptor coupling efficiency to adenylyl cyclase. J Biol Chem 2001;276:42063-9. [PubMed]
- Chen L, Xiong S, She H, et al. Iron causes interactions of TAK1, p21ras, and phosphatidylinositol 3-kinase in caveolae to activate IkappaB kinase in hepatic macrophages. J Biol Chem 2007;282:5582-8. [PubMed]
- Filardo EJ, Quinn JA, Frackelton AR Jr, et al. Estrogen action via the G protein-coupled receptor, GPR30: stimulation of adenylyl cyclase and cAMP-mediated attenuation of the epidermal growth factor receptor-to-MAPK signaling axis. Mol Endocrinol 2002;16:70-84. [PubMed]
- Zhu Y, Bond J, Thomas P. Identification, classification, and partial characterization of genes in humans and other vertebrates homologous to a fish membrane progestin receptor. Proc Natl Acad Sci U S A 2003;100:2237-42. [PubMed]
- Zhang Y, Gan B, Liu D, et al. FoxO family members in cancer. Cancer Biol Ther 2011;12:253-9. [PubMed]
- Fresno Vara JA, Casado E, de Castro J, et al. PI3K/Akt signalling pathway and cancer. Cancer Treat Rev 2004;30:193-204. [PubMed]
- Cantley LC. The phosphoinositide 3-kinase pathway. Science 2002;296:1655-7. [PubMed]
- Brunet A, Bonni A, Zigmond MJ, et al. Akt promotes cell survival by phosphorylating and inhibiting a Forkhead transcription factor. Cell 1999;96:857-68. [PubMed]
- Huang H, Tindall DJ. FOXO factors: a matter of life and death. Future Oncol 2006;2:83-9. [PubMed]
- Nakhla AM, Romas NA, Rosner W. Estradiol activates the prostate androgen receptor and prostate-specific antigen secretion through the intermediacy of sex hormone-binding globulin. J Biol Chem 1997;272:6838-41. [PubMed]
- Fortunati N, Fissore F, Fazzari A, et al. Sex steroid binding protein exerts a negative control on estradiol action in MCF-7 cells (human breast cancer) through cyclic adenosine 3',5'-monophosphate and protein kinase A. Endocrinology 1996;137:686-92. [PubMed]
- Ikonen T, Palvimo JJ, Kallio PJ, et al. Stimulation of androgen-regulated transactivation by modulators of protein phosphorylation. Endocrinology 1994;135:1359-66. [PubMed]