Near-infrared fluorescence and nuclear imaging and targeting of prostate cancer
Introduction
Prostate cancer (PCa) is a heterogeneous disease with the biological potential to develop an aggressive and lethal phenotype (1). PCa is the most common cancer diagnosed in men in the Western countries but only one of every 8-10 patients diagnosed with PCa will die from this disease (2,3). Thus it is important to develop effective methods not only to diagnose PCa but also to effectively select patients who need to be treated and avoid unnecessary treatment. It is also vital to target tumors that have recurred, escaping initial treatments and developing into metastatic disease. Molecular imaging of PCa using such conventional imaging methodologies as X-ray computed tomography (CT), magnetic resonance imaging (MRI) and ultrasound has been successful for detecting organ-confined or metastatic disease for disease staging and companion diagnosis and prognosis (4,5). However, these techniques also show the limitations of current cancer-specific imaging and cannot reliably delineate the occurrence, the location, and the biochemical status of cancer and cancer metastases. Positron emission tomography (PET)/single-photon emission computed tomography (SPECT) for nuclear imaging have distinct advantages over conventional imaging, with unique noninvasive properties capable of monitoring the metabolic and molecular characteristics of cancer cells. These approaches utilize short-lived nuclear tracers and acquire signals emanating from the body after administration of imaging agents that target cancer-specific alterations, including glucose, amino acid and fatty acid metabolism, receptor status, cellular proliferation, tumor hypoxia and blood flow (6). Currently, several PET tracers have been applied for clinical imaging of both early- and late-stage PCa, such as 18F-fluorodeoxyglucose (FDG), choline (11C and 18F labeled), 18F-dihydrotestosterone (FDHT) and sodium 18F-fluoride (NaF). These molecular imaging agents can potentially greatly elevate our ability to diagnose, prognose and monitor treatment responses in PCa patients.
Near-infrared fluorescence (NIRF) imaging agents can potentially increase the sensitivity and specificity of cancer diagnosis because NIRF have low autofluorescence, tissue absorbance, and scatter at NIR wavelengths (700-900 nm) (7). Once the specificity of these agents is established, a number of modifications can be made including the conversion of these agents into effective nuclear imaging probes, or conjugating these agents with effective therapeutic drugs to target cancer metastasis. The NIRF approach shares common physical properties with nuclear imaging techniques, such as the potential use of tracer administration of a contrast agent, which enables the development of combinational use of both techniques for cancer imaging (see below). Most conventional approaches for utilizing NIR dyes in cancer imaging require chemical conjugation of NIR fluorophores with appropriate cancer-targeting moieties such as peptides, antibodies, aptamers, growth factors and metabolic substrates (8-11). However, these approaches are less efficient because of tumor cell heterogeneity. Only a limited number of cancer cells may express the specified cell surface receptors or ligands, and the constant evolution of cancer cells known to occur within a tumor could alter cancer cell surface properties (12,13). The specificity and affinity of targeting ligands can also be altered subtly following chemical conjugation (14). Our group has recently discovered a novel class of NIR heptamethine carbocyanine dyes that can be used as dual imaging and targeting agents, and demonstrated their preferential accumulation and retention in cancer but not normal cells, enabling cancer-specific targeting without the need for chemical conjugation (15,16). These agents greatly fulfill the unmet needs for specific imaging of PCa despite its heterogeneity, which to some extent is inadequately assessed by conventional methods. In this review, we will discuss recent advances in the development of novel NIRF, NIRN and NIRF-derived agents and techniques for imaging and targeting localized and metastatic PCa.
NIRF imaging of prostate cancer
NIRF imaging agents
Indocyanine green (ICG) is a noninvasive NIRF imaging dye that has been used clinically for more than 50 years for ophthalmic angiography and to determine cardiac output and liver blood flow and function (7,17). This tricarbocynanine dye is also used in cancer patients to map sentinel lymph nodes, for the detection of some tumors due to their enhanced angiogenesis, and for angiography during reconstructive surgery as the only NIRF agent approved by the United States Food and Drug Administration (FDA) (18-21). Most human NIR imaging studies employ ICG within the blood and lymphatic vasculatures. ICG and its derivatives are widely used clinically and show reasonable NIR features (ex/em 760-785/820-840 nm) and the capability to image normal but not cancerous tissues, generating weaker fluorescent properties (i.e., lower extinction coefficients) in comparison to the NIRF described herein (7,17). Several new NIRF agents have been developed including heptamethine carbocyanine dyes. Some of these agents have become commercially available in recent years, such as Cy5.5 (22) and IRDye 800-CW (23), which have been coupled with peptides or antibodies and successfully used for the targeted visualization of neoplastic tumors in animal models. Marshall et al., for example, reported a safety and toxicity study of the NIRF dye IRDye 800CW in rats that showed no evidence of organ toxicity based on the hematologic, clinical chemistry and histopathologic analyses of tissues harvested from the experimental animals (23). These new NIRF agents offer great promise for future clinical applications of NIRF imaging agents.
NIRF imaging of prostate cancer
Conventional application of NIRF agents in PCa imaging use the chemical conjugation of specified NIRF agents to cancer cell-surface targeting moieties, such as peptides and antibodies recognized as tumor-specific biomarkers (19). One such biomarker is prostate-specific antigen (PSA), an androgen receptor (AR) target gene expressed almost exclusively by prostate epithelium. PSA, expressed by both benign and malignant prostate epithelium, potentially reflects active AR signaling activity (24). Since AR-mediated cell signaling pathways are known to determine human PCa initiation and progression, and AR expression and activity are elevated in some castration-resistant PCa (CRPC) (25), many groups have sought to image PCa through the use of PSA as a biomarker. PSA is initially produced as a catalytically active serine protease (free PSA), released subsequently into the perivascular space, by rapid and irreversible conversion to non-catalytic forms (26,27). In a recent study, Ulmert et al. described a new approach to specifically image tumor-associated free PSA in multiple preclinical models with 89Zr-labeled 5A10, a novel radiotracer consisting of a monoclonal antibody that specifically recognizes an epitope adjacent to the catalytic cleft of PSA (28). In line with the same principle, Ho et al. developed an enzymatically cleavable peptide sequence labeled with NIR fluorophores (ex/em 740/770 nm), PSA750, which is optically quenched (>95%) and only becomes fluorescent upon cleavage by enzymatically-active PSA, yielding a significantly increased NIR signal from the site where PSA is secreted or deposited (29). Currently, serum PSA levels are widely used clinically as an indicator for primary screening and a biomarker for therapeutic responses, but PSA expression alone cannot distinguish benign from malignant prostate epithelium (30,31). The noninvasive imaging tools developed for measuring tumor-associated PSA expression could more clearly reflect AR-driven changes in PSA expression and could be used to supplement the current clinical PSA test.
Another cell-surface antigen for PCa is prostate-specific membrane antigen (PSMA). PSMA, elevated in CRPC, is a plausible target for imaging probe development (32-34). Radioactive 111In-labeled PSMA antibody has been used as a reagent in SPECT imaging. In addition to being a biomarker of PCa, PSMA has been proposed as a target for image-guided surgery due to its cell surface-expressing characteristics. To better identify prostate tumor margins during surgery, Nakajima et al. synthesized an activatable anti-PSMA monoclonal antibody (J591)-NIR fluorophore (ICG) conjugate and tested it in a PC-3 prostate tumor xenograft mouse model (35). Prior to binding to PSMA and cellular internalization, the conjugate yielded little light. However, upon internalization and cleavage, NIR-ICG intensity in PCa was elevated by 18-fold, permitting the detection of PSMA+ PC-3 but not PSMA- PC-3 tumors for up to 10 days after a low-dose (0.25 mg/kg) injection. In another study, Humblet et al. synthesized a single nucleophile-containing small molecule specific for the active site of PSMA enzyme that is chemically conjugated to an ICG derivative (10). This conjugate shows high-affinity binding to PSMA in xenograft prostate tumors by NIRF imaging. These NIRF-based PSMA-targeting imaging approaches are reproducible at the cellular level in PCa as well. Liu et al. developed a NIRF imaging probe (Cy5.5-CTT-54.2) by chemical conjugation of a Cy5.5 derivative with a potent PSMA inhibitor (CTT-54.2) (36). The probe displays high potency against PSMA and has demonstrated successful application for specifically labeling PSMA+ LNCaP PCa cells in both 2D and 3D cell culture conditions.
Another approach to developing targeted molecules relies on the aberrant metabolic pathways established by cancer cells. Cancer cells have been observed to exhibit altered metabolism and increased requirements for glucose and glutamine, which is facilitated by the overexpression of glucose transporter proteins (GLUTs) (37-39). FDG is a glucose analog that has been used extensively in cancer detection and therapeutic monitoring in the form of a 18F-FDG probe, detected by PET (40,41). The 18F-FDG probe, however, has several limitations, such as an extremely short half-life for following by positron-emitting nuclides, exhibiting low spatial resolution, being a radioactive compound, and is also abundantly taken up by tissues with high basal metabolic rates, such as the brain. To overcome these intrinsic PET imaging limitations, an NIRF imaging approach has been proposed and developed as a replacement for metabolic imaging using a similar targeting principle. Korotcov et al. (42) designed an NIRF probe (cypate) chemically conjugated to one or more glucosamine (GlcN) moieties, a common substrate for all 4 isoforms of GLUTs with higher affinity for GLUT2 than glucose (39,42,43), and demonstrated good uptake of the GlcN-linked NIRF probe in both PC-3 cell culture and live mice. In summary, diverse molecular imaging approaches from different research groups have demonstrated the effectiveness of targeting cell surface-based biomarkers or the metabolic differences between normal and cancer cells for monitoring PCa growth and recognizing the surgical margins of PCa tumors during surgery.
NIRF imaging of metastases in experimental prostate cancer models
Imaging of lymph node metastases
Early-stage PCa develops seminal vesicle invasion and micrometastases to surrounding lymph nodes (LNs). Pelvic LN dissection (PLND) is widely used in the clinic for nodal staging and assessing LN metastases in PCa (44). However, this method is invasive and underestimates LN involvement; 40-50% of patients are found to have metastatic LNs outside the standard resection area (45,46). There is an unmet need for more accurate noninvasive diagnostic techniques. Abnormal lymphatic function has been associated with a wide spectrum of diseases and is also intimately involved in cancer metastases (7,47). Preclinical cancer studies show apparent dilation and proliferation of tumor-draining lymphatic vessels and tumor-draining lymph node remodeling (47), which offers a targeting opportunity for noninvasive lymphatic imaging with NIRF probes. As the only NIRF agent approved by the FDA, ICG has been used noninvasively in humans to uniquely detect blood and lymphatic vasculatures and used intraoperatively in sentinel LN mapping for visualization of tumor-draining LNs in several types of cancers including PCa (7). Van der Poel et al. recently reported an approach integrating ICG with a radioisotope (99mTc) and nanoparticles for injection into the prostate prior to surgery for improved surgical guidance via multimodal imaging, particularly fluorescence imaging (48). Ex vivo analysis further revealed a strong correlation between the radioactive and fluorescent content in the excised LNs. Similar detection outcomes in the percentages of PCa metastases to LNs by NIRF imaging (63%) and μPET/CT (64%) were also reported by Hall et al., further confirmed by pathological examination (49). Alternatively, targeting PCa biomarker molecules as a conventional approach facilitates NIRF detection of LN metastases. Cai et al. (50) synthesized NIRF dye (Alexa Fluor 680) conjugated BBN[7-14]NH2 peptides that target gastrin-releasing peptide receptors (GRPRs), which showed high densities on the cell membranes of prostatic intraepithelial neoplasia (PIN), primary PCa and invasive prostatic carcinomas with predominately negative expression in normal prostate tissue and benign prostatic hyperplasia (BPH) (51,52), in an orthotopic PC-3 xenograft mouse model. Within 2-hour post-injection, the conjugate reached the highest binding specificity and affinity in GRPR+ cancer in vivo, and LN and peritoneal metastases were detected by NIRF imaging, which was later confirmed by histopathology. These studies across different groups suggest the promising future clinical utility of NIRF imaging in PCa staging and laparoscopic LN dissection, which would be boosted by improved imaging devices with better signal capture from deep tissue in the near future.
Imaging of bone metastases
PCa patients develop lethal bone metastases in the late stage of disease progression. Current clinical examinations of PCa bone metastases rely on PET/SPECT/CT scans. While potential limitations in the use of these radiotracers in PET and SPECT imaging including exposure to radiation, difficulty in synthesizing radioactive tracers, and short interval of tracer retention in tumors, they are nevertheless preferred in patients due to the variability of tumor depth in patients. NIRF imaging, however, offers companion diagnostic and prognostic value by having a potentially better safety profile, lower cost and desirable sensitivity for the diagnosis of cancer metastasis. Recent advances have been made in multiple NIRF imaging approaches to the visualization of bone metastases utilizing different targeting strategies. We previously synthesized a NIR quantum dot (QD) probe (QD800) conjugated to an anti-PSMA antibody and demonstrated the specific recognition of C4-2B xenografts residing in mouse tibia by this NIRF conjugate using the IVIS Imaging System (53). The maximal light emission was detected 30 minutes after intravenous injection of QD800 conjugate in mice. In addition to the conventional chemical conjugation method, we were also able to detect metastatic PCa cells in either bone metastases or in the precursor state as circulating tumor cells (CTCs) by a novel class of heptamethine carbocyanine dyes (see below) in an orthotopic ARCaPM xenograft mouse model (16).
Novel heptamethine carbocyanine fluorescence dye-based imaging of prostate cancer
Heptamethine carbocyanine fluorescence imaging agents
We recently discovered a novel class of NIRF heptamethine carbocyanine dyes, IR-783 and MHI-148, which is an effective cancer-specific imaging agent. These agents show preferential uptake and retention in cancer but not normal cells (15,16). By conjugating chemotherapeutic agents with these dyes, we observed tumor-specific cell kill without cytotoxicity in host mice, suggesting the potential use of these carbocyanine dyes as carriers for cancer-specific targeting by small molecules (see below). The advantages of this new class of NIRF as imaging agents are: (I) they have relatively low molecular weights that facilitate their effective uptake into both localized and metastatic cancers; (II) they can be synthesized in pure form and are stable upon storage; (III) they are taken up by many different types of cancer cells, including circulating or disseminated tumor cells and cancer tissues regardless of their cell-surface properties and their plasticity; and (IV) they have the potential of recognizing live versus dead cells and therefore can be used for follow-up in patients subjected to treatment by hormonal, radiation and chemotherapeutic agents. We found that these dyes can be retained in established PCa cell lines (C4-2, PC-3 and ARCaPM) with the dyes enriched in the mitochondria and lysosomes, but not in normal prostatic epithelial and fibroblast cells. In an orthotopic ARCaPM xenograft mouse model receiving intraperitoneal injection of low dose of IR-783 (10 nmol/20 g), the NIRF signals were specifically detected in the primary tumor and its associated bone metastases within 24 hours by fluorescence optical imaging. Similar targeting was also found in spontaneously developed prostate and colon tumors in the TRAMP PCa and ApcMin/+ colon cancer mouse models, respectively (16). Recently, we extended these studies to demonstrate successful detection of dye uptake in freshly harvested human PCa tissue xenografts as well as CTCs using these novel NIRF agents. Additionally this novel class of NIRF showed no systemic toxicity when mice were given a 100-fold excess of the imaging dose of NIRF.
Near-infrared nuclear imaging of prostate cancer using novel heptamethine carbocyanine dyes conjugated to 64Cu as PET probes
Although early detection of PCa by blood tests for elevated levels of PSA has led to early treatment and a reduction in death rates, PSA level alone does not distinguish between PCa and normal conditions that cause elevated PSA (30,31). Because PCa can be a very slow-growing cancer, even confirmation of PCa cells in a biopsy gives no indication whether an active disease will progress within the individual’s lifetime. As a result many patients receive painful repeated needle biopsies when PSA is found to be elevated. Successful management of prostate cancer requires early detection, appropriate risk assessment, and optimal treatment to avoid the development of CRPC with potential of lethal progression (54). Nuclear imaging is an attractive modality for the detection and characterization of disease because it is non-invasive, quantitative, provides dynamic real-time data, and allows the diagnosis and follow-up of patients undergoing therapy (6). Whether the development of new nuclear imaging probes could offer the opportunity of differentiating indolent from aggressive prostate tumors remains untested.
Different radionuclide-based imaging agents for planar, PET and SPECT imaging are currently used in the clinic with some under development for PCa. Clinical agents include the bone agent methylene diphosphonate (MDP, 99mTc labeled), the metabolic agent 18F-FDG, and receptor targeted radiolabeled monoclonal antibodies including the PSMA-based ProstaScint. Agents in development for PCa include acetate (11C labeled), choline (11C and 18F labeled), 1-aminocyclobutane-1-carboxylic acid (11C and 18F labeled), radiolabeled AR binding compounds, radiolabeled peptides and small molecules for receptors overexpressed in PCa or PCa-associated tumor neovasculature. Despite a variety of probes using nuclear imaging modalities, neither the detection of minimal disease nor the prediction of indolent versus aggressive PCa has been accomplished. A simple, accurate method for localizing cancer within the prostate for focal therapy also remains elusive.
As part of our extensive search for agents that might have cancer-specific uptake in PCa, we reported the discovery of a new class of heptamethine carbocyanine dyes that allow detection of human and mouse tumors with a high degree of sensitivity and specificity (15,16). To further improve the sensitivity and clinical utility of this class of carbocyanine dyes for deep-tissue detection of tumors, we modified the dye by conjugating it with a positron-emitting radionuclide (see below) and tested its feasibility in cultured human PCa cells and metastatic prostate tumors in mice.
We synthesized a PET/NIRF probe PC-1001 by conjugating MHI-148 with a DOTA chelator and subsequent chelating with 64Cu for independent PET and fluorescence imaging (Figure 1). This two-component probe is the first example of novel tumor-specific fluorescent dye with both targeting and detection properties in one component and a second component (64Cu-DOTA) with the capability to perform nuclear imaging. In contrast to all other multimodal probes reported to date, the tumor-targeting component is separate from the detection component and thus needs a minimum of three components. The NIRF imaging modality has the merits of simplicity, convenience, and high throughput. The NIRF property of the probe simplifies the early stages of its development for in vitro and in vivo optimization of parameters and its validity prior to final live animal PET imaging. NIRF alone has inherent shortcomings such as its low resolution and non-quantitative nature. The sensitivity and resolution of NIRF imaging is severely influenced by position and depth of the imaging probes in the body. The positron emitting property of the conjugated probe can overcome the shortcomings of NIRF and provide high sensitivity and deep-tissue spatial resolution for initial detection of primary tumors and their metastatic lesions. Tumor size can then be monitored over time with NIRF imaging. Our recent results showed successful PC-1001/NIRF image of a mouse with a metastatic RANKL-overexpressing LNCaP tumor demonstrating two superficial tumors in the mouse (55) (Figure 2A), while the PC-1001/PET image of the same mouse revealed an extra tumor in a deep location (Figure 2B).
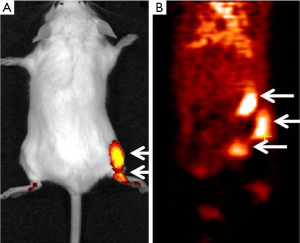
The dual-modality PC-1001 molecular imaging probe described above has demonstrated its applicability for tumor detection and quantitative image analysis in a metastatic PCa mouse model. The PC-1001 probe is accumulated specifically in cancerous tissue with good contrast to normal tissue. This probe could be useful in assisting the evaluation of anti-cancer therapies, anti-cancer drug discovery, and cancer-related biological studies. Further biological and toxicological evaluation of this imaging agent is ongoing, with the aim of advancing into clinical trials. Other laboratories have also designed dual-modality PCa imaging probes. In a recent study, Ghosh et al. designed a multimodality chelation scaffold (MMC) that combined a radiometal chelating agent (64Cu) and NIRF dye (a IRDye 800CW derivative) (56). Using MMC-immunoconjugate to target an epithelial cell adhesion molecule (EpCAM), which shows elevated expression with PCa biochemical recurrence and correlation with Gleason scores (57,58), multimodal imaging studies indicated higher tumor accumulation of the dual-labeled conjugate compared to either single-labeled agent in a PC-3 tumor-bearing mouse model. Another example is EphB4, a key member of the Eph receptors overexpressed in numerous tumor types including PCa (59-61), which has been developed as a promising imaging target. Zhang et al. reported the visualization of EphB4+ PC-3M PCa xenografts with an EphB4-binding peptide (TNYL-RAW)-nanoparticle conjugate dually labeled with NIRF fluorophores (Cy7) and a radioisotope 111In, with both NIRF and NIRN imaging (62). The high accumulation of dually labeled peptide in PC-3M tumor could be significantly reduced after co-injection with an excess amount of unlabeled peptide, suggesting the specificity of this imaging probe for recognizing EphB4 receptor. These reports support the promise of dual labeling imaging approaches for improved sensitivity and depth of imaging compared to NIRF imaging alone.
Novel heptamethine carbocyanine drug conjugates for targeting castration-resistant and bone metastatic prostate cancer
Because CRPC is considered as the most advanced and lethal form of PCa, we synthesized a number of heptamethine carbocyanine drug conjugates to target metastatic PCa more efficiently. The basic principle of these studies is to use heptamethine carbocyanine dyes as the drug carriers. They will be covalently conjugated to drugs through a linker composed of either an ester or a peptide bond. Upon uptake, these dye-drug conjugates accumulate exclusively in tumor tissues. Results using IR-783-docetaxel conjugate showed metastatic PCa tumor shrinkage in mouse tibia (Figure 3). While mice treated with this dye-drug conjugate showed no visible toxicity or reduced body weights, control mice treated with docetaxel alone had dramatically reduced body weights and over 50% mortality. These encouraging results have been shown to be repeatable using other dye-drug conjugates. Future safety studies are necessary before moving this concept into the clinic.
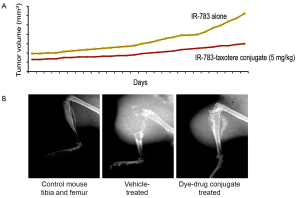
Mechanisms of uptake of heptamethine carbocyanine dyes by prostate cancer cells
We have studied the underlying mechanisms by which heptamethine carbocyanine dyes are taken up specifically into cancer but not normal cells. We investigated the effects of tumor hypoxia, a common condition found in a wide range of cancer cells or solid tumors, on the uptake of heptamethine cyanines into cancer cells. In our unpublished studies (Wu and Shao et al. 2013), unlike ICG that has a relatively low value of tumor-to-background ratio at 1.4-1.7 (63), measured at 24 hours after the administration of the dye into tumor-bearing mice, we showed that this ratio increased to 9.1 in tumors when MHI-148 was used, which was further enhanced by 2-fold when cells were maintained under hypoxic conditions. We found that a superfamily of organic anion carrier transporters, named organic anion-transporting peptides (OATPs), plays key roles accounting for the differential uptake of these dyes into cancer but not normal cells. We conducted microarray analyses and later confirmed by qRT-PCR, western blots and immunohistochemistry that specific isoforms of OATPs might be responsible for cancer-specific dye uptake. Currently there are 11 known human OATPs classified into 6 families and subfamilies on the basis of their amino acid sequence homologies, which facilitate the transport of a large number of substrates, including organic acids, drugs and hormones into cells in a highly substrate- and pathophysiologic-dependent manner (64). The dye uptake and retention in cancer cells can be blocked completely by several competitive inhibitors of OATPs, such as bromosulfophthalein (16). Increased expression of select OATPs, such as OATP1B3 and OATP2B1, by either aberrant gene regulation or genetic variation, has been reported in clinical PCa, particularly during progression to a CRPC state (64-67). Notably, OATP1B3 also serves as a testosterone transporter (68,69), and its transporting activity may be further exacerbated by low levels of testosterone in CRPC (67,70,71). In addition, we and another group dissected a direct regulatory mechanism of OATP1B3 expression by hypoxia through HIF1α in PCa cells, which provides a functional link among different mediators that enhance dye uptake. Specific accumulation of dye and dye-drug conjugates in cancer cells can also be attributed to the high-affinity binding of this class of dyes once they enter into cells to interact with nucleic acids and proteins, which warrants further investigation.
Conclusions and future perspectives
We demonstrated the specific uptake and retention of a novel class of NIRF imaging agents, heptamethine carbocyanine dyes that can be used for imaging solid tumors and CTCs freshly harvested from human patients. This new class of NIRF imaging agents has been successfully tested as dual NIRF and NIRN agents using PET/SPECT to detect PCa bone and soft tissue metastases in experimental models. By further conjugating this class of NIRF/NIRN agents with cancer therapeutic drugs, we found that they can serve as drug carriers for the safe delivery of chemotherapies to experimental tumors. We found also that the differential uptake of this class of negatively charged carbocyanine dyes into cancer but not normal cells was largely due to the presence of specific isoforms of OATPs, coupled with specific metabolisms regulated by hypoxia and mitochondrial membrane potentials and the physical chemical reactions of this class of dyes when in close contact with nucleic acids and proteins in cancer cells. While promising data have accumulated thus far, crucial evaluation of the PK, PD and toxicity of the dyes and dye-drug conjugates are needed before this group of novel compounds can be moved into the clinic for improved cancer diagnosis, prognosis and treatment.
Acknowledgements
Funding: This work was supported by NIH Grants 5P01CA098912, R01CA122602, Prostate Cancer Foundation Challenge Award, and Board of Governors Chair in Cancer Research fund (L.W-K.C.).
The authors thank Mr. Gary Mawyer for his editorial assistance.
Footnote
Conflicts of Interest: Dr. Dongfeng Pan and Dr. Leland W. K. Chung are the co-owner of Imol Radiopharmaceuticals, LLC (Charlottesville, Virginia) and Dr. Leland W. K. Chung is a co-owner of Da Zen Theranostics, LLC (Los Angeles, California).
References
- Scher HI, Heller G. Clinical states in prostate cancer: toward a dynamic model of disease progression. Urology 2000;55:323-7. [PubMed]
- Siegel R, Naishadham D, Jemal A. Cancer statistics, 2012. CA Cancer J Clin 2012;62:10-29. [PubMed]
- Cooperberg MR, Carroll PR, Klotz L. Active surveillance for prostate cancer: progress and promise. J Clin Oncol 2011;29:3669-76. [PubMed]
- O’ Donoghue PM, McSweeney SE, Jhaveri K. Genitourinary imaging: current and emerging applications. J Postgrad Med 2010;56:131-9. [PubMed]
- Marks L, Young S, Natarajan S. MRI-ultrasound fusion for guidance of targeted prostate biopsy. Curr Opin Urol 2013;23:43-50. [PubMed]
- Fox JJ, Schöder H, Larson SM. Molecular imaging of prostate cancer. Curr Opin Urol 2012;22:320-7. [PubMed]
- Sevick-Muraca EM. Translation of near-infrared fluorescence imaging technologies: emerging clinical applications. Annu Rev Med 2012;63:217-31. [PubMed]
- Gao X, Cui Y, Levenson RM, et al. In vivo cancer targeting and imaging with semiconductor quantum dots. Nat Biotechnol 2004;22:969-76. [PubMed]
- Hintersteiner M, Enz A, Frey P, et al. In vivo detection of amyloid-beta deposits by near-infrared imaging using an oxazine-derivative probe. Nat Biotechnol 2005;23:577-83. [PubMed]
- Humblet V, Lapidus R, Williams LR, et al. High-affinity near-infrared fluorescent small-molecule contrast agents for in vivo imaging of prostate-specific membrane antigen. Mol Imaging 2005;4:448-62. [PubMed]
- Wu X, Liu H, Liu J, et al. Immunofluorescent labeling of cancer marker Her2 and other cellular targets with semiconductor quantum dots. Nat Biotechnol 2003;21:41-6. [PubMed]
- Edwards PA. Heterogeneous expression of cell-surface antigens in normal epithelia and their tumours, revealed by monoclonal antibodies. Br J Cancer 1985;51:149-60. [PubMed]
- Heppner GH, Miller FR. The cellular basis of tumor progression. Int Rev Cytol 1998;177:1-56. [PubMed]
- Rao J, Dragulescu-Andrasi A, Yao H. Fluorescence imaging in vivo: recent advances. Curr Opin Biotechnol 2007;18:17-25. [PubMed]
- Yang X, Shao C, Wang R, et al. Optical imaging of kidney cancer with novel near infrared heptamethine carbocyanine fluorescent dyes. J Urol 2013;189:702-10. [PubMed]
- Yang X, Shi C, Tong R, et al. Near IR heptamethine cyanine dye-mediated cancer imaging. Clin Cancer Res 2010;16:2833-44. [PubMed]
- Zhu B, Rasmussen JC, Lu Y, et al. Reduction of excitation light leakage to improve near-infrared fluorescence imaging for tissue surface and deep tissue imaging. Med Phys 2010;37:5961-70. [PubMed]
- Crane LM, Themelis G, Buddingh KT, et al. Multispectral real-time fluorescence imaging for intraoperative detection of the sentinel lymph node in gynecologic oncology. J Vis Exp 2010.2225. [PubMed]
- Sevick-Muraca EM, Sharma R, Rasmussen JC, et al. Imaging of lymph flow in breast cancer patients after microdose administration of a near-infrared fluorophore: feasibility study. Radiology 2008;246:734-41. [PubMed]
- Kaibori M, Ishizaki M, Matsui K, et al. Intraoperative indocyanine green fluorescent imaging for prevention of bile leakage after hepatic resection. Surgery 2011;150:91-8. [PubMed]
- Chen SF, Kato Y, Oda J, et al. The application of intraoperative near-infrared indocyanine green videoangiography and analysis of fluorescence intensity in cerebrovascular surgery. Surg Neurol Int 2011;2:42. [PubMed]
- The MICAD Research Team. Bombesin peptide conjugated–cross-linked iron oxide-Cy5.5.
- Marshall MV, Draney D, Sevick-Muraca EM, et al. Single-dose intravenous toxicity study of IRDye 800CW in Sprague-Dawley rats. Mol Imaging Biol 2010;12:583-94. [PubMed]
- Lilja H, Ulmert D, Vickers AJ. Prostate-specific antigen and prostate cancer: prediction, detection and monitoring. Nat Rev Cancer 2008;8:268-78. [PubMed]
- Tsao CK, Galsky MD, Small AC, et al. Targeting the androgen receptor signalling axis in castration-resistant prostate cancer (CRPC). BJU Int 2012;110:1580-8. [PubMed]
- Lilja H. A kallikrein-like serine protease in prostatic fluid cleaves the predominant seminal vesicle protein. J Clin Invest 1985;76:1899-903. [PubMed]
- Christensson A, Laurell CB, Lilja H. Enzymatic activity of prostate-specific antigen and its reactions with extracellular serine proteinase inhibitors. Eur J Biochem 1990;194:755-63. [PubMed]
- Ulmert D, Evans MJ, Holland JP, et al. Imaging androgen receptor signaling with a radiotracer targeting free prostate-specific antigen. Cancer Discov 2012;2:320-7. [PubMed]
- Ho G, Morin J, Delaney J, et al. Detection and quantification of enzymatically active prostate-specific antigen in vivo. J Biomed Opt 2013;18:101319. [PubMed]
- Ulmert D, Serio AM, O’Brien MF, et al. Long-term prediction of prostate cancer: prostate-specific antigen (PSA) velocity is predictive but does not improve the predictive accuracy of a single PSA measurement 15 years or more before cancer diagnosis in a large, representative, unscreened population. J Clin Oncol 2008;26:835-41. [PubMed]
- Ulmert D, Cronin AM, Björk T, et al. Prostate-specific antigen at or before age 50 as a predictor of advanced prostate cancer diagnosed up to 25 years later: a case-control study. BMC Med 2008;6:6. [PubMed]
- Bostwick DG, Pacelli A, Blute M, et al. Prostate specific membrane antigen expression in prostatic intraepithelial neoplasia and adenocarcinoma: a study of 184 cases. Cancer 1998;82:2256-61. [PubMed]
- Wright GL Jr, Haley C, Beckett ML, et al. Expression of prostate-specific membrane antigen in normal, benign, and malignant prostate tissues. Urol Oncol 1995;1:18-28. [PubMed]
- Mannweiler S, Amersdorfer P, Trajanoski S, et al. Heterogeneity of prostate-specific membrane antigen (PSMA) expression in prostate carcinoma with distant metastasis. Pathol Oncol Res 2009;15:167-72. [PubMed]
- Nakajima T, Mitsunaga M, Bander NH, et al. Targeted, activatable, in vivo fluorescence imaging of prostate-specific membrane antigen (PSMA) positive tumors using the quenched humanized J591 antibody-indocyanine green (ICG) conjugate. Bioconjug Chem 2011;22:1700-5. [PubMed]
- Liu T, Wu LY, Hopkins MR, et al. A targeted low molecular weight near-infrared fluorescent probe for prostate cancer. Bioorg Med Chem Lett 2010;20:7124-6. [PubMed]
- Macheda ML, Rogers S, Best JD. Molecular and cellular regulation of glucose transporter (GLUT) proteins in cancer. J Cell Physiol 2005;202:654-62. [PubMed]
- Shaw RJ. Glucose metabolism and cancer. Curr Opin Cell Biol 2006;18:598-608. [PubMed]
- Calvo MB, Figueroa A, Pulido EG, et al. Potential role of sugar transporters in cancer and their relationship with anticancer therapy. Int J Endocrinol 2010;2010. pii: 205357.
- Gambhir SS. Molecular imaging of cancer with positron emission tomography. Nat Rev Cancer 2002;2:683-93. [PubMed]
- Pysz MA, Gambhir SS, Willmann JK. Molecular imaging: current status and emerging strategies. Clin Radiol 2010;65:500-16. [PubMed]
- Korotcov AV, Ye Y, Chen Y, et al. Glucosamine-linked near-infrared fluorescent probes for imaging of solid tumor xenografts. Mol Imaging Biol 2012;14:443-51. [PubMed]
- Uldry M, Ibberson M, Hosokawa M, et al. GLUT2 is a high affinity glucosamine transporter. FEBS Lett 2002;524:199-203. [PubMed]
- Briganti A, Larcher A, Abdollah F, et al. Updated nomogram predicting lymph node invasion in patients with prostate cancer undergoing extended pelvic lymph node dissection: the essential importance of percentage of positive cores. Eur Urol 2012;61:480-7. [PubMed]
- Heesakkers RA, Jager GJ, Hövels AM, et al. Prostate cancer: detection of lymph node metastases outside the routine surgical area with ferumoxtran-10-enhanced MR imaging. Radiology 2009;251:408-14. [PubMed]
- Heidenreich A, Varga Z, Von Knobloch R. Extended pelvic lymphadenectomy in patients undergoing radical prostatectomy: high incidence of lymph node metastasis. J Urol 2002;167:1681-6. [PubMed]
- Tammela T, Alitalo K. Lymphangiogenesis: Molecular mechanisms and future promise. Cell 2010;140:460-76. [PubMed]
- van der Poel HG, Buckle T, et al. Intraoperative laparoscopic fluorescence guidance to the sentinel lymph node in prostate cancer patients: clinical proof of concept of an integrated functional imaging approach using a multimodal tracer. Eur Urol 2011;60:826-33. [PubMed]
- Hall MA, Kwon S, Robinson H, et al. Imaging prostate cancer lymph node metastases with a multimodality contrast agent. Prostate 2012;72:129-46. [PubMed]
- Cai QY, Yu P, Besch-Williford C, et al. Near-infrared fluorescence imaging of gastrin releasing peptide receptor targeting in prostate cancer lymph node metastases. Prostate 2013;73:842-54. [PubMed]
- de Visser M, van Weerden WM, de Ridder CM, et al. Androgen-dependent expression of the gastrin-releasing peptide receptor in human prostate tumor xenografts. J Nucl Med 2007;48:88-93. [PubMed]
- Markwalder R, Reubi JC. Gastrin-releasing peptide receptors in the human prostate: relation to neoplastic transformation. Cancer Res 1999;59:1152-9. [PubMed]
- Shi C, Zhu Y, Xie Z, et al. Visualizing human prostate cancer cells in mouse skeleton using bioconjugated near-infrared fluorescent quantum dots. Urology 2009;74:446-51. [PubMed]
- Zaheer A, Cho SY, Pomper MG. New agents and techniques for imaging prostate cancer. J Nucl Med 2009;50:1387-90. [PubMed]
- Hu P, Chu GC, Zhu G, et al. Multiplexed quantum dot labeling of activated c-Met signaling in castration-resistant human prostate cancer. PLoS One 2011;6:e28670. [PubMed]
- Ghosh SC, Ghosh P, Wilganowski N, et al. Multimodal chelation platform for near-infrared fluorescence/nuclear imaging. J Med Chem 2013;56:406-16. [PubMed]
- Benko G, Spajić B, Krušlin B, et al. Impact of the EpCAM expression on biochemical recurrence-free survival in clinically localized prostate cancer. Urol Oncol 2013;31:468-74. [PubMed]
- Mukherjee S, Richardson AM, Rodriguez-Canales J, et al. Identification of EpCAM as a molecular target of prostate cancer stroma. Am J Pathol 2009;175:2277-87. [PubMed]
- Dodelet VC, Pasquale EB. Eph receptors and ephrin ligands: embryogenesis to tumorigenesis. Oncogene 2000;19:5614-9. [PubMed]
- Nakamoto M, Bergemann AD. Diverse roles for the Eph family of receptor tyrosine kinases in carcinogenesis. Microsc Res Tech 2002;59:58-67. [PubMed]
- Lee YC, Perren JR, Douglas EL, et al. Investigation of the expression of the EphB4 receptor tyrosine kinase in prostate carcinoma. BMC Cancer 2005;5:119. [PubMed]
- Zhang R, Xiong C, Huang M, et al. Peptide-conjugated polymeric micellar nanoparticles for Dual SPECT and optical imaging of EphB4 receptors in prostate cancer xenografts. Biomaterials 2011;32:5872-9. [PubMed]
- Kosaka N, Mitsunaga M, Longmire MR, et al. Near infrared fluorescence-guided real-time endoscopic detection of peritoneal ovarian cancer nodules using intravenously injected indocyanine green. Int J Cancer 2011;129:1671-7. [PubMed]
- Obaidat A, Roth M, Hagenbuch B. The expression and function of organic anion transporting polypeptides in normal tissues and in cancer. Annu Rev Pharmacol Toxicol 2012;52:135-51. [PubMed]
- Pressler H, Sissung TM, Venzon D, et al. Expression of OATP family members in hormone-related cancers: potential markers of progression. PLoS One 2011;6:e20372. [PubMed]
- Fujimoto N, Kubo T, Inatomi H, et al. Polymorphisms of the androgen transporting gene SLCO2B1 may influence the castration resistance of prostate cancer and the racial differences in response to androgen deprivation. Prostate Cancer Prostatic Dis 2013;16:336-40. [PubMed]
- Yang M, Xie W, Mostaghel E, et al. SLCO2B1 and SLCO1B3 may determine time to progression for patients receiving androgen deprivation therapy for prostate cancer. J Clin Oncol 2011;29:2565-73. [PubMed]
- Sharifi N, Hamada A, Sissung T, et al. A polymorphism in a transporter of testosterone is a determinant of androgen independence in prostate cancer. BJU Int 2008;102:617-21. [PubMed]
- Hamada A, Sissung T, Price DK, et al. Effect of SLCO1B3 haplotype on testosterone transport and clinical outcome in caucasian patients with androgen-independent prostatic cancer. Clin Cancer Res 2008;14:3312-8. [PubMed]
- Wright JL, Kwon EM, Ostrander EA, et al. Expression of SLCO transport genes in castration-resistant prostate cancer and impact of genetic variation in SLCO1B3 and SLCO2B1 on prostate cancer outcomes. Cancer Epidemiol Biomarkers Prev 2011;20:619-27. [PubMed]
- Pressler HM, Figg WD. Androgen synthesis and steroid transporters in prostate cancer: rethinking the transition to CRPC. Cancer Biol Ther 2010;9:1043-5. [PubMed]