Novel non-AR therapeutic targets in castrate resistant prostate cancer
Introduction
Since the initial experiences of Charles Huggins treating with advanced prostate cancer, it has been clear that the prostate cancer does not uniformly and completely regress as a result of androgen ablation (1,2). Subsequently, there have been decades of research to identify and characterize pathways and targets which allow prostate cancer to progress independently of androgens. More recently, it has become clearer that the androgen receptor (AR) remains a principal target even in castrate resistant prostate cancer (CRPC). This is highlighted by the clinical success of potent AR antagonists and steroidogenesis inhibitors in men with CRPC (3-5). The continued usefulness of PSA as a prognostic marker in CRPC also highlights how the AR axis remains a principal target (6). However, despite these recent improvements, CRPC remains a lethal disease and the search for new and improved treatments continues.
In recent years, an increased understanding of the molecular biology of CRPC has led to a proliferation of novel targeted therapeutics in clinical evaluation. Table 1 lists some of the non-AR targets which are in clinical evaluation in CRPC. These novel targets emerge as CRPC develops more genetic and epigenetic alterations over time (7). In addition, tumours acquire resistance through alternative pathways as a result of the selective pressure of current treatments. Accordingly, the potent AR-targeting agents abiraterone and enzalutamide will likely lead to cancers that survive and proliferate through activation of alternate pathways.
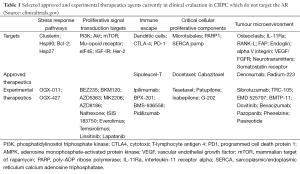
Full table
Along with the excitement of the number of new and emerging non-AR therapeutics in the CRPC clinical space also comes some hesitation as to their eventually utility given the large number of recent disappointing phase III trial results in CRPC. This includes almost all targeted therapies in combination with docetaxel (Table 2). This highlights the difficulties in the generalizability of pre-clinical models and the need for well-designed and planned Phase II and III clinical trials. Further, better selection of patients for these non-AR therapies is another need which may improve the success of new agents in clinical evaluation.
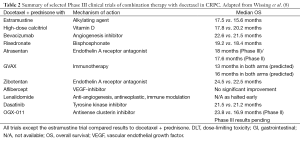
Full table
The molecular classification of CRPC will continue to evolve and is expected to play a large role in selecting patients for future trials of targeted therapy. Most of the molecular targets under investigation are not specific to prostate cancer, with overlap with other advanced cancers. While the AR plays a unique role in prostate cancer, the division of molecular targets in CRPC into AR targets vs. non-AR targets can be misleading. Many non-AR cellular targets appear to support continued activation of the AR-axis through complex signalling and structural pathways. For example, microtubules are considered important in AR cellular transport (9). Similarly, the Akt pathway regulates and is regulated by the AR (10,11). Further characterization of the molecular changes which occur with CRPC progression may allow for identification of predictive and prognostic biomarkers for personalized targeted therapy.
This review will highlight some of the recently approved and currently researched molecular targets in CRPC beyond novel AR antagonists and steroid synthesis inhibitors. These targets, with their corresponding therapeutics, focus on many of the hallmarks of cancer (Figure 1). We will focus on discussing both the biologic mechanisms and clinical experience with these targets in CRPC. Particular emphasis is on agents which have reached Phase II-III clinical trials. With a particular concern that neuroendocrine cancers (also known as small cell cancer of the prostate) will be more prevalence as a result of recent treatment improvements, we will also discuss briefly this relatively rare subtype of CRPC.
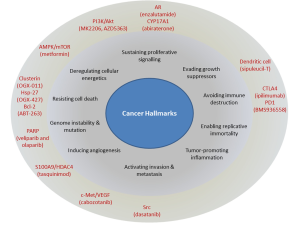
Targeting microtubules
Docetaxel and cabazitaxel are the two taxanes which are in clinical use in CRPC. Taxanes function by stabilizing the dynamic polymerization of microtubules. The ability of microtubules to assemble and disassemble is critical for mitosis and thus targeting microtubules preferentially targets rapidly dividing cancer cells. It also affects AR signalling through its alteration of microtubules-associated AR cellular transport and nuclear translocation (9,12). Docetaxel is the first approved agent outside of hormonal therapy which has a demonstrated survival benefit in CRPC (13,14).
Two studies demonstrated the survival benefit of docetaxel in CRPC patients. The TAX327 study showed that docetaxel every 3 weeks plus daily prednisone was superior to docetaxel every week plus prednison or weekly mitoxantrone plus prednisone (14). Updated survival results indicate a median survival of 19.2 months in the q3weekly docetaxel plus prednisose arm versus 17.8 months in the weekly docetaxel arm plus prednisone versus 16.3 months in the mitoxantrone/prednisone arm (13). The SWOG9916 study also found a 2-month survival benefit of docetaxel plus estramustine compared to mitoxantrone plus prednisone (15). However, no benefit in pain palliation or quality of life was noted; subsequently, estramustine is not in clinical use in CRPC.
Resistance to taxanes may be mediated through overexpression of the multi-drug resistant P-glycoprotein efflux pump (16), mutations in the microtubule binding sites, and mutations in microtubule-associated proteins giving greater stability to cellular microtubule assembly (17,18). Cabizataxel is a newer taxane which was selected through pre-clinical studies which found it had the greatest activity against docetaxel-resistant cell lines in vitro and in vivo (19). However, there is no clear definition of clinical docetaxel resistance. Similarly, the optimal duration of treatment with docetaxel is usually based on physician judgement. In the TAX-327, patients received up to 10 cycles; however it appears that more can be given if patients are receiving a tolerable response. Further, re-challenging patients with docetaxel after the recurrence of CRPC has also demonstrated some clinical success (20,21).
The TROPIC study established the role of cabazitaxel as second line therapy in CRPC after docetaxel. This study randomized men with progressive disease during or after docetaxel to receive cabazitaxel plus prednisone versus mitoxantrone plus prednisone (22). Cabazitaxel improved overall survival by a median of 2.4 months in this second-line setting. Cabazitaxel had a higher rate of adverse effects, particularly myelosupression, though even with mitoxantrone adverse events were higher than prior trials (14,15), highlighting the selection of sicker patients in this trial. Side effects of neutropenia and diarrhea were common (82% versus 58% and 6% versus <1% cabazitaxel versus mitoxantrone). Significantly, 28 patients (8%) in the cabazitaxel group had febrile neutropenia during the study versus 5 (1%) in the mitoxantrone arm. Two phase III trials are ongoing: FIRSTANA assesses cabazitaxel prior to docetaxel, while PROSELICA evaluates a lower dose (20 versus 25 mg/m2) in men treated with prior docetaxel.
Newer therapeutics targeting the microtubules are in development. In contrast to the parenterally administered docetaxel and cabizataxel, tesetaxel is a novel, orally available taxane (Tesetaxel in Chemotherapy-naive Patients with Progressive, Castration-resistant Prostate Cancer http://clinicaltrial.gov/ct2/show/NCT01296243).It is currently in Phase II trials of men with CRPC. Epothilones also target microtubules through a different mechanism of action. Patupilone in a Phase II study recently demonstrated antitumor activity and safety as second line therapy (23). The oral synthetic epothilone, ixabepilone, demonstrated better activity in chemo naïve patients (24-26) compared to use as second line therapy, but has not been advanced to phase III trials.
Targeting the immune response
The goal of immunotherapy is to boost the tumour suppressive response of the patient’s own immune system. This approach has been validated in CPRC with the approval of sipuleucel-T. Phase III randomized trials demonstrate an overall survival benefit (27) in men with minimally or asymptomatic metastatic prostate cancer, though an effect on progression-free survival or PSA-response was not seen. The treatment consists of re-infusing patient’s autologous peripheral blood monocytes and antigen-presenting cells which have been exposed ex vivo to the fusion protein of prostatic acid phosphatase and granulocyte-macrophage colony-stimulating factor (GM-CSF).
The approach of priming of the immune system to tumour antigens has several advantages. It may result in a durable decreased rate of tumour growth. While this has yet to be identified, this theory corroborates with data suggesting that immunotherapy is of greatest benefit in patients with a lower burden of disease (28). The benefit of immunotherapy in lower volume disease is not unique to prostate cancer (29). The comparison of concurrent or sequential treatment of sipuleucel-T with abiraterone in patient with CRPC is underway and may help further understand the sequencing of immunotherapy with other available treatments. Further, in the case of sipuleucel-T, the side effects of this therapy are very tolerable, limited mostly to flu-like symptoms. However, the cost of this treatment remains a challenge to its implementation in many jurisdictions.
The PROSTVAC-VF vaccine similarly aims to boost natural immunity against tumor cells in CRPC. The vaccine consists of transgenes for PSA, as well as three co-stimulatory molecules (B7.1, leukocyte function-associated antigen-3 (LFA-3), and intercellular adhesion molecule-1 (ICAM-1) to enhance immune memory against the weakly immunogenic PSA antigen (30). A priming injection is followed by monthly booster injections. An ongoing Phase III placebo-controlled study evaluates the efficacy of this vaccine +/- GM-CSF on the overall survival of men with minimally symptomatic metastatic prostate cancer. A whole cell vaccine, GVAX, has had two phase III trials terminated early due to an absence of benefit and an increased incidence of deaths in the treatment arm.
Checkpoint modulators of the immune system aim to remove the negative feedback signals in the patient’s own immune system, thereby decreasing the immune system’s tolerance of tumour antigens. Both ipilimumab and programmed death-(PD-1) inhibitors employ this strategy. Ipilimumab has previously demonstrated success in treating metastatic melanoma (31) It is a fully human monoclonal antibody which targets CTLA-4. CTLA-4 is an important negative regulatory receptor on T-cells. By blocking CTLA-4, ipilimumab releases the homeostatic negative feedback on T-regulatory cells which the immune system normally establishes in order to avoid autoimmunity. As a result, the immune system’s tolerance of tumour antigens should decrease, resulting in greater immune-mediated destruction of tumour cells. Two randomized trials comparing ipilimumab versus placebo prior to and after chemotherapy are underway in CRPC. Further, there has also been reported some clinical success using an anti-PD-1 monoclonal antibody in refractory solid tumours (32). PD-1 is an immune inhibitory receptor expressed on T cells which also modulates the immune response. Blocking this receptor reduces some of the negative self-regulation of the immune system, conceptually similar to CTLA-4 inhibitors. In the first large clinical trial of solid tumours, treatment responses were observed in patients with lung, melanoma and renal cell carcinoma, many of whom had failed multiple therapies (32). In the small subset of 17 patients with CRPC, no objective responses were seen, though clinical evaluation in CRPC is ongoing (32). It is possible this target will have fewer side effects than targeting CTLA-4 as a result of a greater specificity for the tumour microenvironment.
Targeting angiogenesis and c-Met
Several trials evaluating anti-angiogenic agents in CRPC have reported universally disappointing results, including aflibercept, bevacizumab, lenalidomide, sunitinib and sorafenib in combination with docetaxel or as second-line monotherapy. Anti-angiogenic inhibitors currently in phase III evaluation include tasquinimod and cabozantinib. Other novel angiogenesis inhibitors currently in Phase II trials include TRC-105, which is a monoclonal antibody against endoglin, a receptor overexpressed on proliferating endothelium and the vascular epithelial growth factor(VEGF)/fibroblast growth factor receptor(FGFR) inhibitor dovitinib (30).
Tasquinimod is a novel orally administered quinoline-3-carboxamide with anti-angiogenic and anti-tumorigenic effects which is now in Phase III trials. In contrast to other angiogenesis inhibitors targeting VEGF and/or tyrosine kinases receptors, tasquinimod disrupts cross talk within the tumour microenvironment by modulating HDAC4 and also targets S100A9 (33). Phase II results demonstrated in men with minimally symptomatic metastatic disease a median PFS of 7.6 compared to 3.3 months in the placebo arm (P=0.0042) (34).
Cabozantinib (XL184) is a dual c-MET and VEGF-receptor inhibitor. c-MET is a receptor tyrosine kinase which binds hepatocyte growth factor. When activated through phosphorylation, c-MET activates downstream signalling pathways involved in survival, growth and invasion. These downstream pathways include the phosphatidylinositol triphosphate (PI3K)/Akt pathway and the mitogen activated protein kinase (MAPK) pathway (Figure 2). In reported Phase II clinical trial results with cabozantinib, 68% of patients at 12 weeks experienced an improvement on bone scan, with 12% having complete remission (36). Further, the median profession-free survival of those on treatment was 23.9 versus 5.9 weeks for placebo. However, randomization was halted early in the trial due to the benefit seen, so the numbers were small (36). Tivantinib is another c-MET inhibitor which in is in Phase II clinical evaluation in CRPC (NCT01519414).
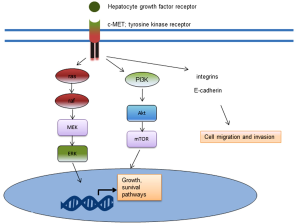
Targeting cellular energetics
Metformin has been demonstrated in several retrospective and large cohort studies to confer an overall survival benefit in men with prostate cancer (37,38), though the literature is not entirely consistent (39,40). The survival benefit appears particular to metformin, as no benefit was seen with other anti-diabetic medications (37). The relative safety, availability and cost of metformin make it an appealing agent for further investigation in CRPC.
Metformin functions as an adenosine monophosphate-activated protein kinase (AMPK) activator. Activated AMPK regulates cellular energetics through inactivation of enzymes involved in adenosine triphosphate consumption such as fatty acid and protein synthesis. It also functions through negative regulation on the mammalian target of rapamycin (mTOR) pathway (37). Clinical studies evaluating metformin alone and in combination with docetaxel for CRPC are now underway (NCT01796028, NCT01215032).
Targeting the stress-response
Molecular chaperones have an important role in the cellular stress response through maintaining protein homeostasis and regulating pro-survival networks. Chaperone proteins stabilize intracellular proteins against against misfolding and aggregation during stress, as well as facilitating intracellular and compartmental transport (41). In CRPC, two stress-activated cytoprotective chaperones, clusterin and Hsp27, are targets in ongoing clinical trials.
Clusterin exists in two forms, nuclear clusterin and secretory clusterin. Secretory clusterin (sCLU) functions as a cytoprotective chaperone which is up-regulated in CRPC. sCLU has been demonstrated to play a role in inhibiting endoplasmic reticulum stress, cytosolic protein aggregation and also inhibits mitochondrial apoptosis (42-44). Custirsen (OGX-011) is a second-generation antisense oligonucleotide against the clusterin mRNA. Preclinical studies demonstrate that OGX-011 potently suppresses sCLU levels in vitro and in vivo (45,46). Further, cotargeting of sCLU and AR delays CRPC progression in pre-clinical models models through inhibiting the adaptive stress response and regulating AR stability (47). In a Phase II clinical trial, a survival advantage of 6.9 months for custirsen plus docetaxel and prednisone over docetaxel and prednisone was seen (48). Phase III results of this combination are expected in 2014.
Heat shock protein-27 (Hsp27) is another abundant stress-induced cellular chaperone protein implicated with the AR signalling and treatment resistance (49,50). OGX-427 is a second-generation antisense oligonucleotide against Hsp27 now in phase II trials as second line treatment in metastatic CRPC in combination with abiraterone.
Targeting survival pathways
Deregulation of normal cellular functions of apoptosis is one of the common characteristics of cancer. Bcl-2 is a regulator of apoptosis; several other anti-apoptotic family members include Bcl-2, Bcl-XL, Mcl-1, BCL-W and BFL-1 (51). The balance of cell survival or cell death is further regulated by multidomain pro-apoptotic proteins such as BAX, BAK and BOK. As in several advanced cancers, Bcl-2 gene expression it is up-regulated in CRPC (52), presenting a targetable oncogene.
A small phase II trial of 13-cis retinoic acid and interferon-alpha2b in combination with paclitaxel demonstrated the ability to modulate Bcl-2 levels in peripheral monocytes. However, the low treatment response rates and decreased quality of life halted further development. Similarly, the use of a Bcl-2 anti-sense oligonucleotide showed promising data in vitro (53,54) and demonstrated proof-of principle target inhibition in phase I trials (55). However, challenges with a short half-life upon infusion contributed to sub-optimal target inhibition and disappointing phase II results (54,56).
BH3 mimetics target the function of Bcl-2 family proteins through hydrophobic binding which displaces the BH3-only proteins, allowing them to activate Bax or Bak proteins and subsequently signal cell death (51). Examples of BH3 mimetics include ABT-737 and its oral-derived enantiomer ABT-263. A clinical trial aims to compared ABT263 plus abiraterone to treatment with ABT263 plus abiraterone and an autophagy inhibitor hydroxychloroquine (NCT01828476).
Targeting DNA-damage repair
PARP inhibitors represent a different class of therapeutics and include veliparib and olaparib. The enzyme poly-ADP ribose polymerase (PARP) is responsible for repairing single strand breaks in DNA. Inhibition of this enzyme leads to alterations in the ability of DNA replication to occur, causing cell death (57). It may have a specific benefit in tumours with BRCA1 or BRCA2 mutations, both of which are implicated in more aggressive prostate cancer (58). BRCA1 and BRCA2 proteins are responsible for repairing double-strand DNA breaks in DNA. With the inhibition of PARP, single-strand breaks may become non-repairable (and thus lethal) double-strand breaks in BRCA1/2 mutant cancers. Similarly, a synthetic lethality using DNA-damage repair inhibitors has also been proposed to apply to the common PTEN-deletion CRPC tumours, which are reported to have defects in homologous recombination (59). Therefore, this represents a possible tailored therapy for patients with these mutations. A clinical trial is also underway for patients with ETS fusions, based on pre-clinical data suggesting the involvement of TMPRSS2 gene fusions with DNA repair cellular machinery, including PARP1 and topoisomerase II (60).
Targeting the tumour microenvironment
With increased understanding the importance of the tumour microenvironment on the progression of CRPC, therapeutic strategies are emerging to target the adjacent stroma (61,62). Further, it appears that treatments which target both the stroma and epithelium compartments may be expected to be more successful. Both androgen androgen deprivation and cabozantinib are examples of this approach: the AR and c-MET are both active in both the stroma and epithelial compartments during CRPC (63,64). IGF-IR inhibitors also target both stroma and tumour components (65). However, with the failure to date of several angiogenesis inhibitors in CRPC, agents targeting the microenvironment are likely best evaluated in rationale combination strategies with other treatments. For example, pre-clinical research suggests that IGF-IR blockade may enhance Src inhibition (66).
Hedgehog signalling is an important paracrine factor during organogenesis and appears to be de-regulated during prostate cancer progression. Sonic hedgehog secreted by the tumour appears to alter the tumour microenvironment to ultimately increase oncogenic Gli-1/2 transcription factors through paracrine signalling. Sonic hedgehog ligands signal via Patched-1 and result in the loss of the Smoothened repression on Gli-1 and Gli-2. Hedgehog signalling appears to be up-regulated following androgen deprivation conditions (67,68). Preclinical data on TAK-441 and GDC-0449 (vismodegib) in CRPC models (68,69) and an ongoing neo-adjuvant study of GDC-0449 should lead to upcoming clinical trials in CRPC patients.
Another novel drug which targets the tumour microenvironment is the monoclonal antibody sibrotuzumab. It targets fibroblast-activated protein (FAP). This protein expressed in cancer-associated stroma, but not normal stroma-associated with epithelial cancers. It is considered to play a role in tumor growth and proliferation (70).
Targeting the bone micro-environment
Therapeutic targeting of the bone microenvironment addresses side effects associated with androgen deprivation therapy as well as the commonest metastatic location of CRPC. Several clinical trials have now established new treatment options for patients with CRPC and should be used appropriately alongside lifestyle changes and calcium supplementation. Bisphosphonates were the first agents approved for men with metastatic prostate cancer. Zoledronic acid was approved based on studies which demonstrated an improvement in skeletal related events, though no survival benefit was observed (71,72). More recently, denosumab has been approved has been approved for me with CRPC. It functions as a monoclonal antibody against RANK-L, which prevents bone loss through the inactivation of osteoclasts. Further, denosumab also appears to have an effect on the metastatic niche, with a delay in the appearance of bone metastasis (73). However, this did not result in differences in overall survival. Compared to zoledronic acid, denosumab appears to have superior potency, with a greater reduction in skeletal-related events (74).
Radiopharmaceuticals also target the bone metastatic environment. Historical agents Rhenium-186 and Samarium-135 have demonstrated improved bone pain in patients with metastatic CRPC in small randomized trials (75,76). Strontium-89 is another radiopharmaceutical which as a calcium mimetic has a strong propensity for the bone microenvironment (77,78). An ongoing phase III trial evaluates Sr89 plus docetaxel and prednisolone versus docetaxel and prednisolone. Radium-223 chloride is a newer calcium mimetic radiopharmaceutical. In contrast to the aforementioned agents which emit beta-radiation, it emits alpha radiation. Alpha-radiation has a shorter penetration depth with higher energy and is therefore less toxic to the bone marrow. Bone marrow toxicity is a challenging toxicity in men with CRPC and bone metastasis who often have anemia to begin with. Clinical experience has demonstrated now significant differences in hematologic side effects using radium-223. Notably, radium-223 has also demonstrated an improvement in overall survival in recent clinical trials of men with painful bone metastasis (79,80). In a placebo-controlled trials, the radium-223 treated arm had a hazard ratio of 0.70 for overall survival at the interim analysis (81). As well as validating the benefit of this drug, these studies suggest that a survival benefit may be achieved through targeting of metastatic disease.
Targeting intra-cellular signalling transduction pathways
Further understanding of the molecular biology of cancer has led to several intracellular transduction pathway inhibitors now in clinical evaluation. Often, these agents are evaluated in combination with current treatment strategies to synergize anti-cancer activity and minimize toxicity. For example, studies suggest a synergist effect of targeting of both AR and signal transduction pathways such as PI3K/Akt and MAPK pathways (11,82).
The phosphatidylinositol 3-kinase (PI3K)/AKT/mTOR pathway represents the most commonly activated pathway in CRPC. Alterations in this pathway have been identified in 42% of primary tumours and 100% of metastases (83). This pathway is also active in many other advanced cancers (84). Loss of function of the PTEN repressor results in increased levels of activated Akt and downstream effects, as does an activating mutation of the PIK3A gene. The activated downstream effectors, including GSK3β and S6 kinase, result in cell survival, proliferation, migration and invasion (Figure 3) (84-87). Activation of the PI3K/Akt pathway is associated with higher Gleason score, decreased metastasis free survival (87). Due to the reciprocal interactions of this pathway, combination targeting strategies with AR antagonists are under investigation using novel therapeutics targeting nodes of this pathway (11,88).
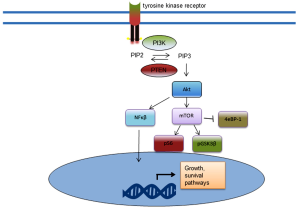
The Src family nonreceptor tyrosine kinases are another intra-cellular target of interest in CRPC. There are nine members of this kinase group (Blk, Fgr, Fyn, Hck, Lck, Lyn, Src, Yes, and Yrk) (89). Src is the most studied as it relates to prostate cancer progression. Src and related kinases are associated with prostate cancer progression through various mechanisms, including proliferation, invasion and interactions with the AR (90-92). Src signalling is also involved in regulating bone turnover in prostate cancer (93) and may therefore be important in the progression of bone metastasis, a common event in CRPC.
Dasatinib and saracatinib (AZD0530) are two Src inhibitors which have completed phase II trials in CRPC. Dasatinib is a small-molecule multi-tyrosine kinase inhibitor of with broad activity against receptor tyrosine kinases, Src family kinases, c-Kit, PDGFR, Bcr-Abl and ephrins (94). In a Phase I/II trial of dasatinib plus docetaxel, 30% of patients had disappearance of lesions on bone scan and 57% of patients experienced a durable PSA response (95). In Phase II dasatinib monotherapy trials in men prior to chemotherapy, no responses were seen but there was a lack of progression in 43% of patients at 12 weeks (96). Results of the phase III READY trial of dasatinib in addition to standard docetaxel in metastatic CRPC presented at GU-ASCO 2013 showed no benefit to OS (97). Dasatinib is currently in Phase III trials in combination with abiraterone.
Targeting neuroendocrine prostate cancer
Neuroendocrine prostate cancer is a separate entity from most cases of CPRC. Clinically, it remains a relatively rare and thoroughly aggressive phenotype. Disease progression appears entirely unrelated to the AR axis with patients usually identified through a disproportionately low PSA. Visceral and brain metastases are more common. Serum and tissue markers of chromogranin A, NSE and synaptophysin are commonly elevated. Recently, protocadherin-PC has also been suggested to be a marker of neuroendocrine transdifferentiation (98).
There is a renewed interest in this subtype of CRPC for a couple reasons. Firstly, the increasing use of potent AR antagonism is postulated to increase the incidence of NEPC, though this requires further research to validate this hypothesis. Secondly, new treatments for this aggressive entity are now in development. Aurora kinase inhibitors such as MLN8237 target neuroendocrine/small cell prostate cancer. Sequencing studies have identified overexpression and gene amplification of aurora kinase A and N-myc in 40% of NEPC vs. 5% of prostate cancers (99). Phase II trials of MLN8237 are ongoing in men with elevated NEPC markers.
Conclusions
An improved understanding of the molecular biology of CRPC has led the way to a relative explosion in the number of targets and novel treatments for this lethal disease. Many new targets beyond the AR have the potential to further improve the outcomes for patients. Past failures of non-AR agents in clinical trials highlight the need for rigorous evaluation of agents which are selected to proceed to Phase III clinical evaluation. Combination strategies will likely optimize the efficacy of targeting alternate pathways. Further, the molecular classification of tumour subtypes will further aid in patient selection for these targeted therapies.
Acknowledgements
None.
Footnote
Conflicts of Interest: The University of British Columbia has submitted patent applications, listing Dr. Gleave as inventor, on the antisense sequence described in this paper. This IP has been licensed to OncoGenex Pharmaceuticals, a Vancouver-based biotechnology company that Dr. Gleave has founding shares in. The other author has no conflicts of interest to declare.
References
- Huggins C, Hodges CV. Studies on prostatic cancer: I. The effect of castration, of estrogen and of androgen injection on serum phosphatases in metastatic carcinoma of the prostate. 1941. J Urol 2002;168:9-12. [PubMed]
- Denmeade SR, Isaacs JT. A history of prostate cancer treatment. Nat Rev Cancer 2002;2:389-96. [PubMed]
- Scher HI, Fizazi K, Saad F, et al. Increased survival with enzalutamide in prostate cancer after chemotherapy. N Engl J Med 2012;367:1187-97. [PubMed]
- Ryan CJ, Smith MR, de Bono JS, et al. Abiraterone in metastatic prostate cancer without previous chemotherapy. N Engl J Med 2013;368:138-48. [PubMed]
- de Bono JS, Logothetis CJ, Molina A, et al. Abiraterone and increased survival in metastatic prostate cancer. N Engl J Med 2011;364:1995-2005. [PubMed]
- Smith MR, Cook R, Lee KA, et al. Disease and host characteristics as predictors of time to first bone metastasis and death in men with progressive castration-resistant nonmetastatic prostate cancer. Cancer 2011;117:2077-85. [PubMed]
- Huang J, Wang JK, Sun Y. Molecular pathology of prostate cancer revealed by next-generation sequencing: opportunities for genome-based personalized therapy. Curr Opin Urol 2013;23:189-93. [PubMed]
- Wissing MD, van Diest PJ, van der Wall E, et al. Antimitotic agents for the treatment of patients with metastatic castrate-resistant prostate cancer. Expert Opin Investig Drugs 2013;22:635-61. [PubMed]
- Gan L, Chen S, Wang Y, et al. Inhibition of the androgen receptor as a novel mechanism of taxol chemotherapy in prostate cancer. Cancer Res 2009;69:8386-94. [PubMed]
- Wang Y, Kreisberg JI, Ghosh PM. Cross-talk between the androgen receptor and the phosphatidylinositol 3-kinase/Akt pathway in prostate cancer. Curr Cancer Drug Targets 2007;7:591-604. [PubMed]
- Carver BS, Chapinski C, Wongvipat J, et al. Reciprocal feedback regulation of PI3K and androgen receptor signaling in PTEN-deficient prostate cancer. Cancer Cell 2011;19:575-86. [PubMed]
- Zhu ML, Horbinski CM, Garzotto M, et al. Tubulin-targeting chemotherapy impairs androgen receptor activity in prostate cancer. Cancer Res 2010;70:7992-8002. [PubMed]
- Berthold DR, Pond GR, Soban F, et al. Docetaxel plus prednisone or mitoxantrone plus prednisone for advanced prostate cancer: updated survival in the TAX 327 study. J Clin Oncol 2008;26:242-5. [PubMed]
- Tannock IF, de Wit R, Berry WR, et al. Docetaxel plus prednisone or mitoxantrone plus prednisone for advanced prostate cancer. N Engl J Med 2004;351:1502-12. [PubMed]
- Petrylak DP, Tangen CM, Hussain MH, et al. Docetaxel and estramustine compared with mitoxantrone and prednisone for advanced refractory prostate cancer. N Engl J Med 2004;351:1513-20. [PubMed]
- Greenberger LM, Lothstein L, Williams SS, et al. Distinct P-glycoprotein precursors are overproduced in independently isolated drug-resistant cell lines. Proc Natl Acad Sci U S A 1988;85:3762-6. [PubMed]
- Hari M, Wang Y, Veeraraghavan S, et al. Mutations in alpha- and beta-tubulin that stabilize microtubules and confer resistance to colcemid and vinblastine. Mol Cancer Ther 2003;2:597-605. [PubMed]
- Wilson L, Jordan MA. New microtubule/tubulin-targeted anticancer drugs and novel chemotherapeutic strategies. J Chemother 2004;16 Suppl 4:83-5. [PubMed]
- Michielsen DP, Braeckman JG, Denis L. Cabazitaxel for the treatment of prostate cancer. Expert Opin Pharmacother 2011;12:977-82. [PubMed]
- Caffo O, Pappagallo G, Brugnara S, et al. Multiple rechallenges for castration-resistant prostate cancer patients responding to first-line docetaxel: assessment of clinical outcomes and predictive factors. Urology 2012;79:644-9. [PubMed]
- Loriot Y, Massard C, Gross-Goupil M, et al. The interval from the last cycle of docetaxel-based chemotherapy to progression is associated with the efficacy of subsequent docetaxel in patients with prostate cancer. Eur J Cancer 2010;46:1770-2. [PubMed]
- de Bono JS, Oudard S, Ozguroglu M, et al. Prednisone plus cabazitaxel or mitoxantrone for metastatic castration-resistant prostate cancer progressing after docetaxel treatment: a randomised open-label trial. Lancet 2010;376:1147-54. [PubMed]
- Chi KN, Beardsley E, Eigl BJ, et al. A phase 2 study of patupilone in patients with metastatic castration-resistant prostate cancer previously treated with docetaxel: Canadian Urologic Oncology Group study P07a. Ann Oncol 2012;23:53-8. [PubMed]
- Rosenberg JE, Galsky MD, Rohs NC, et al. A retrospective evaluation of second-line chemotherapy response in hormone-refractory prostate carcinoma: second line taxane-based therapy after first-line epothilone-B analog ixabepilone (BMS-247550) therapy. Cancer 2006;106:58-62. [PubMed]
- Hussain M, Tangen CM, Lara PN Jr, et al. Ixabepilone (epothilone B analogue BMS-247550) is active in chemotherapy-naive patients with hormone-refractory prostate cancer: a Southwest Oncology Group trial S0111. J Clin Oncol 2005;23:8724-9. [PubMed]
- Galsky MD, Small EJ, Oh WK, et al. Multi-institutional randomized phase II trial of the epothilone B analog ixabepilone (BMS-247550) with or without estramustine phosphate in patients with progressive castrate metastatic prostate cancer. J Clin Oncol 2005;23:1439-46. [PubMed]
- Kantoff PW, Higano CS, Shore ND, et al. Sipuleucel-T immunotherapy for castration-resistant prostate cancer. N Engl J Med 2010;363:411-22. [PubMed]
- Joniau S, Abrahamsson PA, Bellmunt J, et al. Current vaccination strategies for prostate cancer. Eur Urol 2012;61:290-306. [PubMed]
- Gulley JL, Madan RA, Schlom J. Impact of tumour volume on the potential efficacy of therapeutic vaccines. Curr Oncol 2011;18:e150-7. [PubMed]
- TRACON Pharmaceuticals Announces Dosing of Initial Three Cancer Patients in a Phase 1 Clinical Trial with TRC105, a Human Chimeric Antibody. Available online: http://www.traconpharma.com/pr_01_9_08.php
- Tarhini A, Lo E, Minor DR. Releasing the brake on the immune system: ipilimumab in melanoma and other tumors. Cancer Biother Radiopharm 2010;25:601-13. [PubMed]
- Topalian SL, Hodi FS, Brahmer JR, et al. Safety, activity, and immune correlates of anti-PD-1 antibody in cancer. N Engl J Med 2012;366:2443-54. [PubMed]
- Isaacs JT, Antony L, Dalrymple SL, et al. Tasquinimod Is an Allosteric Modulator of HDAC4 survival signaling within the compromised cancer microenvironment. Cancer Res 2013;73:1386-99. [PubMed]
- Pili R, Häggman M, Stadler WM, et al. Phase II randomized, double-blind, placebo-controlled study of tasquinimod in men with minimally symptomatic metastatic castrate-resistant prostate cancer. J Clin Oncol 2011;29:4022-8. [PubMed]
- Loriot Y, Zoubeidi A, Gleave ME. Targeted therapies in metastatic castration-resistant prostate cancer: beyond the androgen receptor. Urol Clin North Am 2012;39:517-31. [PubMed]
- Smith DC, Smith MR, Sweeney C, et al. Cabozantinib in patients with advanced prostate cancer: results of a phase II randomized discontinuation trial. J Clin Oncol 2013;31:412-9. [PubMed]
- Margel D, Urbach DR, Lipscombe LL, et al. Metformin Use and All-Cause and Prostate Cancer-Specific Mortality Among Men With Diabetes. J Clin Oncol 2013;31:3069-75. [PubMed]
- Spratt DE, Zhang C, Zumsteg ZS, et al. Metformin and prostate cancer: reduced development of castration-resistant disease and prostate cancer mortality. Eur Urol 2013;63:709-16. [PubMed]
- Wright JL, Stanford JL. Metformin use and prostate cancer in Caucasian men: results from a population-based case-control study. Cancer Causes Control 2009;20:1617-22. [PubMed]
- Murtola TJ, Tammela TL, Lahtela J, et al. Antidiabetic medication and prostate cancer risk: a population-based case-control study. Am J Epidemiol 2008;168:925-31. [PubMed]
- Zoubeidi A, Gleave M. Small heat shock proteins in cancer therapy and prognosis. Int J Biochem Cell Biol 2012;44:1646-56. [PubMed]
- Nizard P, Tetley S, Le Dréan Y, et al. Stress-induced retrotranslocation of clusterin/ApoJ into the cytosol. Traffic 2007;8:554-65. [PubMed]
- Carver JA, Rekas A, Thorn DC, et al. Small heat-shock proteins and clusterin: intra- and extracellular molecular chaperones with a common mechanism of action and function? IUBMB Life 2003;55:661-8. [PubMed]
- Shannan B, Seifert M, Leskov K, et al. Challenge and promise: roles for clusterin in pathogenesis, progression and therapy of cancer. Cell Death Differ 2006;13:12-9. [PubMed]
- Gleave M, Miyake H. Use of antisense oligonucleotides targeting the cytoprotective gene, clusterin, to enhance androgen- and chemo-sensitivity in prostate cancer. World J Urol 2005;23:38-46. [PubMed]
- Sowery RD, Hadaschik BA, So AI, et al. Clusterin knockdown using the antisense oligonucleotide OGX-011 re-sensitizes docetaxel-refractory prostate cancer PC-3 cells to chemotherapy. BJU Int 2008;102:389-97. [PubMed]
- Matsumoto H, Yamamoto Y, Shiota M, et al. Cotargeting androgen receptor and clusterin delays castrate-resistant prostate cancer progression by inhibiting adaptive stress response and AR stability. Cancer Res 2013;73:5206-17. [PubMed]
- Saad F, Hotte S, North S, et al. Randomized phase II trial of Custirsen (OGX-011) in combination with docetaxel or mitoxantrone as second-line therapy in patients with metastatic castrate-resistant prostate cancer progressing after first-line docetaxel: CUOG trial P-06c. Clin Cancer Res 2011;17:5765-73. [PubMed]
- Zoubeidi A, Zardan A, Wiedmann RM, et al. Hsp27 promotes insulin-like growth factor-I survival signaling in prostate cancer via p90Rsk-dependent phosphorylation and inactivation of BAD. Cancer Res 2010;70:2307-17. [PubMed]
- Zoubeidi A, Zardan A, Beraldi E, et al. Cooperative interactions between androgen receptor (AR) and heat-shock protein 27 facilitate AR transcriptional activity. Cancer Res 2007;67:10455-65. [PubMed]
- Ni Chonghaile T, Letai A. Mimicking the BH3 domain to kill cancer cells. Oncogene 2008;27 Suppl 1:S149-57. [PubMed]
- McDonnell TJ, Troncoso P, Brisbay SM, et al. Expression of the protooncogene bcl-2 in the prostate and its association with emergence of androgen-independent prostate cancer. Cancer Res 1992;52:6940-4. [PubMed]
- Benimetskaya L, Miller P, Benimetsky S, et al. Inhibition of potentially anti-apoptotic proteins by antisense protein kinase C-alpha (Isis 3521) and antisense bcl-2 (G3139) phosphorothioate oligodeoxynucleotides: relationship to the decreased viability of T24 bladder and PC3 prostate cancer cells. Mol Pharmacol 2001;60:1296-307. [PubMed]
- Sternberg CN, Dumez H, Van Poppel H, et al. Docetaxel plus oblimersen sodium (Bcl-2 antisense oligonucleotide): an EORTC multicenter, randomized phase II study in patients with castration-resistant prostate cancer. Ann Oncol 2009;20:1264-9. [PubMed]
- Tolcher AW, Kuhn J, Schwartz G, et al. A Phase I pharmacokinetic and biological correlative study of oblimersen sodium (genasense, g3139), an antisense oligonucleotide to the bcl-2 mRNA, and of docetaxel in patients with hormone-refractory prostate cancer. Clin Cancer Res 2004;10:5048-57. [PubMed]
- Tolcher AW, Chi K, Kuhn J, et al. A phase II, pharmacokinetic, and biological correlative study of oblimersen sodium and docetaxel in patients with hormone-refractory prostate cancer. Clin Cancer Res 2005;11:3854-61. [PubMed]
- Murai J, Huang SY, Das BB, et al. Trapping of PARP1 and PARP2 by Clinical PARP Inhibitors. Cancer Res 2012;72:5588-99. [PubMed]
- Gallagher DJ, Gaudet MM, Pal P, et al. Germline BRCA mutations denote a clinicopathologic subset of prostate cancer. Clin Cancer Res 2010;16:2115-21. [PubMed]
- Mendes-Pereira AM, Martin SA, Brough R, et al. Synthetic lethal targeting of PTEN mutant cells with PARP inhibitors. EMBO Mol Med 2009;1:315-22. [PubMed]
- Haffner MC, Aryee MJ, Toubaji A, et al. Androgen-induced TOP2B-mediated double-strand breaks and prostate cancer gene rearrangements. Nat Genet 2010;42:668-75. [PubMed]
- Gonda TA, Varro A, Wang TC, et al. Molecular biology of cancer-associated fibroblasts: can these cells be targeted in anti-cancer therapy? Semin Cell Dev Biol 2010;21:2-10. [PubMed]
- Sluka P, Davis ID. Cell mates: paracrine and stromal targets for prostate cancer therapy. Nat Rev Urol 2013;10:441-51. [PubMed]
- Varkaris A, Corn PG, Gaur S, et al. The role of HGF/c-Met signaling in prostate cancer progression and c-Met inhibitors in clinical trials. Expert Opin Investig Drugs 2011;20:1677-84. [PubMed]
- Cano P, Godoy A, Escamilla R, et al. Stromal-epithelial cell interactions and androgen receptor-coregulator recruitment is altered in the tissue microenvironment of prostate cancer. Cancer Res 2007;67:511-9. [PubMed]
- Kawada M, Inoue H, Masuda T, et al. Insulin-like growth factor I secreted from prostate stromal cells mediates tumor-stromal cell interactions of prostate cancer. Cancer Res 2006;66:4419-25. [PubMed]
- Dayyani F, Parikh NU, Varkaris AS, et al. Combined Inhibition of IGF-1R/IR and Src family kinases enhances antitumor effects in prostate cancer by decreasing activated survival pathways. PLoS One 2012;7:e51189. [PubMed]
- Bragina O, Njunkova N, Sergejeva S, et al. Sonic Hedgehog pathway activity in prostate cancer. Oncol Lett 2010;1:319-25. [PubMed]
- Ibuki N, Ghaffari M, Pandey M, et al. TAK-441, a novel investigational smoothened antagonist, delays castration-resistant progression in prostate cancer by disrupting paracrine hedgehog signaling. Int J Cancer 2013;133:1955-66. [PubMed]
- Karlou M, Lu JF, Wu G, et al. Hedgehog signaling inhibition by the small molecule smoothened inhibitor GDC-0449 in the bone forming prostate cancer xenograft MDA PCa 118b. Prostate 2012;72:1638-47. [PubMed]
- Liu R, Li H, Liu L, et al. Fibroblast activation protein: A potential therapeutic target in cancer. Cancer Biol Ther 2012;13:123-9. [PubMed]
- Saad F, Gleason DM, Murray R, et al. Long-term efficacy of zoledronic acid for the prevention of skeletal complications in patients with metastatic hormone-refractory prostate cancer. J Natl Cancer Inst 2004;96:879-82. [PubMed]
- Ernst DS, Tannock IF, Winquist EW, et al. Randomized, double-blind, controlled trial of mitoxantrone/prednisone and clodronate versus mitoxantrone/prednisone and placebo in patients with hormone-refractory prostate cancer and pain. J Clin Oncol 2003;21:3335-42. [PubMed]
- Smith MR, Saad F, Coleman R, et al. Denosumab and bone-metastasis-free survival in men with castration-resistant prostate cancer: results of a phase 3, randomised, placebo-controlled trial. Lancet 2012;379:39-46. [PubMed]
- Fizazi K, Carducci M, Smith M, et al. Denosumab versus zoledronic acid for treatment of bone metastases in men with castration-resistant prostate cancer: a randomised, double-blind study. Lancet 2011;377:813-22. [PubMed]
- Sartor O, Reid RH, Hoskin PJ, et al. Samarium-153-Lexidronam complex for treatment of painful bone metastases in hormone-refractory prostate cancer. Urology 2004;63:940-5. [PubMed]
- Han SH, de Klerk JM, Tan S, et al. The PLACORHEN study: a double-blind, placebo-controlled, randomized radionuclide study with (186)Re-etidronate in hormone-resistant prostate cancer patients with painful bone metastases. Placebo Controlled Rhenium Study. J Nucl Med 2002;43:1150-6. [PubMed]
- Lewington VJ, McEwan AJ, Ackery DM, et al. A prospective, randomised double-blind crossover study to examine the efficacy of strontium-89 in pain palliation in patients with advanced prostate cancer metastatic to bone. Eur J Cancer 1991;27:954-8. [PubMed]
- Porter AT, McEwan AJ. Strontium-89 as an adjuvant to external beam radiation improves pain relief and delays disease progression in advanced prostate cancer: results of a randomized controlled trial. Semin Oncol 1993;20:38-43. [PubMed]
- Nilsson S, Franzén L, Parker C, et al. Bone-targeted radium-223 in symptomatic, hormone-refractory prostate cancer: a randomised, multicentre, placebo-controlled phase II study. Lancet Oncol 2007;8:587-94. [PubMed]
- Parker CC, Pascoe S, Chodacki A, et al. A randomized, double-blind, dose-finding, multicenter, phase 2 study of radium chloride (Ra 223) in patients with bone metastases and castration-resistant prostate cancer. Eur Urol 2013;63:189-97. [PubMed]
- Parker C, Heinrich D, O’Sullivan JM, et al. Overall survival benefit of radium-223 chloride (Alpharadin) in the treatment of patients with symptomatic bone metastases in castration-resistant prostate cancer (CRPC): a phase III randomized trial (ALSYMPCA). Eur J Cancer 2011;47:3.
- Schayowitz A, Sabnis G, Njar VC, et al. Synergistic effect of a novel antiandrogen, VN/124-1, and signal transduction inhibitors in prostate cancer progression to hormone independence in vitro. Mol Cancer Ther 2008;7:121-32. [PubMed]
- Taylor BS, Schultz N, Hieronymus H, et al. Integrative genomic profiling of human prostate cancer. Cancer Cell 2010;18:11-22. [PubMed]
- McCubrey JA, Steelman LS, Kempf CR, et al. Therapeutic resistance resulting from mutations in Raf/MEK/ERK and PI3K/PTEN/Akt/mTOR signaling pathways. J Cell Physiol 2011;226:2762-81. [PubMed]
- Reid AH, Attard G, Ambroisine L, et al. Molecular characterisation of ERG, ETV1 and PTEN gene loci identifies patients at low and high risk of death from prostate cancer. Br J Cancer 2010;102:678-84. [PubMed]
- Fang J, Ding M, Yang L, et al. PI3K/PTEN/AKT signaling regulates prostate tumor angiogenesis. Cell Signal 2007;19:2487-97. [PubMed]
- Pourmand G, Ziaee AA, Abedi AR, et al. Role of PTEN gene in progression of prostate cancer. Urol J 2007;4:95-100. [PubMed]
- Thomas C, Lamoureux F, Crafter C, et al. Synergistic targeting of PI3K/AKT-pathway and androgen-receptor axis significantly delays castration-resistant prostate cancer progression in vivo. Mol Cancer Ther 2013;12:2342-55. [PubMed]
- Fizazi K. The role of Src in prostate cancer. Ann Oncol 2007;18:1765-73. [PubMed]
- Kung HJ, Evans CP. Oncogenic activation of androgen receptor. Urol Oncol 2009;27:48-52. [PubMed]
- Lee LF, Louie MC, Desai SJ, et al. Interleukin-8 confers androgen-independent growth and migration of LNCaP: differential effects of tyrosine kinases Src and FAK. Oncogene 2004;23:2197-205. [PubMed]
- Cai J, Zhang S, Zheng M, et al. Design, synthesis, and in vitro antiproliferative activity of novel Dasatinib derivatives. Bioorg Med Chem Lett 2012;22:806-10. [PubMed]
- Yang JC, Bai L, Yap S, et al. Effect of the specific Src family kinase inhibitor saracatinib on osteolytic lesions using the PC-3 bone model. Mol Cancer Ther 2010;9:1629-37. [PubMed]
- Gnoni A, Marech I, Silvestris N, et al. Dasatinib: an anti-tumour agent via Src inhibition. Curr Drug Targets 2011;12:563-78. [PubMed]
- Araujo JC, Mathew P, Armstrong AJ, et al. Dasatinib combined with docetaxel for castration-resistant prostate cancer: results from a phase 1-2 study. Cancer 2012;118:63-71. [PubMed]
- Yu EY, Wilding G, Posadas E, et al. Phase II study of dasatinib in patients with metastatic castration-resistant prostate cancer. Clin Cancer Res 2009;15:7421-8. [PubMed]
- Araujo JC, Trudel GC, Saad F, et al. Overall survival (OS) and safety of dasatinib/docetaxel versus docetaxel in patients with metastatic castration-resistant prostate cancer (mCRPC): Results from the randomized phase III READY trial. J Clin Oncol 2013;31:abstr LBA8.
- Terry S, Maillé P, Baaddi H, et al. Cross modulation between the androgen receptor axis and protocadherin-PC in mediating neuroendocrine transdifferentiation and therapeutic resistance of prostate cancer. Neoplasia 2013;15:761-72. [PubMed]
- Beltran H, Rickman DS, Park K, et al. Molecular characterization of neuroendocrine prostate cancer and identification of new drug targets. Cancer Discov 2011;1:487-95. [PubMed]