Multiphoton microscopy: applications in Urology and Andrology
Multiphoton microscopy (MPM)
MPM is an optical imaging technology which utilizes nonlinear excitation as a means of exciting fluorescence (1,2). MPM, first demonstrated over 20 years ago, has proven to be extremely useful in biological imaging and has become the standard for fluorescence microscopy both ex vivo and in vivo imaging (1). MPM has been used in neuroscience, oncology, and immunology because it enables the visualization of various biological processes at the cellular level in vivo. Further, MPM is able to provide real time images of unstained tissue in vivo. Many researchers are also searching for clinical applications of this imaging technique. As the equipment for MPM becomes commercially available and miniaturized it could prove to be a useful tool for clinicians. MPM could provide urologists with another novel technology that can aid in the diagnosis and evaluation.
MPM utilizes two-photon excitation fluorescence, which is based on the theoretical principle from quantum mechanics that two photons of low energy can simultaneously be absorbed by a molecule and cause excitation that would normally be produced by the absorption of one photon of higher energy. If the molecule that has been excited is fluorescent, it will emit a photon just as one would expect from the absorption of a single photon of greater energy. MPM has been termed nonlinear excitation because the simultaneous interaction of two photons with a single molecule has a quadratic dependence on light intensity in contrast to the linear dependence seen in conventional fluorescence (1). In MPM, a laser is used to scan a sample and will produce excitation only at the focal point of the laser where there is enough photon density. The resulting resolution is comparable to conventional confocal microscopy and tissue penetration is even greater than conventional confocal microscopy (2,3).
MPM utilizes two-photon excitation that enables autofluorescence in unstained living tissue through intrinsic tissue emissions. The intrinsic emissions in cells are produced by the cellular autofluorescence of nicotinamide adenine dinucleotide (NADH) and flavin adenine dinucleotide (FAD) within cells as well as elastin and connective tissue. MPM can also utilize higher order scattering phenomena such as second harmonic generation to identify non-centrosymmertric structures, such as collagen (1-3). Together, these two phenomena can provide distinctive optical signals which enables imaging of animal and human tissue with minimal phototoxicity to cells (4-6). The images can provide detailed information on cellular morphology and tissue architecture at submicron resolution in three dimensions to a depth over 1 mm below the sample surface depending on the tissue (7). The images can be acquired at a rate of one image per second containing greater than 105 pixels per an image (8,9).
Multiphoton microscopy in the identification of spermatogenesis
Microdissection testicular sperm extraction is the first line procedure for sperm retrieval in men with nonobstructive azoospermia (10,11). This procedure coupled with intracytoplasmic sperm injection into oocytes in vitro has made it possible for men with impaired sperm production to father children. The efficacy of this procedure is limited by the ability of the operating surgeon to identify seminiferous tubules containing spermatozoa. In searching for areas of spermatogenesis within the testis, the seminiferous tubules are currently evaluated by subjective assessment of their size and opacity using the operating microscope. With the current technique, sperm has been retrieved in 40-60% of cases, depending on the underlying cause of low sperm production (12,13). MPM provides the operating surgeon with the opportunity for real time visualization of seminiferous tubules in living tissue. The use of MPM in microdissection testicular sperm extraction has the potential to improve the success of this procedure.
Two studies have been performed exploring the potential application of MPM in the identification of spermatogenesis. In the first study, MPM was utilized to identify the stages of spermatogenesis in a rodent model. MPM was able to successfully identify the specific stage of spermatogenesis in a seminiferous tubule in fresh tissue. Additionally, the study showed that the tubules displayed significant differences in fluorescence depending on the presence or absence of sperm. Tubules with Sertoli-cell only histology had ample auto fluorescence in the 420-490 and 550-650 nm wavelength ranges. In contrast, tubules containing sperm had autofluorescence only in the 420-490 nm range (11). This difference in spectroscopic characteristics of the seminiferous tubules is not completely understood, but it could be utilized to improve the retrieval rate in testicular sperm extraction. It was proposed that the differences in autofluorescence could be due to a byproduct of steroid metabolism in Sertoli cells (11). The study also showed that there was minimal damage done to any DNA due to the MPM laser (11). These results are all encouraging and have led to further evaluation of the use of MPM in a human tissue.
In another study, MPM was utilized to analyze testicular biopsies from men with normal and abnormal spermatogenesis (Figure 1) (14). The diagnosis using MPM was correlated with the diagnosis made with hematoxylin and eosin (H&E) stained tissue samples. Figure 1 shows images taken using MPM and the associated H&E stained samples. The results from this study showed that overall concordance rate between MPM diagnoses and H&E histology was 86% (14). In addition, they found that the patients with nonobstuctive azoospermia had smaller seminiferous tubules compared to controls when measured with both MPM and by staining (14). This study further validates the potential impact MPM could have in the retrieval of sperm in men with nonobstructive azoospermia, however, there are still impediments.
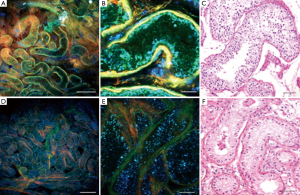
Safety of MPM laser must be addressed before MPM can be used to in microdissection testicular sperm extraction. There are investigators currently working on several prototypes to make the laser imaging technique accessible (15). These prototypes will need to be small enough so they can be utilized within the confines of the operating room. MPM safety concerns include thermal damage to tissue and, more importantly, non-linear damage to DNA. Although the rodent model showed minimal phototoxicity, this must be confirmed in a human model. Once these obstacles are overcome, MPM may take on a large role in the evaluation of men with nonobstructive azoospermia.
Multiphoton microscopy in the evaluation of bladder cancer
Bladder cancer is the fourth most common malignancy and ninth leading cause of cancer death in men (16). Urothelial carcinomas compose more than 90% of diagnosed bladder cancers (17). At the time of diagnosis, approximately 70% of these cancers represent superficial disease, which is non-muscle invasive (18). When these non-invasive cancers are detected and resected, most patients will likely have a good prognosis, however, it is often difficult to detect them and completely remove the tumors. The current standard of practice for detection of bladder cancer includes urine cytology and white light cystoscopy. Urine cytology provides a high specificity of 94%, but a sensitivity of only 35% (19). While white light cystoscopy is good for detecting large papillary tumors, it does not perform as well in finding flat and microscopic papillary tumors (20). In addition to difficulty in detection, the clinical management of non-invasive tumors is difficult because of very high recurrence rates of 31-78% at five years (20). One study has shown that up to half of these recurrences were actually found to be pre-existing tumors that were missed on initial diagnosis (21). MPM represents a possible solution to the difficulty in detecting and caring for patients with bladder cancer. MPM can be incorporated into the cystoscopy procedure in order to image the bladder tissue and search for potential suspicious areas.
In an initial study that explored the ability of MPM to diagnose various types of tumors in bladder tissue biopsies, it was found that the MPM fared well in comparison with the gold standard (H&E) (4). In the study the investigators were able to obtain diagnostic quality imaged by utilizing a combination of autofluorescence and second harmonic generation. There was no need for staining the human tissue with the MPM method. In a follow-up study, the same group performed a blinded experiment in which 77 fresh bladder biopsies were imaged by MPM and then submitted for a pathology diagnosis (9). Figure 2 shows the images taken with MPM compared to H&E stained slides of the tissue. The final diagnosis from each method was compared in order to find correlation data. The study found that the MPM diagnosis was accurate in 88% of cases based on the architecture and cytologic grade. The sensitivity was 90.4% and the specificity was 76.9%. The MPM diagnosis also had a 94% positive predictive value and 66% negative predictive value (9). These promising results further validate the potential usefulness of MPM in the evaluation of bladder cancer.
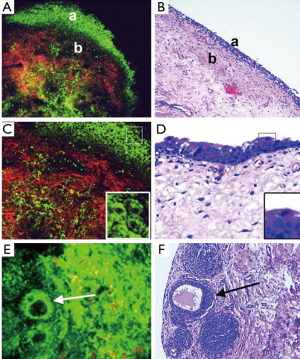
The first of two possible applications of MPM in bladder cancer includes its use in order to provide pathologists with an immediate impression to evaluate the specimen, similarly to the way in which frozen section analysis is utilized. Biopsy specimens are not sent for frozen section analysis because it is difficult to get any information from the small sample (9). MPM could be utilized to provide the surgeon with information about the adequacy of the specimen immediately after the biopsy is performed. The second possible application of MPM in bladder cancer is its use during the surgery as a multiphoton endoscope. There are other current technologies that are used by surgeons to help find areas of interest to biopsy such as photodynamic detection or fluorescence cystoscopy. One limitation of MPM is its limited field of view and slow image acquisition speed. It is theoretically possible that some of the current methods of finding areas of interest could be combined with MPM so that a desired area can be investigated.
In addition to the limitations mentioned, there are some other obstacles that will prevent the immediate adoption of MPM in bladder cancer detection. MPM can currently provide meaningful images only up to 0.5 mm below the surface of a sample in bladder. Given the thickness of the bladder wall, this limitation prevents the evaluation of invasion into the lamina propria and muscularis propria. Another limitation associated with MPM is that nuclei do not emit any fluorescence in the current wavelength ranges that the technology uses. They therefore appear as dark areas associated with no signal. Though nuclei themselves can often still be identified by the absence of fluorescence inside a brightly fluorescing cytoplasm, intranuclear details, such as nucleoli and chromatin pattern cannot be evaluated. This limitation prevents the differentiation of various cancer stages/grades. Despite these limitations MPM does have the potential to greatly impact the evaluation of bladder cancer. If this tool can be successfully transitioned into clinical use it could help the surgeon more specifically identify areas for resection, identify early lesions which are not picked up on white light endoscopy, and assess margins of a resection to ensure all tumor has been removed. This could potentially decrease recurrence of cancer, therefore decreasing the cost associated with bladder cancer and improving the disease outcomes.
Multiphoton microscopy in prostate surgery
Prostate cancer is the most common cancer in males, resulting in almost 27,000 deaths every year in the US (22). It can be successfully treated through a radical prostatectomy when the cancer is confined to the prostate gland. In addition to removal of the cancer, the success of this procedure is measured in the preservation of cavernous nerves surrounding the prostate that are responsible for erectile function. During the operation, the surgeon must examine the area beyond the gland for extension of the cancer, which is termed extra-prostatic extension. The prostate gland is surrounded by a capsule, which is surrounded by a complex fascia containing fat tissue, nerves, and vessels. The complex fascia contains both the nerve responsible for erectile function. While searching for any extra prostatic extension there is a risk of damaging the contents of the fascia resulting in impotence. The surgeon must therefore balance the goal of removing all the cancer with the goal of preserving potency. One possible solution for this problem is the use of frozen section analysis, however, this process still takes time and could risk damage to the contents of the fascia. It is estimated that up to 80% of the patients that undergo radical prostatectomy require treatment for erectile dysfunction following radical prostatectomy (8). MPM provides a potential solution to this problem, as it enables the visualization of living tissue and could help determine where these important structures lie within the fascia.
The use of MPM to visualize the prostate and periprostatic tissue was first tested in a rodent model. In this study, the investigators showed that they could successfully image the prostate using MPM and also showed good correlation between MPM and H&E staining (6). A follow-up human study was subsequently performed on 95 robotic radical prostatectomy samples, which included biopsies, surgical margins, and sections of excised prostate (8). This study further validated the usefulness of MPM in order to image the prostate and periprostatic space. The imaging technique enabled the identification of important anatomy within the prostatic fascia, such as blood vessels, nerve fibers, and underlying acini. MPM also enables the identification of normal prostate tissue, as can be seen in Figure 3 (8). This is of great value in potentially helping the surgeon minimize the risk of positive margins during a prostatectomy. Additionally, MPM can be used to distinguish between areas of benign inflammation and areas of cancer pathology, further aiding in the decisions that must be made when excising prostatic tissue.
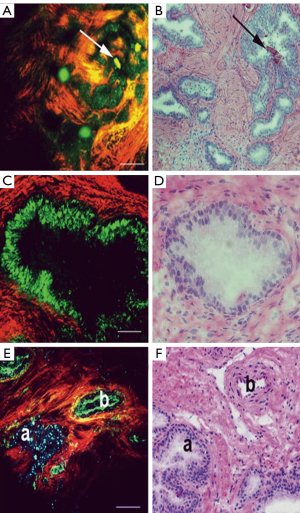
Although the results of the use in MPM in imaging the prostate are quite promising, there are some potential challenges that must be overcome before MPM can be used by the surgeon. The studies cited above utilized a commercial bench top multiphoton microscope, which would not be compatible with a clinical environment. Further validation must be performed to confirm the ability of MPM to be used to make the correct diagnosis. Even with these challenges, MPM has great potential to benefit patients undergoing radical prostatectomy. Further research must be performed so that this technology can make its way from the laboratory to the operating room.
Translation of multiphoton microscopy into the clinic
In recent years several groups have made advances in developing miniature multiphoton endoscopes with potential for clinical applications (15,23-29), including in vivo imaging of unstained tissues in rodents (30,31). These involve multiple different approaches including flexible small diameter endoscopes to image hollow organs as well as rigid needle-like endoscopes that could guide a biopsy. Clinical use of MPM has also been demonstrated with a microendoscope used in conjunction with the MPM system DermaInspect to characterize delayed wound healing in chronic leg ulcers in human subjects (32,33). An example in vivo image of an unstained rat kidney is shown in Figure 4. This was taken with a needle MPM endoscope using a rigid distal tip of 1.2 mm diameter and 8 cm length (31).
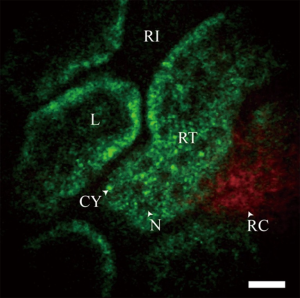
While these devices have shown great promise for the translation of MPM to the clinic, several challenges remain. The small field of view limits the diagnostic capabilities of most of these devices and motion artifacts can be expected to be more challenging in human imaging than in rodent imaging. While strategies have been suggested to improve the optical capabilities of these devices, it is also essential to ensure that these devices can be used safely without damaging tissue. Some studies have suggested that reasonable images can be obtained using excitation powers below the damage threshold, but further studies are needed to verify this and fully characterize the interaction of the high energy laser pulses with the tissue (11,34).
Nevertheless, MPM has shown great potential to aid in the real time cellular assessment of unstained tissue in vivo. These nonlinear optical techniques could prove to be very helpful in guiding a diagnostic biopsy and providing a surgeon with valuable tissue information intra-operatively.
Acknowledgements
None.
Footnote
Conflicts of Interest: The authors have no conflicts of interest to declare.
References
- Zipfel WR, Williams RM, Webb WW. Nonlinear magic: multiphoton microscopy in the biosciences. Nat Biotechnol 2003;21:1369-77. [PubMed]
- Denk W, Strickler JH, Webb WW. Two-photon laser scanning fluorescence microscopy. Science 1990;248:73-6. [PubMed]
- Zipfel WR, Williams RM, Christie R, et al. Live tissue intrinsic emission microscopy using multiphoton-excited native fluorescence and second harmonic generation. Proc Natl Acad Sci U S A 2003;100:7075-80. [PubMed]
- Mukherjee S, Wysock JS, Ng CK, et al. Human bladder cancer diagnosis using Multiphoton microscopy. Proc SPIE Int Soc Opt Eng 2009;7161:nihpa96839. [PubMed]
- Rogart JN, Nagata J, Loeser CS, et al. Multiphoton imaging can be used for microscopic examination of intact human gastrointestinal mucosa ex vivo. Clin Gastroenterol Hepatol 2008;6:95-101. [PubMed]
- Yadav R, Mukherjee S, Hermen M, et al. Multiphoton microscopy of prostate and periprostatic neural tissue: a promising imaging technique for improving nerve-sparing prostatectomy. J Endourol 2009;23:861-7. [PubMed]
- Horton NG, Wang K, Kobat D, et al. In vivo three-photon microscopy of subcortical structures within an intact mouse brain. Nat Photonics 2013;7. [PubMed]
- Tewari AK, Shevchuk MM, Sterling J, et al. Multiphoton microscopy for structure identification in human prostate and periprostatic tissue: implications in prostate cancer surgery. BJU Int 2011;108:1421-9. [PubMed]
- Jain M, Robinson BD, Scherr DS, et al. Multiphoton microscopy in the evaluation of human bladder biopsies. Arch Pathol Lab Med 2012;136:517-26. [PubMed]
- Schlegel PN. Testicular sperm extraction: microdissection improves sperm yield with minimal tissue excision. Hum Reprod 1999;14:131-5. [PubMed]
- Ramasamy R, Sterling J, Fisher ES, et al. Identification of spermatogenesis with multiphoton microscopy: an evaluation in a rodent model. J Urol 2011;186:2487-92. [PubMed]
- Ramasamy R, Yagan N, Schlegel PN. Structural and functional changes to the testis after conventional versus microdissection testicular sperm extraction. Urology 2005;65:1190-4. [PubMed]
- Okada H, Dobashi M, Yamazaki T, et al. Conventional versus microdissection testicular sperm extraction for nonobstructive azoospermia. J Urol 2002;168:1063-7. [PubMed]
- Najari BB, Ramasamy R, Sterling J, et al. Pilot study of the correlation of multiphoton tomography of ex vivo human testis with histology. J Urol 2012;188:538-43. [PubMed]
- Rivera DR, Brown CM, Ouzounov DG, et al. Compact and flexible raster scanning multiphoton endoscope capable of imaging unstained tissue. Proc Natl Acad Sci U S A 2011;108:17598-603. [PubMed]
- Jemal A, Siegel R, Xu J, et al. Cancer statistics, 2010. CA Cancer J Clin 2010;60:277-300. [PubMed]
- Epstein JI, Amin MB, Reuter VE. eds. Biopsy Interpretation of the Bladder. Lippincott Williams & Wilkins, 2010.
- Nieder AM, Soloway MS. Eliminate the term “superficial” bladder cancer. J Urol 2006;175:417-8. [PubMed]
- van Rhijn BW, van der Poel HG, van der Kwast TH. Urine markers for bladder cancer surveillance: a systematic review. Eur Urol 2005;47:736-48. [PubMed]
- Lee CS, Yoon CY, Witjes JA. The past, present and future of cystoscopy: the fusion of cystoscopy and novel imaging technology. BJU Int 2008;102:1228-33. [PubMed]
- Brausi M, Collette L, Kurth K, et al. Variability in the recurrence rate at first follow-up cystoscopy after TUR in stage Ta T1 transitional cell carcinoma of the bladder: a combined analysis of seven EORTC studies. Eur Urol 2002;41:523-31. [PubMed]
- Jemal A, Siegel R, Ward E, et al. Cancer statistics, 2007. CA Cancer J Clin 2007;57:43-66. [PubMed]
- Rivera DR, Brown CM, Ouzounov DG, et al. Use of a lensed fiber for a large-field-of-view, high-resolution, fiber-scanning microendoscope. Opt Lett 2012;37:881-3. [PubMed]
- Huland DM, Charan K, Ouzounov DG, et al. Three-photon excited fluorescence imaging of unstained tissue using a GRIN lens endoscope. Biomed Opt Express 2013;4:652-8. [PubMed]
- Fu L, Jain A, Cranfield C, et al. Three-dimensional nonlinear optical endoscopy. J Biomed Opt 2007;12:040501. [PubMed]
- Wu Y, Leng Y, Xi J, et al. Scanning all-fiber-optic endomicroscopy system for 3D nonlinear optical imaging of biological tissues. Opt Express 2009;17:7907-15. [PubMed]
- Tang S, Jung W, McCormick D, et al. Design and implementation of fiber-based multiphoton endoscopy with microelectromechanical systems scanning. J Biomed Opt 2009;14:034005. [PubMed]
- Jung JC, Schnitzer MJ. Multiphoton endoscopy. Opt Lett 2003;28:902-4. [PubMed]
- Engelbrecht CJ, Johnston RS, Seibel EJ, et al. Ultra-compact fiber-optic two-photon microscope for functional fluorescence imaging in vivo. Opt Express 2008;16:5556-64. [PubMed]
- Brown CM, Rivera DR, Pavlova I, et al. In vivo imaging of unstained tissues using a compact and flexible multiphoton microendoscope. J Biomed Opt 2012;17:040505. [PubMed]
- Huland DM, Brown CM, Howard SS, et al. In vivo imaging of unstained tissues using long gradient index lens multiphoton endoscopic systems. Biomed Opt Express 2012;3:1077-85. [PubMed]
- König K, Ehlers A, Riemann I, et al. Clinical two-photon microendoscopy. Microsc Res Tech 2007;70:398-402. [PubMed]
- König K, Bückle R, Weinigel M, et al. Clinical multiphoton tomography and clinical two-photon microendoscopy. San Jose, CA, 2009.
- Dela Cruz JM, McMullen JD, Williams RM, et al. Feasibility of using multiphoton excited tissue autofluorescence for in vivo human histopathology. Biomed Opt Express 2010;1:1320-30. [PubMed]