Clinical and investigative applications of Raman spectroscopy in Urology and Andrology
Introduction
Raman and Krishnan first investigated the Raman effect in 1928 (1). The principle of RS is based upon the detection of light when it interacts with a tissue interface. Briefly, incident photons from a diode laser source are inelastically scattered by their interaction with the chemical bonds of a sample. Inelastic scattering by an illuminating photon is a rare event that changes the vibrational state of the molecule’s bonds thereby producing a change in wavelength, also known as the Raman shift (2). The magnitude of the unique pattern of changes in energy relative to the excitation wavelength corresponds to the vibrational energy of a cell’s or tissues’ intrinsic molecular fingerprint. The resultant position, shape and intensity of each Raman peak conveys information about the chemical components of the tissue in question at a molecular level (3) (See Figure 1). The Raman spectrum may be plotted graphically as the Raman intensity against change in photon energy level (Figure 2).
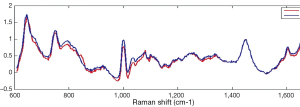
The usage of optical diagnostics to aide in clinical decision-making is a rapidly growing and evolving technology that may change and improve a patient’s therapeutic outcomes. Within the family of optical diagnostics, there is an emerging technology called Raman spectroscopy (RS) that has been in use for more than half-a-century entering clinical practice.
RS is may be performed in vivo or ex vivo and allows for a rapid, real-time assessment of cellular biochemistry with broad clinical applications. The potential advantages of RS include the unique opportunity to non-destructively analyze the chemical composition of single cells or tissue without obligate optical labels or fluorescent probes. Furthermore, the optical RS probe may be combined with endoscopic or laparoscopic procedures for near-real-time evaluation. The clinical applicability of RS was recently highlighted in a fertility review article from TIME magazine whereby Dr. Craig Niederberger states, “This holds a lot of promise”, in reference to RS and sperm selection (4).
Within the last decade, RS has been used in both investigative and clinical Urology. Within this review, we summarize the current literature utilizing this optical technology specific to Urology and Andrology.
RS and the bladder
RS has been extensively studied for malignant bladder pathologies. RS has been shown to provide clinically meaningful diagnostic data as an adjunct to cystoscopy, urine cytology or bladder biopsy, each of which have inherent limitations. For example, both white-light cystoscopy and urinary cytology have high inter and intra-observer variability (5). The mucosal lining of the bladder may be interrogated by RS and provide a picture of the molecular fingerprint of urothelial pathologies to aid directed biopsies or provide real-time information of cellular composition (5).
de Jong et al. first reported RS to differentiate layers of the bladder mucosa in 2002 (6). Using a guinea pig model, the authors collected in vitro samples of bladder tissue and collected spectra from 40-50 micrometer sections following liquid nitrogen freezing. RS was capable of distinguishing the urothelium, lamina propria and the muscularis layers (6).
de Jong and colleagues later report on RS’s discriminatory capabilities between nontumor bladder tissue and tumor in humans (7). Ex vivo tissue sections were compared to histopathologic review. The authors report a 94% sensitivity, a 92% specificity and a receiver operator characteristic curve area of 0.99 for discriminating tumor from nontumor with RS.
In 2010, Draga et al. report their initial experience using high-volume RS for the diagnosis of urothelial carcinoma in vivo (8). The authors measured spectra from 38 patients in vivo prior to a transurethral resection of bladder tumor (TURBT). The controls were taken from normal appearing bladder mucosa prior to biopsy for histopathological evaluation. After background noise was filtered, the normalized spectra were grouped according to pathological classifications. Multivariate logistic regression with principal component analysis was performed whereby the authors report an 85% sensitivity and 79% specificity for detection of urothelial carcinoma (8).
More recently in 2012, Barman et al. used a confocal Raman probe to demonstrate how RS may increase the specificity of bladder cancer detection from ex vivo malignant bladder specimens (9). Following TURBT, Raman spectra were collected from 14 normal specimens and 14 diseased specimens, which subsequently underwent routine pathologic evaluation with Hematoxylin and Eosin staining. After logistic regression and cross-validation, the authors found that RS may detect urothelial carcinoma ex vivo with 85.7% sensitivity and 100% specificity. The authors report a receiver operating characteristic curve area of 0.91 (9). The author’s utilization of a confocal Raman probe allows for a reduction of sampling error by collecting less confounding tissues from deeper layers. Such a probe is ideal for distinguishing high grade from low grade urothelial carcinoma and carcinoma in situ.
In effort to characterize the ability of RS to augment the accuracy of urine cytology Canetta et al. performed RS on an ex vivo bladder tumor cell-line exposed to urine (10). The authors used modulated RS, a technique employed to filter out fluorescence background noise, in conjunction with a cell line derived from recurrent human bladder tumors. The cell lines were exposed to donor urine for six hours and then RS was performed on the centrifuged samples. Controls were from a human urothelial cell line incubated with donor urine. The authors report at 80% sensitivity and 87% specificity for the discrimination between the two cell lines (10). While this study attempts to recapitulate a clinical scenario, the usage of donor urine certainly diminishes the validity of this ex vivo study. To this point, the authors found that the sensitivity and specificity increased to 98% and 95% respectively from cell lines not exposed to urine suggestive of donor urine causing degradation.
Shapiro and colleagues performed a corollary study whereby the authors collected urine samples from patients with urothelial carcinoma (low grade and high grade) and controls without cancer (11). Following collection of 50 mL of urine, the centrifuged pellet was re-suspended then analyzed with RS. The overall sensitivity and specificity was 96% and 90% respectively. Using mathematical modeling in conjunction with RS correctly assigned tumor grade in 88% of low-grade tumors and 98.6% of high-grade tumors (11).
In summary, RS may be a useful adjunct technology for the diagnosis of bladder cancer and other benign pathologies of the urothelium. Furthermore, RS may ultimately reduce the need for unnecessary bladder biopsies however future in vivo studies must be performed.
RS and the prostate
The usage of RS for the diagnosis of prostate cancer is more sparse than that of bladder cancer. Initial work by Crow et al. used RS to differentiate between several well-known prostate cancer cell lines. The authors recorded spectra from two well-differentiated, androgen sensitive cell lines (LNCaP and PCa 2b) and two poorly differentiated, androgen-insensitive cell lines (DU145 and PC3). RS was capable to distinguish the cell lines with an overall sensitivity of 98% and a specificity of 99% (12).
The authors performed a follow-up study on prostate tissue following transurethral resection of the prostate (TURP). The spectra were correlated with histopathology to generate an accuracy of diagnosis of cancer versus benign prostatic hyperplasia of 86% (13). Prieto et al. performed a similar study with a higher predictive algorithm using 56 prostate specimens (14). RS was capable of distinguishing between benign prostatic hyperplasia, prostatitis and carcinoma with sensitivities of 92%, 100% and 85% respectively and specificities of 94%, 94%, 98% (14). RS shows promise for the usage of intraoperative diagnosis of margin status during radical prostatectomy and/or lymph node positivity, which may change the operative and clinical course for patients.
RS and the kidney/urolithiasis
RS has been investigated for both benign and malignant diseases associated with the kidney. With regards to the evaluation of ex vivo renal tumors, Bensalah et al. recently investigated the ability of RS to assess renal tumors at the time of surgery (15). Spectra were acquired prospectively on surgical renal specimens from both partial and radical nephrectomies for renal carcinoma subtypes and compared it to histopathology. The authors report that RS can distinguish between low Fuhrman grades and high Furhman grades with 84% sensitivity and 80% specificity. Similarly, histologic subtypes (clear-cell, papillary or chromophobe) were differentiated with RS with 96% sensitivity and 87% specificity. This study highlights the potential for intraoperative usage of RS to guide the surgical management of renal neoplasms as an “optical biopsy” tool.
RS has long been used in the analysis of biomaterials, therefore it is not surprising that RS has been used for urinary calculi analysis. In 1983, Daudon et al. reported on the usage of RS to classify kidney stones by chemical composition (16). The group found that commonly found urinary calculi (i.e., calcium phosphate, calcium oxalate, struvite, and calcium carbonate) are distinguished based on spectral wavelengths (16). More recently, Durickovic and colleagues used RS to differentiate calcium oxalate monohydrate and dehydrate stones following morcellation (17). Similarly, Chiu et al. performed RS on urine samples following ureteroscopy and laser lithotripsy. Using the micro-stone fragments within the urine, the authors performed RS to analyze the stone composition based on vibrational wave numbers (18). The potential clinical applicability of RS in the treatment of nephrolithiasis is vast. RS may provide ex vivo, real-time stone analysis enabling the clinical Urologist to intervene with metabolic and/or dietary intervention(s) (19).
RS and male infertility
Perhaps the most recent investigations using RS within Urology include the evaluation of the male testis for active spermatogenesis. Liu and colleagues from Shanghai Jiao Tong University Renji Hospital first reported on the ability of confocal RS to distinguish zona pellucida (ZP) bound sperm from unbound sperm (20). It is known that most sperm bound to the ZP have normal morphology and chromatin structure (21) and may improve the clinical pregnancy rate. Therefore, the authors collected 100 ZP bound sperm and individually scanned them with RS only to find a significant difference in several spectra compared to 100 ZP unbound sperm as well as differences in the mean spectral intensities (20).
The same group in Shanghai has previously investigated the use of RS to differentiate Sertoli cells from 100 testicular biopsies following microdissection testicular sperm extraction (micro-TESE) from men with non-obstructive azoospermia versus obstructive azoospermia. Briefly, Ma et al. report four spectral intensity differences noted with RS that distinguish between sertoli cells between groups (22).
RS has also been used to investigate testicular microlithasis, which has been associated with gonadal dysgenesis, testicular torsion, male infertility, and germ cell tumors. It is not currently known the degree of impact testicular microlithasis may play on each of these conditions. To this end, De Jong and colleagues examined testicular microliths using RS and determined that their composition to be primarily hydroxyapatite based upon its spectral wavelengths (23). Using RS, a better understanding into the exact composition of testicular microliths may shed light on their initiating pathologic processes.
Currently, RS is under investigation for the identification of active spermatogenesis following human testicular biopsy with the hope to integrate RS for intraoperative guidance alongside micro-TESE.
Future directions
Undoubtedly, further in vivo studies examining the safety and feasibility of RS will be necessary to prove if RS is a useful adjunct for the real-time diagnosis of a variety of Urologic conditions. The incorporation of fiber optic probes into laparoscopes, cystoscopes, Foley catheters, etc. would be prudent for further investigation and incorporation of Raman technology into operative Urology. Furthermore, RS may be used as an adjunct to computed tomography, ultrasound and/or magnetic resonance imaging to provide more accurate differential diagnoses. RS may even be used for serum studies (i.e., novel biomarkers for prostate cancer) or urine studies, which may provide rapid analysis and diagnosis. RS may be used to assess tumor margin status intracorporeally or be used prior to resection to eliminate need for random, surveillance biopsies.
Conclusions
RS is a valuable and unique diagnostic tool used in a variety of Urologic diseases. It allows for real-time interrogation of biologic tissues without the use of any chemical dyes or labels. The spectral output obtained by RS is easily interpretable and may provide clinically meaningful information. Currently, RS has been used to distinguish benign from malignant tissues in several of the genitourinary organs (kidney, prostate, and bladder). RS holds great promise as an “optical biopsy” tool that may influence clinical decision-making and revolutionize the fields of Urology and Andrology.
Acknowledgements
None.
Footnote
Conflicts of Interest: The authors have no conflicts of interest to declare.
References
- Raman CV, Krishnan KS. A new type of secondary radiation. Nature 1928;121:501-2.
- Crow P, Stone N, Kendall CA, et al. Optical diagnostics in urology: current applications and future prospects. BJU Int 2003;92:400-7. [PubMed]
- Crow P, Uff JS, Farmer JA, et al. The use of Raman spectroscopy to identify and characterize transitional cell carcinoma in vitro. BJU Int 2004;93:1232-6. [PubMed]
- Kluger J, Park A. Frontiers of Fertility. TIME magazine 2013.
- Cauberg EC, de Bruin DM, Faber DJ, et al. A new generation of optical diagnostics for bladder cancer: technology, diagnostic accuracy, and future applications. Eur Urol 2009;56:287-96. [PubMed]
- de Jong BW, Bakker Schut TC, Wolffenbuttel KP, et al. Identification of bladder wall layers by Raman spectroscopy. J Urol 2002;168:1771-8. [PubMed]
- de Jong BW, Schut TC, Maquelin K, et al. Discrimination between nontumor bladder tissue and tumor by Raman spectroscopy. Anal Chem 2006;78:7761-9. [PubMed]
- Draga RO, Grimbergen MC, Vijverberg PL, et al. In vivo bladder cancer diagnosis by high-volume Raman spectroscopy. Anal Chem 2010;82:5993-9. [PubMed]
- Barman I, Dingari NC, Singh GP, et al. Selective sampling using confocal Raman spectroscopy provides enhanced specificity for urinary bladder cancer diagnosis. Anal Bioanal Chem 2012;404:3091-9. [PubMed]
- Canetta E, Mazilu M, De Luca AC, et al. Modulated Raman spectroscopy for enhanced identification of bladder tumor cells in urine samples. J Biomed Opt 2011;16:037002. [PubMed]
- Shapiro A, Gofrit ON, Pizov G, et al. Raman molecular imaging: a novel spectroscopic technique for diagnosis of bladder cancer in urine specimens. Eur Urol 2011;59:106-12. [PubMed]
- Crow P, Barrass B, Kendall C, et al. The use of Raman spectroscopy to differentiate between different prostatic adenocarcinoma cell lines. Br J Cancer 2005;92:2166-70. [PubMed]
- Crow P, Molckovsky A, Stone N, et al. Assessment of fiberoptic near-infrared raman spectroscopy for diagnosis of bladder and prostate cancer. Urology 2005;65:1126-30. [PubMed]
- Prieto MC, Crow P, Kendall C, et al. Urological applications of Raman spectroscopy for improved malignant diagnostics. Proc SPIE 2004.5321.
- Bensalah K, Fleureau J, Rolland D, et al. Raman spectroscopy: a novel experimental approach to evaluating renal tumours. Eur Urol 2010;58:602-8. [PubMed]
- Daudon M, Protat MF, Reveillaud RJ, et al. Infrared spectrometry and Raman microprobe in the analysis of urinary calculi. Kidney Int 1983;23:842-50. [PubMed]
- Durickovic I, Thiébaud L, Bourson P, et al. Spectroscopic characterization of urea aqueous solutions: experimental phase diagram of the urea-water binary system. Appl Spectrosc 2013;67:1205-9. [PubMed]
- Chiu YC, Yang HY, Lu SH, et al. Micro-Raman spectroscopy identification of urinary stone composition from ureteroscopic lithotripsy urine powder. J Raman Spectrosc 2010;41:136-41.
- Miernik A, Eilers Y, Bolwien C, et al. Automated analysis of urinary stone composition using Raman spectroscopy: pilot study for the development of a compact portable system for immediate postoperative ex vivo application. J Urol 2013;190:1895-900. [PubMed]
- Liu F, Zhu Y, Liu Y, et al. Real-time Raman microspectroscopy scanning of the single live sperm bound to human zona pellucida. Fertil Steril 2013;99:684-689.e4.
- Liu DY, Baker HW. Human sperm bound to the zona pellucida have normal nuclear chromatin as assessed by acridine orange fluorescence. Hum Reprod 2007;22:1597-602. [PubMed]
- Ma M, Yang S, Zhang Z, et al. Sertoli cells from non-obstructive azoospermia and obstructive azoospermia patients show distinct morphology, Raman spectrum and biochemical phenotype. Hum Reprod 2013;28:1863-73. [PubMed]
- De Jong BW, De Gouveia Brazao CA, Stoop H, et al. Raman spectroscopic analysis identifies testicular microlithiasis as intratubular hydroxyapatite. J Urol 2004;171:92-6. [PubMed]