Low-intensity extracorporeal shock wave therapy for male chronic pelvic pain syndrome: a systematic review and meta-analysis
Introduction
When low-intensity extracorporeal shock wave therapy (LI-ESWT) is applied to an organ, it carries an energy that can be noninvasively focused to affect a distant selected anatomical region. The shock waves interact with the targeted tissues and induce a cascade of biological reactions, resulting in the release of growth factors, which trigger tissue neovascularization and a consequent improvement in blood supply (1). LI-ESWT has been used to treat male erectile dysfunction (2), non-healing wounds (3), musculoskeletal disorders (4), and myocardial infarction (5).
Several encouraging studies have recently analyzed the efficacy of LI-ESWT for patients with chronic pelvic pain syndrome (CPPS) (6-8). However, some studies showed deterioration in total National Institutes of Health Chronic Prostatitis Symptom Index (NIH-CPSI) scores, which includes subscales for pain, urinary function, and quality of life (QoL), at week 24 of follow-up (9-11). Our goal in this study was to examine the available data to determine the efficacy of LI-ESWT for the treatment of CPPS. We present the following article in accordance with the PRISMA reporting checklist (available at http://dx.doi.org/10.21037/tau-20-1423).
Methods
Search strategy
We performed a systematic search of the Embase and PubMed databases and the Cochrane Register for studies on LI-ESWT and CPPS. The search terms were shock wave AND (chronic pelvic pain syndrome OR chronic prostatitis OR prostatitis OR chronic abacterial prostatitis OR noninflammatory chronic pelvic pain syndrome). We analyzed the treatment efficacy of LI-ESWT for patients with CPPS and the relationships among therapeutic efficacy, protocols, and setup parameters. Additional data were identified by searching relevant conference abstracts, scanning the reference lists of articles, and corresponding with study authors using the approach recommended by the Preferred Reporting Items for Systematic Reviews and Meta-Analyses (PRISMA) guidelines (12). A flow diagram of the study selection is shown in Figure 1.
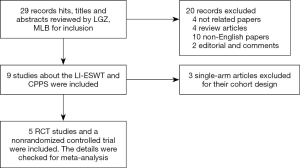
Inclusion and exclusion criteria
All controlled clinical trials that investigated the efficacy of LI-ESWT for CPPS and were published from January 2005 through to August 2019 were included. All literature reviews, editorial comments, animal studies, background publications, case reports, and single-arm studies were excluded.
Data extraction and synthesis
The abstracts were reviewed independently by the 2 authors (LM and GL) to determine inclusion eligibility following a standardized form. The study's details, assessment tools, setup parameters of the LI-ESWT machine, treatment protocols, and P values were extracted manually from each study (LM), and the data were verified (GL). Follow-up data were also extracted from these studies.
Statistical analysis
The data were analyzed using RevMan 5.3 software (Cochrane Collaboration, London, UK). Appropriate statistical analysis methods and effect sizes were used following the evaluation and different data types. The risk difference (RD) and a 95% confidence interval (CI) were calculated for discontinuous variables. The weighted mean difference (MD) and 95% CI were calculated for continuous variables. The I2 test assessed the heterogeneity between studies. A random-effects model analyzed data with heterogeneity. Data without significant heterogeneity (P>0.05, I2 ≤50%) were analyzed by a fixed-effects model. The results of the meta-analysis were presented as forest plots. The risk of bias was investigated with the Cochrane Collaboration tool. Publication bias was shown in funnel plots. The NIH-CPSI scores before and after LI-ESWT were provided in these included studies. Therefore, a meta-analysis could be performed.
Results
This review included 6 studies involving 317 patients who were treated with different medical devices in different countries. Details of the studies are presented in Table 1. Patient inclusion criteria were based on the NIH classification (8-10,13-15). Some of the studies required the total scores and pain scores to meet specified requirements (10,13,15). Most of the studies emphasized a medical history of more than 3 months, and only the study by Zhang et al. failed to include a history of more than 3 months (15). Zeng et al.’s study included patients for whom drug treatments were ineffective (13). The studies by Zhang et al. (15) and Pajovic et al. (10) used the drug treatment group as the control group, while the other studies used a sham control (8,9,13,14). Four studies introduced evaluation criteria for treatment efficacy (8,10,13,15). Pajovic et al. (10) and Zhang et al. (15) used the primary endpoint of QoL score ≤2 at the treatment endpoint, and the secondary endpoints were a greater than 50% reduction in pain scores and total NIH-CPSI scores. In the study by Zeng et al. (13), responders were defined as men who experienced a decrease of 6 or more points in the total NIH-CPSI score (perceptible improvement) compared to baseline, or a 12 point decrease (clinically significant improvement), which reflected a 50% decrease in the total NIH-CPSI score compared to baseline. Zimmermann et al. (8) considered a decrease of 5 or more points in the total NIH-CPSI score as the criterion for treatment efficacy. Zimmermann et al. (8), Moayednia et al. (9), Vahadpour et al. (14), and Zhang et al. (15) employed the Duolith® SD1 and MP100 devices (Storz Medical, Tägerwilen, Switzerland), Zeng et al. (13) used the HB-ESWT 1® device (Haibin Medical Equipment Co. Ltd., Beijing, China), and Pajovic et al. (10) used the Lubisone KM-2000 S device (K1 Med Co., Ltd., Seoul, Korea). The device used in the study by Zhang et al. (15) was a shock wave unit with a radial shock wave source, but the devices used in the other 5 studies had a focus shock wave source (8-10,13,14). The setup parameters of LI-ESWT differed among the studies. The energy flux density (EFD) in most studies was 0.25 mJ/mm2, while only 2 studies had lower EFDs of 0.05 and 0.06 mJ/mm2 (9,13). In most of the studies, each treatment consisted of 3,000 shock wave pulses, while only 1 study administered fewer shock wave pulses (2,000) (13). The treatment course ranged from 4 to 12 weeks (8-10,13,14). Each study’s endpoint was the longest follow-up time, namely, 12 (8,13-15) or 24 weeks (9,10) after LI-ESWT.
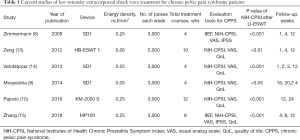
Full table
The quality of the studies and the risk of bias were assessed by the Cochrane Collaboration tool (shown in Figures 2,3). Only 1 of the 6 studies did not use a randomization method (14). The randomized controlled trials (RCTs) reported that the patients were assigned randomly into the LI-ESWT or control groups but did not further describe the randomization process (8-10,14). Only Zeng et al. mentioned the closed-envelope method. Most of the studies did not describe how the doctors were blinded to participants’ allocation (9,10,13-15). Blinding of the physician would be difficult to maintain since the LI-ESWT output energy would need to be reduced to zero for patients in the control group receiving sham treatment. Only Zimmermann et al. (8) described the process of ensuring double-blinding. As shown in Figure 3, 66.7% of the studies had an unclear risk of bias in randomization, and only 16.7% of the studies had good blinding for both patients and doctors.
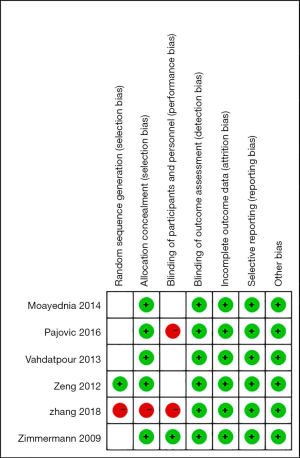
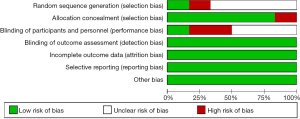
At 12 weeks after treatment, the data indicated that LI-ESWT was effective for the treatment of CPPS (RD: 0.46; 95% CI, 0.28–0.63; P<0.00001) according to the overall meta-analysis (shown in Figure 4). Additionally, a subgroup analysis was conducted in this study. The total NIH-CPSI scores at 1 and 12 weeks after LI-ESWT were significantly decreased (MD: −4.46; 95% CI, −6.67–−2.25; P<0.00001, shown in Figure 5; and MD: −5.00; 95% CI, −7.24–−2.75; P<0.0001, shown in Figure 6, respectively). However, the total NIH-CPSI scores at 24 weeks after treatment were not significantly decreased (MD: −4.59, 95% CI, −12.63–3.46; P=0.26, shown in Figure 7).
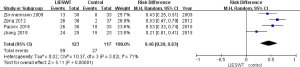
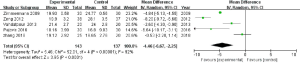
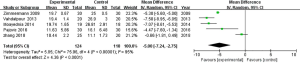

The studies were divided into 3 groups based on time after LI-ESWT (1, 12, and 24 weeks), and the visual analog scale (VAS) scores were compared. According to the meta-analysis, the patients’ VAS scores improved significantly at 12 weeks after LI-ESWT (MD: −3.16; 95% CI, −5.03–−1.30; P=0.0009), but did not improve significantly at 1 and 24 weeks (MD: −1.18; 95% CI, −2.55–0.20; P=0.09; and MD: −3.39; 95% CI, −10.10–3.32; P=0.32, respectively).
The urinary symptom scores were not significantly improved at 1 and 24 weeks after treatment (MD: −0.24, 95% CI, −1.17–0.69; P=0.61; and MD: −1.02; 95% CI, −2.01–0.05; P=0.06, respectively), but were significantly improved at 12 weeks after treatment (MD: −1.29; 95% CI, −1.95–−0.64; P=0.0001).
Furthermore, the QoL scores were significantly improved at 12 weeks after treatment (MD: −1.31; 95% CI, −2.33–−0.30; P=0.01), but not at 1 or 24 weeks after treatment (MD: −1.08, 95% CI, −2.57–0.41; P=0.15; and MD: -1.78; 95% CI, −5.08–1.52; P=0.29, respectively).
Different LI-ESWT setup parameters, such as EFDs, resulted in differences in reported efficacy. The studies were divided into 2 groups according to EFD. The total NIH-CPSI scores using the EFDs 0.06 and 0.25 mJ/mm2 were significantly decreased (MD: −8.20; 95% CI, −9.72–−6.68; P<0.00001; and MD: −3.46; 95% CI, −5.86–−1.06; P=0.005, shown in Figure 8, respectively).
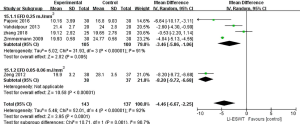
Discussion
This systematic review and meta-analysis of 6 studies involving 317 male patients revealed significant improvements in total NIH-CPSI scores, QoL, pain scores, and urinary symptom scores in the LI-ESWT group compared to the control group at 12 weeks after treatment.
Some deterioration of total NIH-CPSI scores, pain scores, QoL, and urinary symptom scores was observed at 24 weeks of follow-up compared with 1 week. As described in the study by Fojiecki et al., LI-ESWT is likely to relieve pain in CPPS patients in the short term (16,17). One potential explanation could be the mechanism of LI-ESWT. Mechanotransduction (18), microcavitation (19), and thermodynamic effects lead to the energy transfer of LI-ESWT. LI-ESWT applies a mechanical force on cell membranes and contents. LI-ESWT can regulate cellular signal transduction and affects the transcription and modification of intracellular proteins (20). LI-ESWT shock waves are transformed into biochemical signals through the process of mechanotransduction, which may hyperstimulate nociceptors and interrupt the former pain memory nerve impulses to achieve ‘reprogramming’, thereby alleviating pain (21). Furthermore, cavitation bubbles are generated and popped, producing secondary energy waves called microjets that lead to additional mechanical forces, which increases local microvascularity (8), reduces pain, and helps heal tissue (22). This mechanism may explain the short-term nature of shock waves’ effects, as pain sensations can be prevented relatively transiently without persistent modulation of sensitivity at the treatment area.
The longer-term effect of LI-ESWT may involve other mechanisms. A possible cause of CPPS is immunogenic inflammation, which activates prostate afferent nerves and induces inflammation, prostate pain, and referred pain (23). LI-ESWT may trigger an anti-inflammatory response related to the mechanism of mechanotherapy, inducing different biological reactions and immunomodulation pathways. LI-ESWT suppresses the production of proinflammatory cytokines (IL-1α, IL-4, IL-6, etc.), chemokines (CCL2, CCL12, etc.), and matrix metalloproteinases (MMPs) (23). LI-ESWT is administered at different time points, and energies exert different effects on inflammatory processes (24). Moreover, long-term effects of LI-ESWT are considered to be mediated by multiple overlapping and crosstalking signaling pathways, for example, protein synthesis/secretion, structural reorganization, proliferation, and vitality (25). Specific cellular processes and molecules modulated by LI-ESWT include extracellular signal-regulated kinase (ERK) (26), focal adhesion kinase (FAK) (27), ATP/P2X7 (28), Wnt (28), protein kinase R-like endoplasmic reticulum kinase/activated transcription factor (PERK/ATF) (29), vascular endothelial growth factor (VEGF), and brain-derived neurotrophic factor (BDNF) (30).
BDNF promotes the survival of neurons and stimulates the growth and differentiation of new neurons. LI-ESWT has been shown to improve the expression of BDNF at a level that was maintained until 26 days after nerve injury (31). This prolonged expression may likely sustain neurotrophic healing beyond what can be expected naturally and without intervention. The LI-ESWT-mediated BDNF activation mechanism was studied in RT4-D6P2T Schwann cells and was revealed to be related to the activation of PERK/ATF4 (31). The p75 gene and p-ERK1/2 were considered as Schwann cell activation-related markers and were upregulated after LI-ESWT (32). The PERK inhibitor GSK265615753 decreased LI-ESWT-mediated eIF2α phosphorylation and downstream target gene ATF4 expression. Accordingly, the expression of BDNF was also significantly reduced in this context (31).
Other studies have revealed that LI-ESWT induces mesenchymal stem cells to express VEGF, and VEGF then upregulates the PI3K/AKT/mTOR pathway, thus inducing autophagy. Autophagy and apoptosis assays have shown that LI-ESWT activates autophagy and effectively decreases apoptosis. Some studies applied LI-ESWT to the injured spinal cords of rats. In the LI-ESWT group, CD31 and alpha-smooth muscle actin expression increased, and TUNEL-positive cells were reduced in the injured spinal cords (33). VEGF expression also significantly improved in the NeuN-, GFAP, and Olig2-labeled cells. These studies suggest that the neuroprotective effects of VEGF induced by LI-ESWT may decrease axonal damage and cell apoptosis and promote locomotor and sensory functions after spinal cord injury.
Another potential explanation for the effect of short term of LI-EWST in this article is that only 2 of the 6 studies performed a long-term follow-up at 24 weeks. In the study by Pajovic et al. (10), the treatment effects of LI-ESWT + triple therapy (α-blocker, anti-inflammatory agent, and muscle relaxant) were compared with those of triple therapy alone to evaluate the long-term efficacy of LI-ESWT for CPPS (24 weeks). Combination therapy might have been the main reason for the positive long-term effects. A randomized controlled study by Moaydenia et al. (9) indicated that the NIH-CPSI scores, QoL, urinary symptom scores, and pain domain scores gradually deteriorated from week 16 to week 24. The scores recorded at week 24 were close to those at baseline, implying a questionable long-term effect of LI-ESWT on CPPS. However, the total treatment course in the study by Moaydenia et al. was 4 weeks, whereas the total treatment course in the study by Pajovic et al. was 12 weeks. The effect of LI-ESWT should also correspond to the dose applied (34). In a single-arm study, treatment efficacy was determined over a longer treatment course, such as 6 and 12 months, after applying a protocol of 2,500 pulses once a week for 1 month (11). The long-term effect of LI-ESWT on CPPS is controversial due to different mechanisms, small sample sizes, different protocols, and different treatment courses. In the future, more long-term, multicenter, large RCTs should be performed to determine the efficacy of LI-ESWT for CPPS.
This systematic review and meta-analysis were the first to examine the efficacy of LI-ESWT for the treatment of CPPS. However, our study had some limitations. The sample sizes in most trials were small. The largest sample size in our meta-analysis only included 80 male patients (10). No blinding of personnel occurred in most of the RCTs (9,13,14).
Regarding patient demographics, few studies described the selection criteria and previous treatment strategies. The short-term follow-up of the included studies is also an important limitation. For most studies, follow-up was limited to approximately 12 weeks. Therefore, the robustness of this approach remains unknown, and long-term data are needed. The 6 studies in this meta-analysis included 5 RCTs and a nonrandomized controlled trial. No placebo response was observed in the sham-treated arm, which is unusual. Previously treated men were also included in some trials (10,13). If any bias occurred, it would substantially affect the interpretation of the results of this meta-analysis.
Additionally, our study had a very high level of heterogeneity (I2 =71%). The potential reasons for this heterogeneity might be subject selection and the therapeutic regimen. Pajovic et al. (10) used 3,000 treatment shocks over 12 weeks, and Zeng et al. (13) applied 2,000 treatment shocks over 10 weeks, whereas other studies administered 3,000 treatment shocks over 4 weeks. Also, Pajovic et al. (10) and Zhang et al. (15) used the drug therapy group as the control group to reveal the effect of LI-ESWT.
Different setup parameters and different treatment protocols of LI-ESWT have a substantial influence on therapeutic efficacy. The clinical outcome of LI-ESWT is closely related to the energy delivered to the target unit area or EFD. The EFDs used in the included studies varied from 0.05 to 0.25 mJ/mm2. Based on this review, we could not determine the best EFD for CPPS therapy. Most of the included studies used an EFD of 0.25 mJ/mm2, which Zimmermann et al. first reported in 2009 (8). Most subsequent studies adopted this EFD and presented encouraging results. Additional studies and a longer duration of treatment are needed to establish whether therapeutic efficacy is positively correlated with energy density.
Future LI-ESWT research should rely on basic science and clinical studies. Extensive basic research is necessary to understand the mechanism of action of LI-ESWT. Several devices with a radial or focus shock wave source, such as electrohydraulic, electromagnetic, and piezoelectric generators, are available on the market, and each type of device employs a different treatment protocol. Further studies are needed to evaluate the different devices and protocols. Well-designed and long-term multicenter RCTs are urgently needed to estimate the real potential and ultimate use of these devices in patients with CPPS.
Conclusions
In this meta-analysis on the efficacy of LI-ESWT for CPPS, total NIH-CPSI scores, QoL, pain scores, and urinary symptom scores transiently improved in the LI-ESWT group compared with the control group. Future research may elucidate the mechanisms underlying the effect of LI-ESWT on CPPS. Well-designed and long-term multicenter RCTs are urgently needed to estimate the real potential and ultimate use of these devices before LI-ESWT is widely applied as a treatment for CPPS.
Acknowledgments
Funding: This study was supported by the Beijing Municipal Science & Technology Commission (No. Z171100001017133).
Footnote
Reporting Checklist: The authors have completed the PRISMA reporting checklist. Available at http://dx.doi.org/10.21037/tau-20-1423
Conflicts of Interest: Both authors have completed the ICMJE uniform disclosure form (available at http://dx.doi.org/10.21037/tau-20-1423). Dr. GI and Dr. LM report grants from Beijing Municipal Science & Technology Commission, during the conduct of the study.
Ethical Statement: The authors are accountable for all aspects of the work in ensuring that questions related to the accuracy or integrity of any part of the work are appropriately investigated and resolved.
Open Access Statement: This is an Open Access article distributed in accordance with the Creative Commons Attribution-NonCommercial-NoDerivs 4.0 International License (CC BY-NC-ND 4.0), which permits the non-commercial replication and distribution of the article with the strict proviso that no changes or edits are made and the original work is properly cited (including links to both the formal publication through the relevant DOI and the license). See: https://creativecommons.org/licenses/by-nc-nd/4.0/.
References
- Rassweiler JJ, Knoll T, Köhrmann KU, et al. Shock wave technology and application: an update. Eur Urol 2011;59:784-96. [Crossref] [PubMed]
- Olsen AB, Persiani M, Boie S, et al. Can low-intensity extracorporeal shockwave therapy improve erectile dysfunction? A prospective, randomized, double-blind, placebo-controlled study. Scand J Urol 2015;49:329-33. [Crossref] [PubMed]
- Hayashi D, Kawakami K, Ito K, et al. Low-energy extracorporeal shock wave therapy enhances skin wound healing in diabetic mice: a critical role of endothelial nitric oxide synthase. Wound Repair Regen 2012;20:887-95. [Crossref] [PubMed]
- Hazan-Molina H, Reznick AZ, Kaufman H, et al. Periodontal cytokines profile under orthodontic force and extracorporeal shock wave stimuli in a rat model. J Periodontal Res 2015;50:389-96. [Crossref] [PubMed]
- Becker M, Goetzenich A, Roehl AB, et al. Myocardial effects of local shock wave therapy in a Langendorff model. Ultrasonics 2014;54:131-6. [Crossref] [PubMed]
- Zimmermann R, Cumpanas A, Hoeltl L, et al. Extracorporeal shock-wave therapy for treating chronic pelvic pain syndrome: a feasibility study and the first clinical results. BJU Int 2008;102:976-80. [Crossref] [PubMed]
- Marszalek M, Berger I, Madersbacher S. Low-energy extracorporeal shock wave therapy for chronic pelvic pain syndrome: finally, the magic bullet? Eur Urol 2009;56:425-6. [Crossref] [PubMed]
- Zimmermann R, Cumpanas A, Miclea F, et al. Extracorporeal shock wave therapy for the treatment of chronic pelvic pain syndrome in males: a randomised, double-blind, placebo-controlled study. Eur Urol 2009;56:418-24. [Crossref] [PubMed]
- Moayednia A, Haghdani S, Khosrawi S, et al. Long-term effect of extracorporeal shock wave therapy on the treatment of chronic pelvic pain syndrome due to non bacterial prostatitis. J Res Med Sci 2014;19:293-6. [PubMed]
- Pajovic B, Radojevic N, Dimitrovski A, et al. Comparison of the efficiency of combined extracorporeal shock-wave therapy and triple therapy versus triple therapy itself in Category III B chronic pelvic pain syndrome (CPPS). Aging Male 2016;19:202-7. [Crossref] [PubMed]
- Al Edwan GM, Muheilan MM, Atta ON. Long term efficacy of extracorporeal shock wave therapy [ESWT] for treatment of refractory chronic abacterial prostatitis. Ann Med Surg (Lond) 2017;14:12-7. [Crossref] [PubMed]
- Moher D, Liberati A, Tetzlaff J, et al. Preferred reporting items for systematic reviews and meta-analyses: the PRISMA statement. Ann Intern Med 2009;151:264-9, W64.
- Zeng XY, Liang C, Ye ZQ. Extracorporeal shock wave treatment for non-inflammatory chronic pelvic pain syndrome: a prospective, randomized and sham-controlled study. Chin Med J (Engl) 2012;125:114-8. [PubMed]
- Vahdatpour B, Alizadeh F, Moayednia A, et al. Efficacy of extracorporeal shock wave therapy for the treatment of chronic pelvic pain syndrome: a randomized, controlled trial. ISRN Urol 2013;2013:972601 [Crossref] [PubMed]
- Zhang ZX, Zhang D, Yu XT, et al. Efficacy of Radial Extracorporeal Shock Wave Therapy for Chronic Pelvic Pain Syndrome: A Nonrandomized Controlled Trial. Am J Mens Health 2019;13:1557988318814663 [Crossref] [PubMed]
- Fojecki GL, Tiessen S, Osther PJ. Extracorporeal shock wave therapy (ESWT) in urology: a systematic review of outcome in Peyronie's disease, erectile dysfunction and chronic pelvic pain. World J Urol 2017;35:1-9. [Crossref] [PubMed]
- Rassweiler J. Re: Extracorporeal Shock Wave Therapy (ESWT) in Urology: A Systematic Review of Outcome in Peyronie's Disease, Erectile Dysfunction, and Chronic Pelvic Pain. Eur Urol 2018;74:115-7. [Crossref] [PubMed]
- d'Agostino MC, Craig K, Tibalt E, et al. Shock wave as biological therapeutic tool: From mechanical stimulation to recovery and healing, through mechanotransduction. Int J Surg 2015;24:147-53. [Crossref] [PubMed]
- Ohl SW, Klaseboer E, Khoo BC. Bubbles with shock waves and ultrasound: a review. Interface Focus 2015;5:20150019 [Crossref] [PubMed]
- Xin ZC, Xu YD, Lin G, et al. Recruiting endogenous stem cells: a novel therapeutic approach for erectile dysfunction. Asian J Androl 2016;18:10-5. [Crossref] [PubMed]
- Melzack R, Wall PD. Pain mechanisms: a new theory. Science 1965;150:971-9. [Crossref] [PubMed]
- Wang CJ, Wang FS, Yang KD, et al. Shock wave therapy induces neovascularization at the tendon-bone junction. A study in rabbits J Orthop Res 2003;21:984-9. [Crossref] [PubMed]
- Wang HJ, Cheng JH, Chuang YC. Potential applications of low-energy shock waves in functional urology. Int J Urol 2017;24:573-581. [Crossref] [PubMed]
- Fischer S, Mueller W, Schulte M, et al. Multiple extracorporeal shock wave therapy degrades capsular fibrosis after insertion of silicone implants. Ultrasound Med Biol 2015;41:781-9. [Crossref] [PubMed]
- Jaalouk DE, Lammerding J. Mechanotransduction gone awry. Nat Rev Mol Cell Biol 2009;10:63-73. [Crossref] [PubMed]
- Weihs AM, Fuchs C, Teuschl AH, et al. Shock wave treatment enhances cell proliferation and improves wound healing by ATP release-coupled extracellular signal-regulated kinase (ERK) activation. J Biol Chem 2014;289:27090-104. [Crossref] [PubMed]
- Xu JK, Chen HJ, Li XD, et al. Optimal intensity shock wave promotes the adhesion and migration of rat osteoblasts via integrin β1-mediated expression of phosphorylated focal adhesion kinase. J Biol Chem 2012;287:26200-12. [Crossref] [PubMed]
- Zhang J, Kang N, Yu X, et al. Radial Extracorporeal Shock Wave Therapy Enhances the Proliferation and Differentiation of Neural Stem Cells by Notch, PI3K/AKT, and Wnt/β-catenin Signaling. Sci Rep 2017;7:15321. [Crossref] [PubMed]
- Wang B, Zhou J, Banie L, et al. Low-intensity extracorporeal shock wave therapy promotes myogenesis through PERK/ATF4 pathway. Neurourol Urodyn 2018;37:699-707. [Crossref] [PubMed]
- Zou ZJ, Liang JY, Liu ZH, et al. Low-intensity extracorporeal shock wave therapy for erectile dysfunction after radical prostatectomy: a review of preclinical studies. Int J Impot Res 2018;30:1-7. [Crossref] [PubMed]
- Wang B, Ning H, Reed-Maldonado AB, et al. Low-Intensity Extracorporeal Shock Wave Therapy Enhances Brain-Derived Neurotrophic Factor Expression through PERK/ATF4 Signaling Pathway. Int J Mol Sci 2017;18:433. [Crossref] [PubMed]
- Li H, Matheu MP, Sun F, et al. Low-energy Shock Wave Therapy Ameliorates Erectile Dysfunction in a Pelvic Neurovascular Injuries Rat Model. J Sex Med 2016;13:22-32. [Crossref] [PubMed]
- Yahata K, Kanno H, Ozawa H, et al. Low-energy extracorporeal shock wave therapy for promotion of vascular endothelial growth factor expression and angiogenesis and improvement of locomotor and sensory functions after spinal cord injury. J Neurosurg Spine 2016;25:745-55. [Crossref] [PubMed]
- Zhang X, Yan X, Wang C, et al. The dose-effect relationship in extracorporeal shock wave therapy: the optimal parameter for extracorporeal shock wave therapy. J Surg Res 2014;186:484-92. [Crossref] [PubMed]
(English Language Editors: C. Betlazar-Maseh and J. Chapnick)