Curcumin plays a protective role against septic acute kidney injury by regulating the TLR9 signaling pathway
Introduction
Acute kidney injury (AKI) is one of the most common and serious complications of sepsis patients in the intensive care unit (ICU). Patients often develop multiple organ failure, resulting in a mortality rate of 60–80% (1).
Toll-like receptors (TLRs) can recognize the nucleic acids of bacteria and viruses and trigger innate immune responses (2). MyD88 protein, an essential downstream adapter molecule in the mitochondrial activated protease and NF-κB signaling pathway, can activate most of the TLR signals (3). Studies have shown that MyD88-deficient rats have good resistance to the development of sepsis, but TLR2- and TLR4-deficient rats do not show resistance (4). TLR9 knockdown significantly inhibited renal cell apoptosis, alleviated the severity of sepsis induced acute kidney injury, and improved the survival rate of rat model (5).
Curcumin (Cur) is a dietary polyphenol extracted from the Zingiberaceae family of plants. Cur has been shown to have good clinical value in cancer (6), osteoarthritis (7), anxiety and depression (8), dyslipidemia (9), metabolic syndrome (10), respiratory diseases (11), nonalcoholic fatty liver (12), type 2 diabetes (13), and ulcerative colitis (14).
Although Cur has been studied in a variety of human disease models, its application in septic acute kidney injury (SAKI) has not been thoroughly explored. In this study, we used a Sprague-Dawley (SD) rat model of SAKI to explore the inhibitory effect of Cur on this disease from different angles. Our results preliminarily proved that Cur exerts its inhibitory effect on SAKI by inhibiting the TLR9 signaling pathway, which provides a theoretical basis for the clinical application of Cur in SAKI.
We present the following article in accordance with the ARRIVE reporting checklist (available at http://dx.doi.org/10.21037/tau-21-385).
Methods
Experimental materials and instruments
Specific pathogen-free (SPF) SD rats (32–40 weeks old) were purchased from Shanghai Xipuer-Bikai Laboratory Animal Co., Ltd. Cur was purchased from SIGMA Biological Company, USA (batch No. 08511). The Cur powder was dissolved in normal saline to prepare a 400 mg/mL solution, which was then filtered by a 0.22 um sterile filter membrane and stored at −20 °C for later use. Purchased from InvivoGene (France), the TLR9-inhibitor and inhibitor-control drugs (batch Nos. ODN 2088 and ODN 2088 control) were prepared according to the method for Cur and stored after filtration. Isoflurane was purchased from Baxter Caribe company, USA (batch No. N094M209). The NF-κB–specific ELISA kit was purchased from Abcam Co., USA (batch No. ab176663). ELISA kits specific for tumor necrosis factor (TNF)-α, interleukin
The 5424R high-speed refrigerated microcentrifuge was purchased from Thermo Fisher Scientific, USA (Model 917), the automatic biochemical analyzer from Hitachi, Japan, and the Model 680 full-wavelength microplate reader from Bio-Rad Company, USA.
Survival rate assessment experiment
Experiments were performed in compliance with Qiqihar Medical University guidelines for the care and use of animals. The study was approved by the Qiqihar Medical University Institutional Review Board (No. QMU-AECC-2018-32), Heilongjiang, China. Twenty-four SPF SD rats in the same batch were randomized into groups A, B, and C, with eight rats in each group. Group A (curcumin group) underwent cecal ligation perforation (CLP). The rats were given Cur orally (40 mg/kg) 6 hours after the operation. Group B (placebo group) was given the same dose of normal saline 6 hours after CLP. Group C (sham group) underwent a sham operation. The experimental animals were observed 24, 48, 72, and 96 hours after treatment for survival status, and were euthanized when they became seriously ill.
The CLP procedure was as follows: After isoflurane anesthesia and laparotomy, 4-0 ligation silk thread was fastened 15 mm away from the cecal tip of the SD rats. Then, the cecum was punctured twice with a 21-gauge needle, and a small amount of fecal excretion was gently extruded and inserted into the center of the abdominal cavity. At the end of the operation, 20 mL/kg of normal saline was injected subcutaneously. For the sham group, CLP was not performed, but the other procedures remained unchanged. After surgery, the abdominal incision was sutured with 6-0 nylon thread (15).
Animal model index detection
Survival rate evaluation experiments were conducted, then blood samples (1.0 mL) and urine samples (0.5 mL) were collected from the tail veins of the animals after 24 hours. After the blood samples were left at room temperature for 20 minutes, the blood and urine samples were centrifuged at 2,000 rpm/min for 20 minutes to collect the serum and urine supernatants, which were then packaged and frozen at −20 °C for the following experiments (16).
Evaluation of renal function injury
The levels of urea nitrogen (UN), creatinine (Cr), and the expression of inflammatory protein NF-κB in the collected animal serum samples were detected to evaluate the degree of kidney injury in the different treatment groups.
- UN detection: a Hitachi 917 automatic biochemical analyzer was used to detect the amount of UN in the collected serum samples.
- Cr detection: the detection of Cr in the serum was determined by the picric acid-based colorimetric kinetic assay using the Astra 8 autoanalyzer.
- NF-κB protein detection: the protein expression level of NF-κB in the serum was detected by an ELISA kit from Bio-Rad, USA (450 ELISA).
Evaluation of functional impairment of other organs
The changes in alanine aminotransferase (ALT), aspartate aminotransferase (AST), amylase, creatine kinase (CK), and lactate dehydrogenase (LDH) in the serum samples were detected to evaluate the functional impairment of other organs in the different treatment groups. A Hitachi 917 automatic biochemical analyzer was utilized to measure the indexes in the collected serum samples.
The degree of immune reaction in the different treatment groups
The changes in TNF-α and IL-10 in the serum samples were detected to evaluate the changes in inflammatory factors. The detection was carried out by TNF-α- and IL-10-specific ELISA kits.
Change trend of AKI markers
The molecular expression levels of NGAL, KIM-1, CysC, and other markers in the urine samples were detected to provide a reference for the clinical development of AKI. NGAL-, Kim-1- and CysC-specific ELISA kits were used for the detection.
Detection of TLR9 expression
By detecting TLR9 expression in the serum samples, the activation degree of the TLR9 signaling pathway in the development of SAKI was preliminarily determined. A TLR9-specific ELISA kit was used for the detection.
Mechanism exploration
Six hours after CLP, the SD rats were randomly assigned into three groups, which were given equal doses of Cur (40 mg/kg), TLR9 inhibitor (ODN 2088), and the control drug (ODN 2088 Control), respectively. After 24 hours, serum UN, Cr, ALT, AST, amylase, and CK levels were determined according to the above experimental protocol.
Meanwhile, MyD88, IRF5, and IRF7 levels in the collected serum samples were detected by MyD88-, IRF5- and IRF7-specific ELISA kits.
All tests were conducted in strict accordance with the manufacturers’ instructions.
Statistic analysis
GraphPad Prism 7.0 software was used for the data processing, and all statistical data were expressed as the mean ± standard error (mean ± SE). Differences between the experimental groups were analyzed by multiple comparisons and correction analysis of variance or t-tests for statistical significance. The level of significance was taken as P<0.05.
Results
Cur significantly reduces SAKI-induced mortality
A significantly improved survival rate was observed in rats who were treated with Cur 6 hours after CLP. Compared with 80% of the rats in the curcumin group, only 40% of the rats in the placebo group survived 96 hours after the operation (Figure 1).
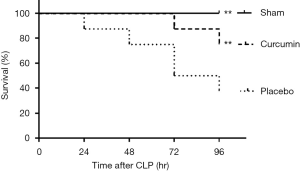
Cur is beneficial in alleviating SAKI-induced kidney damage
To explore the protective effect of Cur on the kidneys, we tested the related factors. The levels of UN, Cr, and NF-κB in the serum of the SD rats in the curcumin and sham groups were found to be significantly lower than those in the placebo group (P<0.05), indicating that Cur treatment 6 hours after surgery can effectively alleviate kidney injury caused by sepsis (Figure 2).
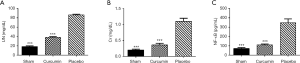
Cur has a good protective effect on many organs
The levels of ALT, AST, amylase, CK, and LDH in the serum samples of the curcumin and sham groups were significantly lower than those in the placebo group (P<0.05, Figure 3), These results indicate that Cur also has a good protective effect on a variety of organs other than the kidney..
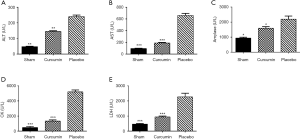
Cur downregulates the inflammatory response induced by SAKI
The medical community generally believes that a continuously activated immune response is the major cause of renal function damage in patients with SAKI (17). In the SD rat model of SAKI, We found significant increases in serum levels of pro-inflammatory cytokines TNF-α and IL-10 in the placebo group (P<0.05), which is consistent with the phenomenon reported in clinical samples, while the levels of TNF-α and IL-10 in curcumin treatment group and sham operation group were significantly lower than those in placebo group (P<0.05, Figure 4). This indicated that the progression of SAKI may be related to the over-continuous activation of an immune response in patients.
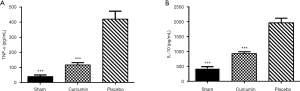
Cur inhibits the expression profiles of related markers during SAKI progression
According to previous studies, NGAL, serum CysC, and KIM-1 can be used as accurate and reliable markers for the early diagnosis of SAKI (18), so Cur may change the molecular expression level of these markers during SAKI progression. The levels of NGAL, CysC, and KIM-1 in the serum samples of the placebo group were significantly higher than those in the sham group (P<0.05). These parameters were significantly lower in the curcumin group compared with the placebo group (P<0.05, Figure 5), validating that NGAL, CysC, and KIM-1 can be used to judge the progression of SAKI. The therapeutic effect of Cur on this disease can also be evaluated by these markers.

Cur inhibits TLR9 upregulation induced by SAKI
Studies have shown that the occurrence of septic acute kidney injury leads to significant up-regulation of TLR9 (19). Cur, as a vital inhibitor of TLR (20), may exert its therapeutic effect on SAKI by inhibiting the TLR9 signaling pathway. In this study, the level of TLR9 in the serum samples from the placebo group was significantly higher than that in the sham and curcumin groups (P<0.05, Figure 6), which provides preliminary confirmation of our hypothesis.
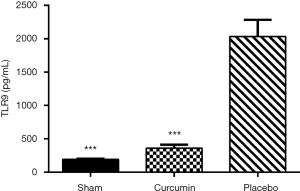
Cur exerts its therapeutic effect on SAKI by inhibiting the TLR9 signaling pathway
The levels of UN, Cr, ALT, AST, amylase, CK, LDH, NGAL, KIM-1, CysC, and other disease-related factors in the curcumin group and TLR9-inhibitor group were significantly lower than those in the control inhibitor group (P<0.05, Figure 7). In addition, the expression levels of the MyD88, IRF5, and IRF7 downstream molecules of the TLR9 signaling pathway in the curcumin group were found to be similar to those in the TLR9-inhibitor group and were significantly lower than those in the control-inhibitor group (P<0.05, Figure 8). The results indicated that Cur exerted its therapeutic effect on SAKI by inhibiting the TLR9 signaling pathway.
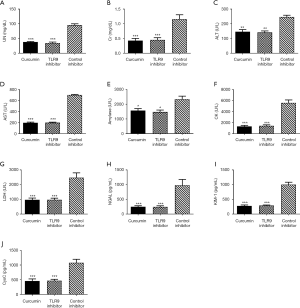
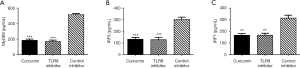
Discussion
Sepsis-induced AKI (SAKI) has been reported to be caused by major surgery, heart failure, respiratory failure, hypovolemia, and tissue hypoperfusion (21). According to recent studies, microcirculatory dysfunction, inflammation, and bio-energetic adaptive response to injury are also contribute to SAKI pathogenesis (22). Due to the insufficient understanding of the pathophysiology of SAKI, there is no guaranteed treatment for SAKI. Based on the mechanisms of SAKI we have got, fluid resuscitation, systematic resuscitation have been used clinically, but the most common therapeutic protocol was Renal replacement therapy (RRT), while the best timing to implement is hard to control (23), thus we should go deeper to explore the mechanism of SAKI.
According to the existing literature, Cur has anti-infective properties against various human pathogens, such as the influenza virus, hepatitis C virus, and HIV. It can also reduce kidney damage associated with rhabdomyolysis (24). Of note, the most promising application potential is its anti-inflammatory effect, and many clinical studies have evaluated its biological activity under various inflammatory conditions. These clinical trial data not only show the actual therapeutic effect of curcumin on some specific diseases, but also indicate that Cur has considerable safety and tolerability in human body, which provides very valuable experience for clinical trials of Cur in SAKI.
In clinical sample reports, SAKI often induces injury to multiple organs, such as the lungs, heart, brain, liver, and kidneys (25). By detecting the concentration changes of related factors in our rat model, we found that SAKI caused damage to the kidneys and other multiple organs. This indicates that the animal model simulates the development of SAKI in human body to a certain extent, and provides a reliable theoretical basis for future research and clinical drug experiments. Besides, we found that Cur significantly reduced the incidence of multiple organ damage. Therefore, we speculate that Cur may play an important role in balancing the immune response of the body.
Previous studies have shown that Cur can significantly downregulate the expression level of inflammatory factors such as TNF-α, IL-1, IL-2, IL-6, IL-8, and mitogen-activated protein kinase (MAPK) (26). Cur can also inhibit the activation of the extracellular TLR2/4 signaling pathway and can suppress the activation of IRAK1, IRAK6, TRIF, and MyD88 downstream of the TLR2/4 signaling pathway. In addition, Cur has been proved to be one of the antagonists of the intracellular TLR9 signaling pathway (27). The change in TLR9 expression is usually considered to be a sign of the development of various inflammation-related diseases, while Cur can reduce TLR9 expression in the rat liver and act as an anti-inflammatory and immunomodulatory agent to treat liver inflammation (28). The most recent study has revealed that Cur could ameliorate the SAKI induced by CLP, but they only detected the kidney protective effect of the Cur (29). While in this paper, we have found that the Cur could significantly decrease the mortality caused by AKI, and we also determined that Cur could prevent multiple organs from dysfunction caused by AKI, meanwhile, our results were almost based on the in vivo data, which may be more coincidence with the pathogenesis of AKI in human.
Conclusions
In this study, we found that the expression of pro-inflammatory factors, which rose significantly in rats with SAKI, can be counteracted by Cur. Also, the activation of the TLR9 molecule was detected in the SAKI rat model, but not in the curcumin group or the control-inhibitor group. The relevant results of this study showed that Cur, while playing its role in the resistance to SAKI, indeed inhibited the expression of downstream molecules in the TLR9 signaling pathway. Based on the above results, Cur can inhibit SAKI by inhibiting the activation of the TLR9 signaling pathway.
Acknowledgments
Funding: This study was funded by the Clinical Research Fund Project of Qiqihar Academy of Medical Sciences (QMSI2019L-10).
Footnote
Reporting Checklist: The authors have completed the ARRIVE reporting checklist. Available at http://dx.doi.org/10.21037/tau-21-385
Data Sharing Statement: Available at http://dx.doi.org/10.21037/tau-21-385
Conflicts of Interest: All authors have completed the ICMJE uniform disclosure form (available at http://dx.doi.org/10.21037/tau-21-385). The authors have no conflicts of interest to declare.
Ethical Statement: The authors are accountable for all aspects of the work in ensuring that questions related to the accuracy or integrity of any part of the work are appropriately investigated and resolved. Experiments were performed in compliance with Qiqihar Medical University guidelines for the care and use of animals. The study was approved by the Qiqihar Medical University Institutional Review Board (No. QMU-AECC-2018-32), Heilongjiang, China.
Open Access Statement: This is an Open Access article distributed in accordance with the Creative Commons Attribution-NonCommercial-NoDerivs 4.0 International License (CC BY-NC-ND 4.0), which permits the non-commercial replication and distribution of the article with the strict proviso that no changes or edits are made and the original work is properly cited (including links to both the formal publication through the relevant DOI and the license). See: https://creativecommons.org/licenses/by-nc-nd/4.0/.
References
- Sun J, Zhang J, Tian J, et al. Mitochondria in Sepsis-Induced AKI. J Am Soc Nephrol 2019;30:1151-61. [Crossref] [PubMed]
- Diakowska D, Nienartowicz M, Grabowski K, et al. Toll-like receptors TLR-2, TLR-4, TLR-7, and TLR-9 in tumor tissue and serum of the patients with esophageal squamous cell carcinoma and gastro-esophageal junction cancer. Adv Clin Exp Med 2019;28:515-22. [Crossref] [PubMed]
- Balka KR, De Nardo D. Understanding early TLR signaling through the Myddosome. J Leukoc Biol 2019;105:339-51. [Crossref] [PubMed]
- Xia S, Lin H, Liu H, et al. Honokiol Attenuates Sepsis-Associated Acute Kidney Injury via the Inhibition of Oxidative Stress and Inflammation. Inflammation 2019;42:826-34. [Crossref] [PubMed]
- Fanelli C, Arias SCA, Machado FG, et al. Innate And Adaptive Immunity are Progressively Activated in Parallel with Renal Injury in the 5/6 Renal Ablation Model. Sci Rep 2017;7:3192. [Crossref] [PubMed]
- Mirzaei H, Shakeri A, Rashidi B, et al. Phytosomal curcumin: A review of pharmacokinetic, experimental and clinical studies. Biomed Pharmacother 2017;85:102-12. [Crossref] [PubMed]
- Asadi S, Gholami MS, Siassi F, et al. Beneficial effects of nano-curcumin supplement on depression and anxiety in diabetic patients with peripheral neuropathy: A randomized, double-blind, placebo-controlled clinical trial. Phytother Res 2020;34:896-903. [Crossref] [PubMed]
- Qin S, Huang L, Gong J, et al. Efficacy and safety of turmeric and curcumin in lowering blood lipid levels in patients with cardiovascular risk factors: a meta-analysis of randomized controlled trials. Nutr J 2017;16:68. [Crossref] [PubMed]
- Azhdari M, Karandish M, Mansoori A. Metabolic benefits of curcumin supplementation in patients with metabolic syndrome: A systematic review and meta-analysis of randomized controlled trials. Phytother Res 2019;33:1289-301. [Crossref] [PubMed]
- Salehi B, Stojanović-Radić Z, Matejić J, et al. The therapeutic potential of curcumin: A review of clinical trials. Eur J Med Chem 2019;163:527-45. [Crossref] [PubMed]
- Lee DE, Lee SJ, Kim SJ, et al. Curcumin Ameliorates Nonalcoholic Fatty Liver Disease through Inhibition of O-GlcNAcylation. Nutrients 2019;11:2702. [Crossref] [PubMed]
- Wojcik M, Krawczyk M, Wojcik P, et al. Molecular Mechanisms Underlying Curcumin-Mediated Therapeutic Effects in Type 2 Diabetes and Cancer. Oxid Med Cell Longev 2018;2018:9698258 [Crossref] [PubMed]
- Cunha Neto F, Marton LT, de Marqui SV, et al. Curcuminoids from Curcuma Longa: New adjuvants for the treatment of crohn's disease and ulcerative colitis? Crit Rev Food Sci Nutr 2019;59:2136-43. [Crossref] [PubMed]
- Momtazi-Borojeni AA, Haftcheshmeh SM, Esmaeili SA, et al. Curcumin: A natural modulator of immune cells in systemic lupus erythematosus. Autoimmun Rev 2018;17:125-135. [Crossref] [PubMed]
- Post EH, Kellum JA, Bellomo R, et al. Renal perfusion in sepsis: from macro-to microcirculation. Kidney Int 2017;91:45-60. [Crossref] [PubMed]
- Yasuda H, Leelahavanichkul A, Tsunoda S, et al. Chloroquine and inhibition of Toll-like receptor 9 protect from sepsis-induced acute kidney injury. Am J Physiol Renal Physiol 2008;294:F1050-F1058. [Crossref] [PubMed]
- Jang HR, Rabb H. Immune cells in experimental acute kidney injury. Nat Rev Nephrol 2015;11:88. [Crossref] [PubMed]
- Assadi F, Sharbaf FG. Urine KIM-1 as a potential biomarker of acute renal injury after circulatory collapse in children. Pediatr Emerg Care 2019;35:104-7. [Crossref] [PubMed]
- Liu L, Li Y, Hu Z, et al. Small interfering RNA targeting Toll-like receptor 9 protects mice against polymicrobial septic acute kidney injury. Nephron Exp Nephrol 2012;122:51-61. [Crossref] [PubMed]
- Boozari M, Butler AE, Sahebkar A. Impact of curcumin on toll-like receptors. J Cell Physiol 2019;234:12471-82. [Crossref] [PubMed]
- Zarbock A, Gomez H, Kellum JA. Sepsis-induced acute kidney injury revisited: pathophysiology, prevention and future therapies. Curr Opin Crit Care 2014;20:588-95. [Crossref] [PubMed]
- Zhang Z. Biomarkers, diagnosis and management of sepsis-induced acute kidney injury: a narrative review. Heart Lung Vessel 2015;7:64-73. [PubMed]
- Bagshaw SM, Wald R, Adhikari NKJ, et al. Timing of Initiation of Renal-Replacement Therapy in Acute Kidney Injury. N Engl J Med 2020;383:240-51. [Crossref] [PubMed]
- Nelson KM, Dahlin JL, Bisson J, et al. The Essential Medicinal Chemistry of Curcumin. J Med Chem 2017;60:1620-37. [Crossref] [PubMed]
- Husain-Syed F, Rosner MH, Ronco C. Distant organ dysfunction in acute kidney injury. Acta Physiol (Oxf) 2020;228:e13357 [Crossref] [PubMed]
- Varma SR, Sivaprakasam TO, Mishra A, et al. Imiquimod-induced psoriasis-like inflammation in differentiated Human keratinocytes: Its evaluation using curcumin. Eur J Pharmacol 2017;813:33-41. [Crossref] [PubMed]
- Dai J, Gu L, Su Y, et al. Inhibition of curcumin on influenza A virus infection and influenzal pneumonia via oxidative stress, TLR2/4, p38/JNK MAPK and NF-κB pathways. Int Immunopharmacol 2018;54:177-87. [Crossref] [PubMed]
- She L, Xu D, Wang Z, et al. Curcumin inhibits hepatic stellate cell activation via suppression of succinate-associated HIF-1α induction. Mol Cell Endocrinol 2018;476:129-38. [Crossref] [PubMed]
- Zhu H, Wang X, Wang X, et al. Curcumin attenuates inflammation and cell apoptosis through regulating NF-κB and JAK2/STAT3 signaling pathway against acute kidney injury. Cell Cycle 2020;19:1941-51. [Crossref] [PubMed]
(English Language Editor: D. Fitzgerald)