Mediators of human ureteral smooth muscle contraction—a role for erythropoietin, tamsulosin and Gli effectors
Introduction
Ureteral peristalsis is the process by which urine is propelled from the kidney to the bladder, and is generated secondary to contraction of the smooth muscles in the wall of the ureter (1). The mechanisms underlying the propagation of a peristaltic wave in the ureter are not well understood. Isolated ureteral tissue specimens have previously been shown to have spontaneous contractile activity in in vitro experiments (2). This implies that the propagation of peristaltic contractions is largely controlled by the smooth muscle cells (SMCs) of the ureteral wall. The contractile ability of ureteral smooth muscle cells is critical for allowing movement of urine from the kidney to the bladder. Peristalsis is important in phenomena as varied as stone passage and congenital disorders such as megaureter and ureteropelvic junction obstruction (3). Our group has recently shown that the recovery of ureteral peristalsis following unilateral ureteral obstruction reversal was delayed by 8 days following resolution of hydronephrosis and 11 days following obstruction reversal (4). Given the significant negative effects that disrupted ureteral peristalsis due to obstruction or congenital issues has on kidney function, health and the morbidity of the patient, there exists a need to improve our understanding of the major molecular players in this process to identify potential targets for future therapeutic agents designed to accelerate the recovery of peristalsis following surgical interventions for stones or congenital disorders.
Electromechanical coupling in the ureter has previously been investigated (5). The general mechanism of smooth muscle contraction is known to involve the action of calcium working to activate myosin within myocytes (6). The propagation of contractions by ureteral SMCs are thought to involve the action of calcium and calcium ion channels (7), a theory supported by experiments using the calcium channel blocker nifedipine (8), which has successfully been used in the management of renal colic by decreasing peristalsis (9).
In this study, we aimed to begin expanding our knowledge regarding mechanisms that drive force generation by ureteral smooth muscle by investigating the role of three putative protein regulators in the peristaltic activity of human ureteral tissue based on previous studies in the literature. The first protein, the alpha-1 adrenergic receptor (α1-AR) has long been known to play a role in this process however actual mechanistic studies are scarce. Furthermore, studies in our lab and others have suggested a role for erythropoietin (EPO) and Gli proteins in mediating ureteral contractions (4,10,11).
Tamsulosin is an α1-AR antagonist that is commonly prescribed to patients with acute kidney stone episodes. Recent prospective studies have cast doubt on its benefit in medical expulsive therapy (12). However, there is evidence to support the contention that alpha blockers are effective in the expulsion of distal ureteral calculi (13,14). While it is expected to work via blockade of α1-AR, its exact mechanism of action is not clear.
Erythropoietin has been demonstrated to play a role in peristaltic activity of the intestinal smooth muscle (15,16). Erythropoietin receptors are known to populate the kidney (17) and we have recently demonstrated EPO expression in murine ureters (4). While the non-hematopoietic mechanism of action of EPO is currently unclear, we contend that it may work in a similar fashion in ureteral and intestinal smooth muscle cells. Thus, we hypothesize that exposure to EPO will modulate peristaltic activity in normal human ureter.
Lastly, Gli family proteins are the downstream effectors of the Sonic Hedgehog-Gli pathway known to play a role in fetal tissue patterning (18). Other work has revealed hydronephrosis, hydroureter and ureteral dyskinesia in mice with Gli3 mutations (19). Our group has previously found a potential role for Gli1 in adult porcine ureteral peristalsis after ureteral stent implantation, which forms a partial obstruction (20). Here, we investigate the effect of Gli proteins, EPO and tamsulosin on the contractile ability of distal human ureteral tissue to better understand their roles in mediating smooth muscle contraction and peristalsis. Collectively the studies presented here are designed to take knowledge gathered from previous studies in our laboratory and others, implicating a role for EPO, Gli and α1-AR’s in mediating ureteral peristalsis, and investigating their role on force production by ureteral smooth muscle and peristalsis. We present the following article in accordance with the MDAR reporting checklist (available at https://dx.doi.org/10.21037/tau-20-1342).
Methods
Electrical field stimulation
The study was conducted in accordance with the Declaration of Helsinki (as revised in 2013). The study was approved by Institutional Clinical Research Ethics Board (NO.: H16-01561) and informed consent was taken from all the patients to procure portions of extraneous distal ureteral tissue from living donor renal transplants. Samples were de-identified prior to release and no data pertaining to patients or donors was obtained or stored, as patient information was not required for the purposes of this study. In total, experiments were carried out on extraneous tissue from 26 different healthy living donors between the ages of 18–65. The tissues were immediately placed in Krebs buffer kept at 4C adjacent to the operating room then transported in an ice-cold temperature maintaining container. The experiments were performed within 24 hours of obtaining the tissue. Ureteral tissue contractility was assessed in an electrical field stimulation (EFS) device designed specifically for the evaluation of smooth muscle contraction Figure 1). Each treatment was tested in three separate experiments containing different biological samples, with each parameter being run a minimum three times. Tissue was defatted and prepared in approximately 1 mm thick rings mounted vertically in a tissue bath with one end fixed to a stationary hook and the other to a servo-controlled lever of a length-force transducer. This was done so that the tissue would be parallel to the electrodes (Figure 1). The tissue bath contained Krebs buffer solution at 37 °C that was bubbled with a 95% O2-5% CO2 mixture. The Krebs solution composition was: 118 mM NaCl, 4 mM KCl, 1.2 mM NaH2PO4, 22.5 mM NaHCO3, 2 mM MgSO4, 2 mM CaCl2, and 2 g/L dextrose at pH 7.4. The ureteral tissue was maintained at the in situ length with minimal passive resting tension (10,21). The muscle preparation was equilibrated for one hour. Muscle was then activated by EFS. A uniform electric current was achieved using a 60-Hz AC stimulator with platinum electrodes. An isometric contraction was elicited after each stretch. The classic length-tension relationship of the muscle was characterized and contractile force was recorded with each stimulation with and without the presence of a Gli inhibitor GANT61 (Sigma-Aldrich, St. Louis, MO) at 20 μM in DMSO solvent (Sigma-Aldrich, St. Louis, MO), 20 IU human biosynthetic epoetin alphaEPO (Eprex 2,000 IU/0.5 mL, Janssen Inc, Beerse, Belgium), 2 mM calcium chloride (Sigma-Aldrich, St. Louis, MO), or generic tamsulosin 1 μM in DMSO solvent. DMSO has previously been used as a solvent in similar studies (22,23). Control tissue in GANT61 and tamsulosin experiments was subjected to DMSO.
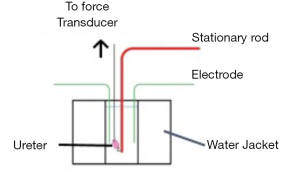
Immunohistochemistry
Each ureteral specimen was subsequently fixed in 10% formalin and embedded in paraffin. Sections of 5 µm thickness were cut, dewaxed in xylene and an alcohol series then washed in Tris-buffered saline. Antigen retrieval was undertaken with citrate buffer and endogenous peroxidase was blocked. Primary antibody was incubated overnight at 4C. Primary antibodies: alpha-smooth muscle actin (dilution 0.13 µg/mL), Gli 1 antibody NBP2-45872 dilution 6.67 µg/mL (Novus Biologicals, Abingdon, UK), EPO receptor antibody sc-365662 dilution 4 µg/mL (Santa Cruz Biotechnology, Dallas, TX), alpha 1 adrenergic receptor antibody dilution 2.5 µg/mL antibody PA5-33327 (Invitrogen, Carlsbad, CA, USA). After washes, sections were incubated with the appropriate secondary antibody for 30 minutes at room temperature. Image capture was performed using the Leica multistainer scanner (Leica Biosystems, Wetzlar, Germany) and protein quantitation was achieved with the Aperio ImageScope software version 12.3.3.5048 (Leica Biosystems, Wetzlar, Germany). Protein quantitation was performed by analyzing the whole section.
Statistical analysis
Statistical significance was determined using an unpaired t-test with significance set at P<0.05 (two-tailed).
Results
Distal ureteral tissue harvested from living donor kidney transplants were subjected to electric field stimulation. Administration of additional calcium salt to the tissue bath resulted in increased contractile force (Figure 2, P=0.043). Tissue subjected to the Gli protein inhibitor GANT61 demonstrated decreased contractility compared to control tissue (Figure 3, P=0.039). Contractile force differences were noted at one hour of incubation.
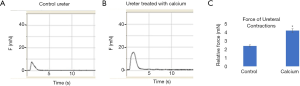
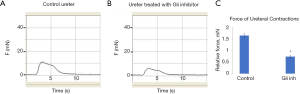
Addition of EPO resulted in decreased ureteral contractility compared to controls (Figure 4, P=0.042). This was noted at five minutes of incubation time. Similarly, tamsulosin resulted in decreased contractility (Figure 5, P=0.048) but did not completely retard contractions. This was noted within one hour of administration.
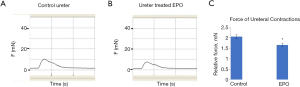
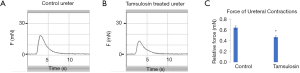
Immunohistochemistry (IHC) was performed to investigate whether contractile force changes correlated with differences in expression of relevant proteins. IHC analysis demonstrated a non-significant increase in Gli1 expression in ureteral tissue subjected to GANT61 (Figure 6A,B,C). Similarly, EPO receptor protein levels were increased compared to control on administration of erythropoietin (Figure 6 D,E,F) but this was not significant. Expression was primarily located in the epithelial layer. Protein expression of α1-AR was unchanged following tamsulosin administration as compared to control ureteral tissue (Figure 6 G,H,I).
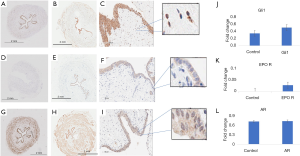
Discussion
The ureters serve a vital function in the urinary system, as they propel urine from the kidneys to the bladder for storage until urination. Partial or complete obstruction results in ureteral dysfunction, manifesting as the cessation of peristaltic activity and urine transport from the kidney on the affected side to the bladder. Previous work has shown that the return of peristalsis to pre-obstruction levels is significantly delayed following obstruction reversal and resolution of hydronephrosis, suggesting that negative effects on urine drainage and possibly kidney function are prolonged (4). An improved understanding of mechanisms that drive ureteral peristalsis as well as those elicited by currently used pharmacological approaches to manipulate ureteral function is required for the development of more effective approaches to regulate ureteral peristalsis pre- and/or post-operatively. The present study was undertaken as an attempt to add to our understanding of this process, by assessing the effect of currently used interventions and possible alternatives identified through previous work, on smooth muscle function of human ureters.
The finding that distal ureteral contractility was found to increase with the administration of calcium supports previous data by other groups indicating that calcium signaling mediates peristalsis. The ionic basis of the electrical activity of the ureter is incompletely understood but is thought to involve L-type calcium channels (24). This SMC excitation likely couples to contraction, with the electrical activity being propagated in a cell to cell manner with the subsequent contraction moving distally to otherwise inactive SMCs in the ureter. This movement creates a contractile wave and urine is propelled down the ureter from the kidney to the bladder for micturition (6). The administration of calcium in this study served as a positive control to ensure that the tissue is viable and able to respond to stimuli that are naturally known to trigger contraction.
Tamsulosin is an α1D-AR inhibitor whose role in renal colic management is thought to be a result of its α1 blockade. Here, we have shown that tamsulosin does indeed decrease the force of ureteral contraction in human ureteral tissue (Figure 5). This is consistent with work by Weiss et al. showing a similar mechanism of action of phentolamine (24). Additionally, a previous study by Troxel et al. investigating the effect of tamsulosin on pig ureters found that it decreased the amplitude of the contraction and increased contraction intervals in porcine ureters. In the current study, tamsulosin administration had no significant effect on the duration of contractions (data not shown). We propose that it is by decreasing the contractile activity of ureteral SMCs without completely eliminating ureteral contractions, that tamsulosin acts to relax ureteral smooth muscle. This relaxation likely allows for the distal progress of ureteral stones. Our work here attempting to investigate whether there were changes in the expression of α1-AR based on tamsulosin administration showed no significant change in α1-AR protein levels in ureteral tissue subjected to tamsulosin versus control, indicating that the decrease in contraction is not mediated at the protein level. The α1D-AR expression was intracellular, as previously demonstrated (25). Further work is necessary to investigate α1-AR expression in obstructed ureters in order to further expand our understanding of the function of tamsulosin in this process.
EPO has previously been demonstrated to improve intestinal dysmotility by increasing the force of contraction in response to acetyl choline administration (15). Our group has shown a similar improvement of ureteral motility following EPO administration in a mouse model of ureteral obstruction (4). In this study using non-obstructed ureteral tissue, we demonstrate that EPO decreases ureteral contraction force in “normal” uninjured human ureteral tissue. The fact that an effect by EPO is seen in both obstructed and unobstructed tissues is highly suggestive of its role in regulating general ureteral contractility, further suggesting a potential for targeting EPO-induced signalling for possible intervention.
EPO was noted to decrease contractility within five minutes of administration, suggesting that its regulation of ureteral contractility occurs via a fast-acting mechanism. A novel finding of this work is the expression of the EPO receptor in the epithelium of human distal ureter. On IHC, there is a trend towards increased expression of the EPO receptor versus control tissue suggesting that the mechanism of action may utilize this receptor. EPO receptor expression was noted at the cell membrane and intracellularly, consistent with its role as a transmembrane receptor with an intracellular domain (26). To our knowledge, such rapid activation of non-hematopoietic EPO activity has not previously been demonstrated to involve EPO receptor changes. It is interesting that EPO is identified primarily in the epithelium, while affecting ureteral contraction. There is some evidence to suggest that in addition to its activity via the traditional EPO receptor, the EPO protein also utilizes the beta-common receptor and cation channel receptors for some of its functions (27,28). Others have shown that erythropoietin bioactivity is associated with downstream effects in non-epithelial tissues (29). We suggest that this may be the case in the human ureter. Further work will be done to characterize the mechanism underlying its activity in ureteral tissue.
In the present study, administration of the Gli inhibitor GANT61 led to a decrease in contractile force of ureteral tissue (Figure 3). This is consistent with the hypothesis that Hedgehog signaling may play a role in mediating ureteral contractility. IHC analysis of Gli1 protein in this ureteral tissue demonstrated that Gli1 is expressed in adult human ureters. There did not appear to be a significant difference in Gli1 levels after administration of GANT61 as compared to controls (Figure 6). This may be partially explained by the mechanism of GANT61 activity which blocks the post-translational binding of Gli proteins to DNA rather than Gli1 protein expression (30). It is therefore not surprising that the level of Gli1 protein expression as determined using immunohistochemistry remains unchanged. Gli1 expression was intracellular, consistent with previous work (10).
The present study was undertaken as a critical first step to better understand the role that previously identified potential regulators of peristalsis affect smooth muscle contraction of human ureters. The expression of specific protein has been demonstrated to differ in different regions of the ureter (31). Since tissue was obtained from the distal ureters of living transplant donors, this is a limitation of the study. Overall, we have shown that EPO, Gli1 and the manipulation of α1-AR have an effect on human ureteral smooth muscle function. Further work must be done both in normal and obstructed ureters in order to fully elucidate the electrical and mechanical underpinnings of ureteral contractions and how EPO, Gli and α1-AR-associated signalling could be manipulated to promote recovery of normal ureteral peristalsis following surgical interventions to remove obstructive stones and/or to correct anatomic abnormalities that affect ureteral function.
Conclusions
Inhibitors of Gli protein and α1-AR decrease distal ureteral contractile forces. EPO decreased ureteral contractility within a short time frame, suggesting ion channel involvement instead of changes in gene expression. The effect of EPO on ureteral contraction may act at least in part via the EPO receptor. Ongoing work is aimed at clarifying the role of Gli proteins, EPO and α1-AR in regulating ureteral contractions.
Acknowledgments
he authors thank the urologists, residents and operating room nursing staff at St. Paul’s Hospital Vancouver for their assistance with collection of the specimens. Dr. Ralph Buttyan is also appreciated for his helpful discussions.
Funding: None.
Footnote
Reporting Checklist: The authors have completed the MDAR reporting checklist. Available at https://dx.doi.org/10.21037/tau-20-1342
Data Sharing Statement: Available at https://dx.doi.org/10.21037/tau-20-1342
Conflicts of Interest: All authors have completed the ICMJE uniform disclosure form (available at https://dx.doi.org/10.21037/tau-20-1342). The authors have no conflicts of interest to declare.
Ethical Statement: The authors are accountable for all aspects of the work in ensuring that questions related to the accuracy or integrity of any part of the work are appropriately investigated and resolved. The study was conducted in accordance with the Declaration of Helsinki (as revised in 2013). The study was approved by Institutional Clinical Research Ethics Board (NO.: H16-01561) and informed consent was taken from all the patients.
Open Access Statement: This is an Open Access article distributed in accordance with the Creative Commons Attribution-NonCommercial-NoDerivs 4.0 International License (CC BY-NC-ND 4.0), which permits the non-commercial replication and distribution of the article with the strict proviso that no changes or edits are made and the original work is properly cited (including links to both the formal publication through the relevant DOI and the license). See: https://creativecommons.org/licenses/by-nc-nd/4.0/.
References
- Zimskind PD, Mannes HA. Clinical aspects of ureteral peristalsis. Urol Clin North Am 1974;1:413-8. [PubMed]
- Osman F, Nadasy GL, Monos E, et al. A novel videomicroscopic technique for studying rat ureteral peristalsis in vivo. World J Urol 2009;27:265-70. [Crossref] [PubMed]
- Mendelsohn C. Functional obstruction: the renal pelvis rules. J Clin Invest 2004;113:957-9. [Crossref] [PubMed]
- Janssen C, Jager W, Moskalev I, et al. Erythropoietin accelerates the regeneration of ureteral function in a murine model of obstructive uropathy. J Urol 2015;193:714-21. [Crossref] [PubMed]
- Maggi CA, Giuliani S, Santicioli P. Effect of the Ca(2+)-ATPase inhibitor, cyclopiazonic acid, on electromechanical coupling in the guinea-pig ureter. Br J Pharmacol 1995;114:127-37. [Crossref] [PubMed]
- McHale N, Hollywood M, Sergeant G, et al. Origin of spontaneous rhythmicity in smooth muscle. J Physiol 2006;570:23-8. [Crossref] [PubMed]
- Imaizumi Y, Muraki K, Watanabe M. Characteristics of transient outward currents in single smooth muscle cells from the ureter of the guinea-pig. J Physiol 1990;427:301-24. [Crossref] [PubMed]
- Santicioli P, Carganico G, Meini S, et al. Modulation by stereoselective inhibition of cyclo-oxygenase of electromechanical coupling in the guinea-pig isolated renal pelvis. Br J Pharmacol 1995;114:1149-58. [Crossref] [PubMed]
- Troxel SA, Jones AW, Magliola L, et al. Physiologic effect of nifedipine and tamsulosin on contractility of distal ureter. J Endourol 2006;20:565-8. [Crossref] [PubMed]
- Janssen C, Buttyan R, Seow CY, et al. A Role for the Hedgehog Effector Gli1 in Mediating Stent-induced Ureteral Smooth Muscle Dysfunction and Aperistalsis. Urology 2017;104:242.e1-e8. [Crossref] [PubMed]
- Park E, Cox M, Scotland K, et al. Erythropoietin promotes functional recovery via anti-apoptotic mechanisms in mouse unilateral ureteral obstruction. Cell Stress Chaperones 2020;25:245-51. [Crossref] [PubMed]
- Wiseman OJ, McClinton S. Alpha blockers should no longer be used for ureteric stones. Int J Surg 2016;36:657-9. [Crossref] [PubMed]
- Bai Y, Yang Y, Wang X, et al. Tadalafil Facilitates the Distal Ureteral Stone Expulsion: A Meta-Analysis. J Endourol 2017;31:557-63. [Crossref] [PubMed]
- Tao RZ, Qin ZQ, Liu FD, et al. Efficacy and Safety of Tamsulosin in the Medical Expulsion Therapy for Distal Ureteral Calculi: A Systematic Review and Meta-Analysis of Placebo-Controlled Trials. Urol J 2019;16:224-31. [PubMed]
- Nagib EM, El-Sayed MH, Ahmed MA, et al. Intestinal motility in acute uremia and effects of erythropoietin. Saudi Med J 2012;33:500-7. [PubMed]
- Ozdamar A, Topcu K, Gumustekin M, et al. Erythropoietin restores bowel damage and hypoperistalsis in gastroschisis. J Pediatr Surg 2006;41:352-7. [Crossref] [PubMed]
- Sharples EJ, Yaqoob MM. Erythropoietin in experimental acute renal failure. Nephron Exp Nephrol 2006;104:e83-8. [Crossref] [PubMed]
- Hu MC, Mo R, Bhella S, et al. GLI3-dependent transcriptional repression of Gli1, Gli2 and kidney patterning genes disrupts renal morphogenesis. Development 2006;133:569-78. [Crossref] [PubMed]
- Cain JE, Islam E, Haxho F, et al. GLI3 repressor controls functional development of the mouse ureter. J Clin Invest 2011;121:1199-206. [Crossref] [PubMed]
- Huang Z-A, Scotland KB, Li Y, et al. Determination of urinary prostaglandin E2 as a potential biomarker of ureteral stent associated inflammation. J Chromatogr B Analyt Technol Biomed Life Sci 2020;1145:122107 [Crossref] [PubMed]
- Wang L, Chitano P, Pare PD, et al. Mechanopharmacology and Synergistic Relaxation of Airway Smooth Muscle. J Eng Sci Med Diagn Ther 2019;2:0110041-110047. [Crossref] [PubMed]
- Haddad L, Corriveau S, Rousseau E, et al. Impact of tamsulosin and nifedipine on contractility of pregnant rat ureters in vitro J Matern Fetal Neonatal Med 2018;31:191-6. [Crossref] [PubMed]
- Li J, Cai J, Zhao S, et al. GANT61, a GLI inhibitor, sensitizes glioma cells to the temozolomide treatment. J Exp Clin Cancer Res 2016;35:184. [Crossref] [PubMed]
- Weiss RM, Bassett AL, Hoffman BF. Adrenergic innervation of the ureter. Invest Urol 1978;16:123-7. [PubMed]
- Garcia-Cazarin ML, Smith JL, Clair DK, et al. The alpha1D-adrenergic receptor induces vascular smooth muscle apoptosis via a p53-dependent mechanism. Mol Pharmacol 2008;74:1000-7. [Crossref] [PubMed]
- Constantinescu SN, Keren T, Socolovsky M, et al. Ligand-independent oligomerization of cell-surface erythropoietin receptor is mediated by the transmembrane domain. Proc Natl Acad Sci U S A 2001;98:4379-84. [Crossref] [PubMed]
- Brines M, Grasso G, Fiordaliso F, et al. Erythropoietin mediates tissue protection through an erythropoietin and common beta-subunit heteroreceptor. Proc Natl Acad Sci U S A 2004;101:14907-12. [Crossref] [PubMed]
- Danielczok J, Hertz L, Ruppenthal S, et al. Does Erythropoietin Regulate TRPC Channels in Red Blood Cells? Cell Physiol Biochem 2017;41:1219-28. [Crossref] [PubMed]
- Juul SE, Ledbetter DJ, Joyce AE, et al. Erythropoietin acts as a trophic factor in neonatal rat intestine. Gut 2001;49:182-9. [Crossref] [PubMed]
- Zhang R, Wu J, Ferrandon S, et al. Targeting GLI by GANT61 involves mechanisms dependent on inhibition of both transcription and DNA licensing. Oncotarget 2016;7:80190-207. [Crossref] [PubMed]
- Malin JM Jr, Deane RF, Boyarsky S. Characterisation of adrenergic receptors in human ureter. Br J Urol 1970;42:171-4. [Crossref] [PubMed]