Development of a novel porcine ischemia/reperfusion model inducing different ischemia times in bilateral kidneys-effects of hydrogen gas inhalation
Introduction
Many animal models have been used for the research of renal ischemia reperfusion injury (IRI), a major condition of acute kidney injury. Renal IRI occurs in several situations represented by decreased circulating blood volume, circulatory arrest, or ischemia time during kidney transplantation. During renal IRI, damage-associated molecular patterns (DAMPs), including degraded DNA from necrotic tubular cells, stimulates the innate immune system and activates neutrophils, leading to NETosis and the further release of DNA and other DAMPs, causing a vicious cycle of necroinflammation (1). In addition, prolonged inflammation of macrophages, which recognize necrotic tubular cells and DAMPs, may lead to fibrosis and the development of chronic kidney disease (2,3).
Hydrogen gas is known to have anti-oxidative or anti-inflammatory effects, and its function as a reactive oxygen species (ROS) scavenger (4), platelet adhesion inhibitor (5), and inhibitor of IRI in several organs have been reported in several rodent models (6), although very few studies have been conducted on human-equivalent models. We have shown in a porcine kidney transplantation model that storage in hydrogen-containing perfusate improves the short-term function of ischemic kidney grafts (7) and inhibits long-term rejection (8). Based on these findings, we investigated whether hydrogen gas inhalation can reduce renal IRI and prevent long-term renal fibrosis using a porcine renal IRI model. However, experiments to confirm the long-term outcomes are very costly in terms of breeding and other expenses, and the risk of animal death during the observation periods due to IRI increases. Therefore, a model that can safely induce IRI and provide a large amount of data with a small number of experimental animals is needed.
Renal IRI in pig models has been induced in many ways, including cardiac arrest (induced ventricular fibrillation) (9), aortic occlusion (10), and renal vascular clamping (11,12). Simultaneous bilateral kidney ischemia or unilateral ischemia along with contralateral nephrectomy models only provide data on the renal response to a single ischemia time. Moreover, renal vascular clamping for 120 min or more, along with contralateral nephrectomy, causes irreversible damage to the kidney and increases mortality in animals (13).
In this study, we developed a novel porcine IRI model that allowed us to induce prolonged ischemia time for 120 min and subsequent reperfusion injury without threatening the lives of pigs and to observe different renal responses to two ischemia times from a single pig by subjecting the left and right kidneys to ischemia for 120 and 60 min, respectively. In this model, the release of DAMPs (DNA) from the kidneys immediately after IRI and subsequent long-term renal fibrosis increased in response to ischemia time. Using this novel model, we investigated whether hydrogen gas inhalation could alleviate IR kidney injury. We present the following article in accordance with the ARRIVE reporting checklist (available at https://tau.amegroups.com/article/view/10.21037/tau-21-1164/rc).
Methods
Experimental animals and ethics
The animal experiment was approved by the Jikei Medical University Animal Research Ethics Committee (approval No. 2021-014) and the Research Council and Animal Care and Use Committee of Keio University (approval No. 20005). The experiment was performed in the facility of Fuji Micra Inc. (Shizuoka, Japan). Animals were treated according to Guidelines for Proper Conduct of Animal Experiments, Science Council of Japan, 2006 (14). Four male micro-miniature pigs aged 24–32 months and weighing 26.5–29.0 kg were used. The pigs were housed in cages under temperature and light-controlled conditions (12-h light/dark cycle) and were provided with food and water ad libitum.
Anesthesia and euthanasia methods
The pigs were fasted for 12 h prior to surgery, with free access to water. Sedation with an intramuscular injection of xylazine (2.0 mg/kg) was followed by anesthetic induction with 5% inhalational isoflurane and 3.0 L/min oxygen. Anesthesia was maintained with 1–3% inhalational isoflurane. At the end of the observation period, phlebotomy from the inferior vena cava, under general anesthesia, was performed for euthanasia.
Experimental protocol
The experimental protocol is shown in Figure 1. After laparotomy via midline abdominal incision under general anesthesia, the parietal peritoneum was incised to expose the left renal artery and vein. The left renal artery and vein were clamped together to initiate a warm ischemia injury for 120 min. The right renal vessels were clamped, and the right kidney was exposed to warm ischemia injury 60 min after the left kidney. During this time difference between the onset of ischemia time in the left and right kidneys, pre-IRI venous blood samples were obtained directly from the inferior vena cava by puncturing with a 21 G needle. Hemostasis was achieved only by compression of the puncture point of the vessel wall. Arterial blood samples could also be withdrawn directly from the catheter inserted into the internal carotid artery or the abdominal aorta by puncturing with a 21 G needle in the same way. After warm ischemia time for 120 min in the left kidney and 60 min in the right kidney, both renal vessels were unclamped to induce reperfusion injury. Post-IRI venous blood samples from the left and right kidneys with different ischemia times were obtained separately by puncturing the left and right renal veins directly. After renal cortical wedge biopsy specimens were obtained, the surgical incision was closed. On day 7, renal cortical wedge biopsy and venous blood sampling from renal vein puncture were performed under general anesthesia via midline abdominal incision in the same way as day 0. Venous blood samples were drawn from the subclavian vein on days 1, 3, 5, 7, 35, 56, and 84, and euthanasia was performed on day 84.
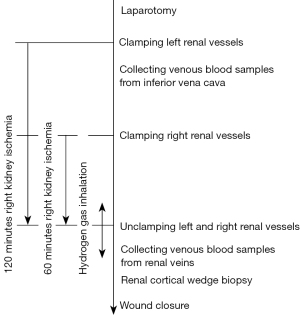
Hydrogen gas inhalation
Two of the four pigs were randomly assigned to the hydrogen gas group (n=2 in hydrogen group and n=2 in control group). Pigs in the hydrogen group received intranasal hydrogen gas inhalation from 10 min before to 30 min after unclamping the renal vessels. Pure hydrogen gas (100%) at 250 mL/min was inhaled from a handmade nasal cannula for pigs inserted under the veterinary anesthesia mask. In our previous study, we confirmed that the concentration of hydrogen gas was 8.3% of the inhaled volume and that the blood hydrogen saturation was 6.7–9.9% after 10 min of administration (7). Pigs in the control group were treated similarly to those in the hydrogen gas group except that they did not receive hydrogen gas inhalation.
Histological evaluation
Samples of renal cortical wedge biopsies and whole kidneys were fixed in 3.7% neutral formaldehyde solution, paraffin-embedded, stained with hematoxylin eosin (HE) and Masson trichrome (MT), and histologically evaluated by two external pathologists (YT and HK; Septosapie Co., Ltd., Tokyo, Japan).
Regarding acute IRI, the areas of tubular necrosis, loss of the brush border, degeneration, cast formation, and tubule dilation were scored using a semiquantitative scale designed to evaluate changes in the kidney after IRI, which were scored as follows: 0=0–5%, 1=5–10%, 2=11–25%, 3=26–45%, 4=46–75%, and 5=>76% (15). Five fields (200× magnification) were analyzed in each kidney at random.
Regarding interstitial fibrosis, the ratio of fibrotic areas in the MT-stained sections was calculated as follows: a single hot spot of fibrosis in MT-stained specimens was photographed at 40× magnification, and the image was analyzed using Image J version 1.52 software (National Institutes of Health, Bethesda, MD, USA) to extract the blue area of fibrosis and determine the ratio of fibrotic area (pixels) to the examination field (pixels) of view (16). Three sections of each kidney were selected at random.
Quantification of cell free DNA
Plasma of venous blood samples from the pre-IRI inferior vena cava and post-IRI renal veins were collected and stored at −80 ℃. DNA content was measured using the Quant-iTTM PicoGreen dsDNA Assay Kit (Thermo Fisher Scientific, Waltham, MA, USA), according to the manufacturer’s instructions.
Statistical analysis
Statistical analyses were not performed because of the small sample size in the large animal model. Instead of statistical data, the raw data were represented.
Results
Post-operative course and trajectory of renal function
All pigs survived until the end of the observation period. The following analysis was performed using all data from all animals (n=2 in hydrogen group and n=2 in control group). Serum creatinine and urea nitrogen levels are shown in Figure 2. Serum creatinine and urea nitrogen levels were elevated until days 1–3 and then reduced to normal levels. We observed a period of appetite loss for a few days after days 0 and 7 surgeries, but this recovered gradually. Trajectories in renal function measurements from blood tests in the hydrogen gas and control groups were similar.
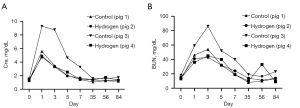
Acute phase evaluation of IRI
The mean increase in plasma DNA levels released from the kidneys by IRI (difference between post-IRI renal vein level and pre-IRI inferior vena cava level) was higher in the 120 min ischemia kidneys than in the 60 min ischemia kidneys and decreased in the hydrogen gas group (Figure 3A).
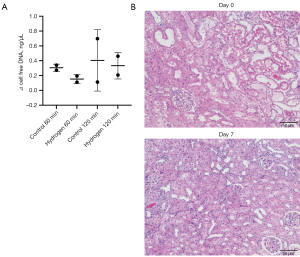
Representative images of HE staining of the kidney cortex on days 0 and 7 are shown in Figure 3B. Almost all HE-stained kidney cortex specimens on day 0 showed severe tubular damage, including loss of the brush border, cast formation, and tubular dilation or necrosis. These findings slightly improved by day 7 (Figure 4). There appeared to be no difference between 60 and 120 min groups, or between hydrogen gas and control groups.
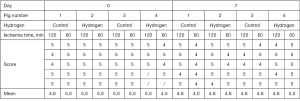
Chronic phase evaluation of IRI
Representative images of MT staining of the kidney cortex on day 84 are shown in Figure 5A. The ratio of fibrotic areas on MT staining was elevated in the 120 min ischemia group compared to that in the 60 min ischemia group (Figure 5B). No fibrosis inhibition was observed in the hydrogen gas group. Since partial scarring was observed in the area where the biopsy was taken, it was excluded from the calculation of the fibrotic area (Figure 5C).
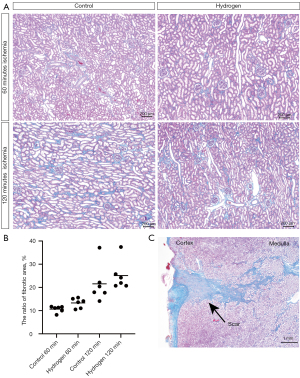
Discussion
The present study demonstrated the first porcine IRI model that induced prolonged ischemia time and subsequent reperfusion injury without threatening the lives of pigs by subjecting the left and right kidneys to different ischemia times. This model allowed us to observe the different renal responses to two ischemia times from a single pig.
In this model, the amount of degraded DNA released from the kidneys; a marker of acute injury and long-term renal interstitial fibrosis; a marker of chronic injury, appeared to increase in response to the ischemia time although this difference was not statistically significant.
There are some advantages to applying different ischemia times to both kidneys. First, applying different ischemia times to the left and right kidneys could provide two-fold information and halve the number of pigs needed for experiments compared to simultaneous bilateral ischemia models or unilateral ischemia models in which the contralateral kidney is removed or left untreated. Since experiments with pigs are expensive and most researchers cannot afford a large number of pigs, it is desirable to obtain a comprehensive amount of information from a limited number of pigs. Second, the two kidneys from the same pig were considered almost equal, and their outcomes were easy to compare. In fact, in this study, the left and right kidneys with different ischemia times showed differences in acute and chronic markers of renal IRI. Third, this model can reduce the mortality rate of pigs despite the bilateral induction of renal IRI. In a previous review, it was reported that unilateral nephrectomy along with ischemia for 120 min in the contralateral kidney results in irreversible damage and increased animal mortality (13). In the present study, all animals survived until the end of the observation period because the contralateral kidney was only mildly damaged. In the field of kidney transplantation, the use of marginal grafts with long ischemia time is increasing due to the global shortage of organs, and there are opportunities to use kidneys with more than 120 min of warm ischemia time (17). Therefore, models that can safely induce a prolonged ischemia time of more than 120 min are required for research targeting such clinical scenarios. In this study, the right and left kidneys were subjected to 60 and 120 min of ischemia, respectively, and it may be possible to subject them to ischemia for longer than 120 min. However, there are also disadvantages. It was difficult to interpret the mixed results of the left and right kidneys when following up with peripheral blood samples, including creatinine or blood urea nitrogen. However, the condition of each kidney immediately before and after IRI can be evaluated by collecting renal venous blood or kidney biopsy specimens separately, as in this study. It is difficult to accurately determine long-term outcomes only from blood samples taken immediately after IRI. However, looking at future therapeutic interventions for renal IRI, local blood flowing out of the IR-injured kidney can be used for research to find new biomarkers or molecular mechanisms as a therapeutic target. Therefore, it is necessary to choose an appropriate model depending on the purpose.
Regarding the effect of hydrogen gas inhalation on renal IRI, the release of DNA from kidneys immediately after IRI appeared to be suppressed in the hydrogen group, although this could not be determined definitively at n=2 in each group. This was thought to be due to the suppression of tubular cell necrosis and subsequent necroinflammation. The biopsy pathology focusing on the cortex in this study did not show a difference in the tubular damage score; however, our previous evaluation of samples from the medulla on day 3 with 60 min of ischemia showed a slight but significant decrease (Figures S1,S2). The present study could not statistically demonstrate the effect of hydrogen gas on long-term renal fibrosis after IRI. Hydrogen gas is known to scavenge ROS (4), inhibit platelet adhesion (5), and reduce IRI in certain organs in various rodent models (6). Some researchers believe that the difference in response to therapeutic agents to IRI in large and small animals is due to differences in the immune system, which is a major component of the inflammatory response in acute kidney injury (18). Further studies in large animals are needed to determine whether hydrogen gas has an inhibitory effect on renal IRI, and when and how it should be administered.
The present study had several limitations. First, due to the high cost, we were only able to use four pigs. Because of the small sample size, we were not able to perform statistical analyses of the changes in outcomes with ischemia time or hydrogen gas in this novel model. Second, since the biopsies on days 0 and 7 were performed by resecting the wedge-shaped renal parenchyma the specimen did not contain medulla, therefore we could not evaluate the medulla. Performing a needle biopsy instead of a wedge biopsy, would allow for the evaluation of the medulla, and may produce more thorough results in this regard as renal ischemia has been reported to affect the medulla more than the cortex. Third, it could be possible that the renal IRI of one side alleviates the simultaneously induced renal IRI of the other side, although the mechanism of remote ischemic preconditioning has not been fully revealed (19). Although this problem could be true for all models of bilateral renal IRI, the results in the present model, in which the left and right kidneys were subjected to different ischemia times, need to be interpreted more carefully. This study is the first step towards establishing a new renal IRI model. We hope that this preliminary model will be further developed and utilized to provide researchers around the world conducting pig experiments with limited funds and facilities with reliable data.
In conclusion, we developed a novel model of ischemia/reperfusion of the kidneys in pigs. Applying different ischemia times between the right and left kidneys allows safe induction of prolonged ischemia time and reduces the animal burden and costs. Further studies are needed to verify the effects of hydrogen gas on renal IRI.
Acknowledgments
We would like to thank Yoshiki Tate and Hiroaki Kawaguchi (Septosapie Co., Ltd., Tokyo, Japan) for the histological evaluation. We would also like to thank Editage (https://www.editage.jp/) for English language editing.
Funding: This study was supported by a research grant from the Doctors Man Co., Ltd. with Keio University School of Medicine (co-operative research No. 04-080-3434).
Footnote
Reporting Checklist: The authors have completed the ARRIVE reporting checklist. Available at https://tau.amegroups.com/article/view/10.21037/tau-21-1164/rc
Data Sharing Statement: Available at https://tau.amegroups.com/article/view/10.21037/tau-21-1164/dss
Conflicts of Interest: All authors have completed the ICMJE uniform disclosure form (available at https://tau.amegroups.com/article/view/10.21037/tau-21-1164/coif). EK and MS are medical advisors of Doctors Man Co., Ltd. The other authors have no conflicts of interest to declare.
Ethical Statement: The authors are accountable for all aspects of the work in ensuring that questions related to the accuracy or integrity of any part of the work are appropriately investigated and resolved. The animal experiment was approved by the Jikei Medical University Animal Research Ethics Committee (approval No. 2021-014) and the Research Council and Animal Care and Use Committee of Keio University (approval No. 20005). The experiment was performed in the facility of Fuji Micra Inc. (Shizuoka, Japan). Animals were treated according to Guidelines for Proper Conduct of Animal Experiments, Science Council of Japan, 2006 (14).
Open Access Statement: This is an Open Access article distributed in accordance with the Creative Commons Attribution-NonCommercial-NoDerivs 4.0 International License (CC BY-NC-ND 4.0), which permits the non-commercial replication and distribution of the article with the strict proviso that no changes or edits are made and the original work is properly cited (including links to both the formal publication through the relevant DOI and the license). See: https://creativecommons.org/licenses/by-nc-nd/4.0/.
References
- Jansen MP, Emal D, Teske GJ, et al. Release of extracellular DNA influences renal ischemia reperfusion injury by platelet activation and formation of neutrophil extracellular traps. Kidney Int 2017;91:352-64. [Crossref] [PubMed]
- Lee S, Huen S, Nishio H, et al. Distinct macrophage phenotypes contribute to kidney injury and repair. J Am Soc Nephrol 2011;22:317-26. [Crossref] [PubMed]
- Tanaka M, Saka-Tanaka M, Ochi K, et al. C-type lectin Mincle mediates cell death-triggered inflammation in acute kidney injury. J Exp Med 2020;217:e20192230. [Crossref] [PubMed]
- Ohsawa I, Ishikawa M, Takahashi K, et al. Hydrogen acts as a therapeutic antioxidant by selectively reducing cytotoxic oxygen radicals. Nat Med 2007;13:688-94. [Crossref] [PubMed]
- Wang Y, Wu YP, Han JJ, et al. Inhibitory effects of hydrogen on in vitro platelet activation and in vivo prevention of thrombosis formation. Life Sci 2019;233:116700. [Crossref] [PubMed]
- Abe T, Li XK, Yazawa K, et al. Hydrogen-rich University of Wisconsin solution attenuates renal cold ischemia-reperfusion injury. Transplantation 2012;94:14-21. [Crossref] [PubMed]
- Kobayashi E, Sano M. Organ preservation solution containing dissolved hydrogen gas from a hydrogen-absorbing alloy canister improves function of transplanted ischemic kidneys in miniature pigs. PLoS One 2019;14:e0222863. [Crossref] [PubMed]
- Nishi K, Iwai S, Tajima K, et al. Prevention of Chronic Rejection of Marginal Kidney Graft by Using a Hydrogen Gas-Containing Preservation Solution and Adequate Immunosuppression in a Miniature Pig Model. Front Immunol 2021;11:626295. [Crossref] [PubMed]
- Liu S, Xu J, Gao Y, et al. Multi-organ protection of ulinastatin in traumatic cardiac arrest model. World J Emerg Surg 2018;13:51. [Crossref] [PubMed]
- Matějková Š, Scheuerle A, Wagner F, et al. Carbamylated erythropoietin-FC fusion protein and recombinant human erythropoietin during porcine kidney ischemia/reperfusion injury. Intensive Care Med 2013;39:497-510. [Crossref] [PubMed]
- Barrera-Chimal J, André-Grégoire G, Nguyen Dinh Cat A, et al. Benefit of Mineralocorticoid Receptor Antagonism in AKI: Role of Vascular Smooth Muscle Rac1. J Am Soc Nephrol 2017;28:1216-26. [Crossref] [PubMed]
- Jayle C, Milinkevitch S, Favreau F, et al. Protective role of selectin ligand inhibition in a large animal model of kidney ischemia-reperfusion injury. Kidney Int 2006;69:1749-55. [Crossref] [PubMed]
- Orvieto MA, Tolhurst SR, Chuang MS, et al. Defining maximal renal tolerance to warm ischemia in porcine laparoscopic and open surgery model. Urology 2005;66:1111-5. [Crossref] [PubMed]
- Science Council of Japan. Guidelines for Proper Conduct of Animal Experiments. 2006. [cited 13 December 2021]. Available online: https://www.scj.go.jp/ja/info/kohyo/pdf/kohyo-20-k16-2e.pdf
- Hou J, Cai S, Kitajima Y, et al. 5-Aminolevulinic acid combined with ferrous iron induces carbon monoxide generation in mouse kidneys and protects from renal ischemia-reperfusion injury. Am J Physiol Renal Physiol 2013;305:F1149-57. [Crossref] [PubMed]
- Schneider CA, Rasband WS, Eliceiri KW. NIH Image to ImageJ: 25 years of image analysis. Nat Methods 2012;9:671-5. [Crossref] [PubMed]
- Manara AR, Murphy PG, O'Callaghan G. Donation after circulatory death. Br J Anaesth 2012;108:i108-21. [Crossref] [PubMed]
- Packialakshmi B, Stewart IJ, Burmeister DM, et al. Large animal models for translational research in acute kidney injury. Ren Fail 2020;42:1042-58. [Crossref] [PubMed]
- Gassanov N, Nia AM, Caglayan E, et al. Remote ischemic preconditioning and renoprotection: from myth to a novel therapeutic option? J Am Soc Nephrol 2014;25:216-24. [Crossref] [PubMed]