Deciphering the myth of icariin and synthetic derivatives in improving erectile function from a molecular biology perspective: a narrative review
Introduction
Erectile dysfunction (ED) is defined as the inability to obtain and/or maintain a sufficient erection for satisfying sex over three months. Currently, oral administration of type 5 phosphodiesterase inhibitors (PDE5Is) given before intercourse are the first-line treatment for ED patients (1). Second-line treatments for ED, including the vacuum devices and intracavernose injection therapy (ICI), present numerous complications like penile pain, priapism, and fibrosis. The overall efficiency of both was about 70%, however, these treatments were only used to relieve ED symptoms. The implantation of a penile prosthesis might be the last option, but is highly cost with the risks of infection, erosion and device failure (2). Recent researches on ED regenerative therapies including gene therapy and stem cell therapy have shown significant benefits in repairing pathological changes of penile tissue of various ED animal models and are important attempts to restore natural erections. However, their clinical application is limited by ethical and potential safety issues (3). Anyhow, a natural erection restoration is always the primary pursuit.
Traditional Chinese medicine has a long history in exploring ED therapy. Many studies have demonstrated the effectiveness of Epimedium for the treatment of ED. The history of Epimedium in ED therapy can be traced back to the ancient Chinese North and South Dynasties (420-589 AD). Tao Hongjing, a famous medical scientist, learned from the shepherds that male sheep consumed a plant that significantly increased the times of penile erections and mating. Tao believed this plant could enhance the “YANG” energy (in Chinese, “Yin Yang Huo”, in English, Epimedium Herb, EH). It has also been widely used in East Asian countries for centuries, with different names such as Epimedium Brevicornum Maxim, E. Sagittatum Maxim, E. Pubescens Maxim, and E. Koreanu Nakai.
Flavonoids have been reported to be the main bioactive components of Epimedium (4). Icariin (ICA) is one of the most abundant flavonoids in Epimedium and is often used as a marker for quality control in Epimedium herbal preparation and chemical taxonomy (5,6). ICA has a similar structure to PDE5Is. In addition, it has a wide range of other pharmacological effects, including anticancer activity (7), anti-osteoporotic activity (8,9), antidepressant activity (10,11), and aphrodisiac (12). ICA is a disaccharide, and several studies have shown that flavonoids in the form of glycosides have low bioactivity because of their low intrinsic absorption permeability (13-15). Both in vivo and in vitro metabolic studies demonstrated that the intestinal microbiota can convert ICA into icariside I, icariside II (ICAII) by hydrolyzing C-3-O-rhamnoside (R1) and C-7-O-glucopyranoside (R2) molecule, respectively (16-19). Pharmacokinetic studies have shown that the intestinal bacterial metabolites of ICA have better biological activity (20,21). Previous studies found that after oral administration, 91.2% of ICA was converted to ICA II. The maximum blood concentration (Cmax) and the degree of absorption (AUC0-t) that occurred after ICA II administration were 3.8 and 13.0 times higher than those of ICA, respectively. Therefore, ICA II, which lacks a glucose group at C-7, is the major metabolite of ICA and has higher activity than ICA (22).
By administering different doses of ICA or ICA II treatment, it was found that appropriate doses (generally low dose, ranging from 10–200 mg/kg) significantly improved erectile function in various ED animal models. Moreover, ICA and ICAII are able to reverse the injury of penile corpus cavernosum, such as restoring the content of cavernous endothelium (23) and smooth muscle (24), regenerating damaged cavernous nerves (25), inhibiting cavernous fibrosis (26), restoring the normal level of testosterone and the ratio of extracellular matrix (27,28). Therefore, ICA/ICAII may have the potential to rehabilitate the pathological injury and achieve spontaneous erection.
In current review, we summarized the advancements in terms of the biological effect of ICA and ICA derivatives on type 5 phosphodiesterase (PDE5) expression and enzymatic function, the modulation of tissue resident stem cells to regenerate penile damaged tissues, and the restoration of testosterone level. We present the following article in accordance with the Narrative Review reporting checklist (available at https://tau.amegroups.com/article/view/10.21037/tau-22-232/rc).
Methods
We conducted a literature search for PUBMED in March 2022 following the Table 1 search strategy. The publication time is limited to last 20 years. Articles had to be published in peer reviewed journals. Keyword searches including “erectile dysfunction” AND “icariin”, “erectile dysfunction” AND “icariside II”, “erectile dysfunction” AND “icariin derivative”, “PDE5” AND “icariin”, “PDE5” AND “icariside II”, “PDE5” AND “icariin derivative”, “testis” AND “testosterone” AND “icariin” , and “testis” AND “testosterone” AND “icariside II”, “testis” AND “testosterone” AND “icariin derivative”. Articles without experimental data related to the search terms and data that did not support relevant conclusions were excluded, as well as articles with low quality. The data were extracted from each study by two investigators (JCP and YHF) independently. After the search, summarized the literature and discussed the different literature. Another researcher (ZCX) was invited to discuss and identify the literature on which consensus could not be reached (Table S1). Our literature search covered English-language clinical or basic research articles published from January 1, 2003 to March 1, 2022, as well as related reviews and meta analyses.
Table 1
Items | Specification |
---|---|
Date of search (specified to date, month and year) | January 1, 2003 to March 1, 2022 |
Databases and other sources searched | PubMed |
Search terms used (including MeSH and free text search terms and filters) (Note: please use an independent supplement table to present detailed search strategy of one database as an example) | As shown in Table S1 |
Timeframe | The publication time is limited to last 20 years |
Inclusion and exclusion criteria (study type, language restrictions etc.) | 1. Articles had to be published in peer reviewed journals |
2. Articles without experimental data related to the search terms and data that did not support relevant conclusions were excluded, as well as articles with low quality | |
3. Written by English | |
Selection process (who conducted the selection, whether it was conducted independently, how consensus was obtained, etc.) | Two experimenters were searched and screened separately. Discussion of the discrepancy literature and invitation to the other experimenter to participate |
Any additional considerations, if applicable | None |
Discussions
Modulating PDE5 expression and function
Numerous studies have shown that ICA and ICA derivatives affected the enzymatic function of PDE5 mainly through competitive inhibition, while the possible regulatory role of PDE5 expression needs to be further investigated (Table 2). Protein binding of ICA/ICA derivatives and PDE5.
Table 2
ICA and ICA derivative | Year of publication | Study type | Mechanism of PDE5 inhibition | The inhibition Effect for PDE5 | Key reference |
---|---|---|---|---|---|
ICAII | 2006 | In vitro (crystal structure and enzyme kinetics) | Bonding with PDE5A1 flexible H-loop | Selectively inhibiting PDE5A1 with an IC50 of 2M | (29) |
ICA derivative-Compounds 3 | 2019 | In vitro (crystal structure and enzyme kinetics) | The hydrophobic group at the 3-o position more significantly inhibiting PDE5 | Inhibiting PDE5 with an IC50 of 0.083±0.01 μM | (30) |
ICA | 2003 | In vitro (enzyme kinetics) | – | Inhibiting PDE5 with an IC50 of 0.432 µM | (31) |
ICA | 2006 | In vitro (rat cavernous smooth muscle cells and enzyme kinetics) | – | Inhibiting PDE5A1, A2, and A3 with an IC50 value of 1.0, 0.75, and 1.1 M | (32) |
ICAII | 2012 | In vitro (rat corpus cavernosum tissue and enzyme kinetics) | – | about 50% of Sildenafil | (33) |
3,7-bis(2-hydroxyethyl) Icaritin | 2008 | In vitro (enzyme kinetics) | – | With a similar IC50 to that of sildenafil (IC50 75 vs. 74 nM) | (34) |
ICA | 2006 | In vitro (rat corpus cavernosum tissue and enzyme kinetics) | Inhibit PDE5 mRNA expression | Inhibiting PDE5 with EC50 was 4.62 micromol/L | (35) |
ICA | 2014 | In vivo (rat corpus cavernosum tissue and enzyme kinetics) | Inhibit PDE5 expression | – | (36) |
PDE5, phosphodiesterase type 5; ICA, icariin; ICAII, icariside II; IC50, the half maximal inhibitory concentration; EC50, concentration for 50% of maximal effect.
The catalytic domain of PDE5 can and the active site, which realizes the role of bind and hydrolyze cyclic guanosine monophosphate (cGMP), while the H-loop structure can modulate the enzyme affinity. Crystal structure of ICAII binding to PDE5 shows that the 7-o-glucose structure is located near the entrance of the active binding site and forms hydrogen bonds with ser668 residues on the flexible H-loop. In addition, the 3-o-rhamnose structure is located in the hydrophobic region that may affect the catalytic efficiency of the enzyme (29,37). In 2019, Chau et al. (30) demonstrated that different functional groups at the 3-o and 7-o positions of the ICA derivative backbone play a role in modulating the ability of ICA to inhibit PDE5. The substitution of the 3-o and 7-o positions using different hydrophilic and hydrophobic groups revealed that the hydrophobic group at the 3-o position had a more pronounced effect on the inhibition of PDE5.
Enzymatic inhibition of PDE5 function by ICA and ICA derivatives
A previous study showed that ICA exhibited a dose-dependent inhibition of PDE5 activity (31). The half-maximal inhibitory concentrations (IC50) of ICA for PDE5 and phosphodiesterase-4 (PDE4) were 0.432 mmol/L and 73.50 mmol/L, respectively. Ning and colleagues (32) also reported that ICA inhibited all three PDE5 isomers with similar IC50 values. However, the inhibitory effect of ICA on PDE5 is about one-tenth that of sildenafil, while the effect of ICA II is significantly higher than ICA, about 50% of sildenafil (33).
In addition, Dell’Agli et al. (34) showed that 3,7-bis (2-hydroxyethyl) icaritin has a strong inhibitory effect on PDE5A1 with a similar IC50 to that of sildenafil (IC50 75 vs. 74 nM) and 80 times than ICA. Therefore, chemical modifications of the ICA molecular structure have been investigated with the aim of obtaining more specific PDE5 inhibitory activity. Recently, Chau et al. (30) developed several novel semi-synthetic ICA derivatives, among which compounds 3 and 7 synthesized by modification with hydroxyethyl substitution of C-3-O-rhamnoside showed specific PDE5 inhibitory activity close to that of commercially available PDE5 inhibitors. Additionally, compound 3 showed less phosphodiesterase-6C (PDE6C) inhibitory effect compared to sildenafil. Compared to naturally derived ICA, synthetic and chemically modified ICA derivatives have good PDE5 inhibition and specificity, and may be promising for further development as better PDE5Is candidates. However, studies on the efficacy of ICA chemically modified compounds are limited to in vitro experiments, while safety needs to be verified in more animal experiments and even clinical trials.
ICA regulates PDE5 expression
In contrast to commercial PDE5Is such as sildenafil, a previous study suggested that ICA may have the ability to inhibit PDE5 expression. Jiang et al. (35) compared the differences of cGMP, cyclic adenosine monophosphate (cAMP) and PDE5 mRNA by exposing isolated rabbit penile corpus cavernosum to ICA solution and sildenafil solution. The results showed an increase in tissue cGMP levels in both solutions, with no significant change in cAMP. The term-half maximal effective concentration (EC50) was 4.62 (ICA) and 0.42 (Sildenafil) µmol/L respectively. More importantly, ICA treatment also differentially inhibited PDE5A1 and PDE5A2 mRNA levels in rat penile corpus cavernosum compared with sildenafil. Another study in which ICA was administered to adult rats showed that ICA significantly reduced PDE5 levels in penile tissues, and these results may be related to the reduction of Rho signaling pathway associated coiled-coil containing protein kinase 1 and 2 (ROCK1 and ROCK2) levels by ICA (36). Thus, ICA treatment is considered to have a longer-term PDE5 inhibitory effect than commercially available PDE5Is. This study suggests another possible direction for future research. However, there are only a few studies on the regulation of PDE5 expression by ICA, and definite conclusions need to be confirmed by clear mechanistic studies and experiments in large sample to exclude distortion of results due to individual differences.
Repair of penile tissue mainly through regulation of tissue-resident stem cells
In fact, the majority of ED patients are not satisfied with one-time symptom improvement and want new therapies that are safer and more effective in restoring erectile function by repairing the pathological changes in ED. Numerous studies have demonstrated the effectiveness of ICA and its derivatives in repairing injured penile tissue. The above-mentioned effect through the regulation of tissue-resident stem cell proliferation and differentiation is one of the most important mechanisms currently being investigated for the treatment of ED (Table 3). Therefore, ICA and its derivatives are considered to be regenerative medicine therapies with the potential to restore spontaneous erections in patients with ED.
Table 3
ICA and ICA derivative | Year of publication | Study design | Study type | Stem cell type | Treatment results | Molecular Mechanisms | Effective dose | STAIR list score (full 7 points) | Key reference |
---|---|---|---|---|---|---|---|---|---|
ICAII | 2013 | Streptozotocin-induced diabetic ED rats | In vivo | SMCs | Increased SMC proliferation and decreased the numbers of autophagosomes | Up-regulated NO-cGMP and downregulated mTOR pathway | 10 mg/kg/day | 4 | (24) |
ICA | 2017 | Male rats with bilateral cavernous nerves injury | In vivo | NSCs | Repaired the damaged neural pathway for erection; Promoted differentiation of endogenous stem cells to Schwann cells | – | 1.5 mg/kg/day | 5 | (25) |
ICA | 2011 | Streptozotocin-induced diabetic ED rats | In vivo | – | Promoted smooth muscle/collagen ratio and endothelial cell content in the corpora cavernosa | up-regulated vWF and PECAM; down-regulated TGFβ1/Smad2 signaling pathway | 1, 5, and 10 mg/kg/day | 5 | (26) |
ICAII | 2018 | Zucker Fatty (ZUC-Leprfa 185; ZF) male rats | In vivo | – | Prevented penile smooth muscle atrophy, endothelial dysfunction, and lipid accumulation | – | 1.5 mg/kg/day | 4 | (38) |
Activated more penile stem cells to proliferate and differentiate | |||||||||
ICAII | 2014 | Male rats with bilateral cavernous nerves injury | In vivo | – | Prevented distortion of normal neural anatomy, smooth muscle atrophy, and collagen deposition of penile | – | 0.5, 1.5 or 4.5 mg/kg/day | 4 | (39) |
Promoted differentiation of penile endogenous stem cells |
|||||||||
ICAII | 2016 | Streptozotocin-induced diabetic ED rats | In vivo | – | Increased density of dorsal nerve bundle of penile | Increased NGF expression | 5 mg/kg/day | 5 | (40) |
YS 10 | 2021 | Male rats with bilateral cavernous nerves injury | In vivo | – | Repaired corpora cavernous nerves injury | Increased β-Catenin and cyclin D1 expression | 2.5 mg/kg/day | 5 | (41) |
ICA | 2018 | NSCs isolated from rats and EdU labeled | In vitro | NSCs | Increased the number of stem cell spheres | Increased mRNA and protein expression of cell cycle genes cyclin D1 and p21 | 50 & 100 μmol/L | – | (42) |
ICA | 2014 | NSCs isolated from mice embryos and EdU labeled | In vitro | NSCs | Increased neurosphere formation and NSCs with EdU | Activated ERK/MAPK pathway | 100 μmol/L | – | (43) |
ICA | 2016 | NSCs isolated from 16‑20‑week human fetuses | In vitro | NSCs | Enhanced NSCs proliferation and neurosphere formation | Upregulated Frizzled 7, DVL3, FGFR1 and down-regulated GSK-3β | 10 μmol/L | – | (44) |
ICA | 2020 | Aβ25-35-treated hippocampal neural stem cells of rats | In vitro | NSCs | promoted the viability and differentiation into neurons and astrocytes | Activated BDNF-TrkB-ERK/Akt signaling pathway | 20, 40, 80 μmol/L | – | (45) |
ICA | 2022 | Chronic unpredictable mild stress for depression rat; Corticosterone treated NSCs from rats embryonic hippocampi | In vivo & In vitro | NSCs | Alleviated dysfunctional neurogenesis and neuronal loss | Down-regulated Rps4x, Rps12, Rps14, Rps19, Hsp90b1, and Hsp90aa1 and up-regulated HtrA1 | 120 mg/kg/day in vivo and 20 μmol/L s in vitro | 5 | (46) |
Promoted neuronal proliferation and differentiation | |||||||||
ICAII | 2012 | Streptozotocin-induceddiabetic ED rats | In vivo | – | Promoted corpus cavernosum smooth muscle/collagen ratio and endothelial cell content | Down-regulated TGFβ1/Smad2/CTGF and up-regulated NO-cGMP | 1, 5, and 10 mg/kg/day | 4 | (47) |
ICA | 2019 | High glucose-induced rats bone marrow derived EPCs | In vitro | EPCs | Partially restored EPCs migration and tube formation | Inhibited p38/CREB pathway and activated Akt/eNOS/NO pathway | 1μmol/L | – | (48) |
ICA | 2015 | H2O2-induced rats bone marrow derived EPCs | In vitro | EPCs | Promoted cell migration and capillary tube formation, abrogated apoptotic and autophagic programmed cell death | Reduced ROS levels and restored ΔΨm; activated rapamycin /p70S6K/4EBP1 and increased ATF2 and ERK1/2 protein levels | 7.5, 15, and 30 µM | – | (49) |
ICAII | 2020 | Streptozotocin-induced diabetic ED rats | In vivo | SMCs | Increased smooth muscle cell/collagen fibril proportions, decreased mitochondrial autophagy, and AGE concentrations | – | 10 mg/kg/day | 4 | (50) |
ICA | 2020 | Monocrotaline-Induced Pulmonary Arterial rats | In vivo | – | Decreased right ventricular systolic pressure (RVSP) and the right ventricular hypertrophy index (RI) | Inhibited TGF-β1, Smad2/3, P-Smad2/3, and MMP2 expressions | 50 or 100 mg/kg/day | 4 | (51) |
ICAII | 2018 | Hemorrhage injection model of subarachnoid hemorrhage rats | In vivo | – | Inhibited subarachnoid fibrosis, attenuated ventriculomegaly, and chronic hydrocephalus | Inhibited TGF-β1/Smad/CTGF signaling pathway | 1, 5, 10 mg/kg/day | 4 | (52) |
ICA, icariin; ICAII, icariside II; NSCs, neural stem cell; EPCs, endothelial progenitor cells; SMCs, smooth muscle cells; TGF-β1, transforming growth factor β1; Smad2, SMAD family member 2; CREB, cAMP response element binding protein; AGE, advanced glycation end products; ERK, extracellular signal-regulated kinase; Akt, protein kinase B; MAPK, mitogen-activated protein kinase; NO, nitric oxide; eNOS, nitric oxide synthase; Frizzled 7, frizzled class receptor 7; DVL3, dishevelled segment polarity protein 3; FGFR1, fibroblast growth factor receptor 1; GSK-3β, Glycogen synthase kinase-3β; ΔΨm, mitochondrial membrane potential; ox-LDL, oxidized low‑density lipoprotein; EdU, 5-ethynyl-2-deoxyuridine; STAIR list, initial stroke therapy academic industry roundtable (evaluation tools commonly used in quality assessment of animal experiments); BDNF, brain-derived neurotrophic factor; cGMP, cyclic guanosine monophosphate; vWF, von Willebrand factor; PECAM, platelet endothelial cell adhesion molecule; NGF, nerve growth factor; ERK, extracellular regulated protein kinases; BDNF, brain derived neurotrophicfactor; TrkB, tyrosine kinase receptor B;CTGF, connective tissue growth factor; p70S6K, ribosomal protein S6 kinase, 70kDa; 4EBP1, 4E-binding protein 1; ATF2, activating transcription factor-2; MMP2, matrix metalloproteinase; Rps4x, ribosomal protein S4 X; Rps12, ribosomal protein 12; Rps14, ribosomal protein 14; Rps19, ribosomal protein 19; Hsp90b1, heat shock protein 90 beta family member 1; and Hsp90aa1, heat shock protein 90 alpha family class A member 1; HtrA1, high-temperature requirement protein A1.
Tissue resident stem cells activation
Stem cells can self-replicate and differentiate into multiple cell types, which are widely used in regenerative medicine research. Recently, many studies have shown that ICA and ICAII can regulate the biological behaviors of a variety of stem cells, including bone marrow mesenchymal stem cells (BMSCs), adipose-derived stem cells (ADSCs), neural stem cells (NSC), endothelial progenitor cells, human umbilical cord mesenchymal stem cells (53-56). Previous studies have demonstrated that ICA could induce BMSC proliferation, differentiation, and ameliorate prednisolone-induced BMSC apoptosis in vitro and in vivo (57,58). Ye et al. (54) reported that diabetic ED rats treated with ADSCs and ICA showed significant improvement in the intracranial pressure (ICP) and ICP/mean arterial pressure (MAP) values. Besides, ICA application increased the survival rate of transplanted ADSCs and repaired the damaged structures of the corpus cavernosum (59). Exogenous stem cell transplantation combined with ICA is effective in treating ED methods, but the escape of stem cells after transplantation and proliferation can lead to side effects such as pulmonary embolism, which has hindered the clinical application (60).
In contrast, endogenous stem cells residing in tissues are safer. Endogenous stem cells (ESCs) or progenitor cells (EPCs), also known as tissue resident stem cells, are present in many organs. In recent years, Scholars are gradually turning their attention to endogenous stem cell research in the penis, and some breakthroughs have been achieved. The label-retaining cell (LRC) strategy is a commonly used technique for identification of stem cells in tissue (61,62). Its mechanism is based on the principle that the rapidly proliferating cells will lose the cell label in a short period of time, while quiescent cells and slow-cycling cells will retain the label for a longer period. A recent research had identified potential stem/progenitor cells in the penis by using a co-localization strategy of 5-ethynyl-2-deoxyuridine (EDU)-LRC and cell differentiation markers (63). Treatment with ICAII in obese and cavernous nerve-injured rats showed that penile stem cell mitosis was significantly increased and multidirectional differentiation occurred to repair histopathological changes in the penile corpus cavernosum (64,65). Researches on the molecular mechanisms involved has increased significantly in recent years. Wingless-type MMTV integration site family (Wnt)/β-catenin, p38 mitogen-activated protein kinase (p38 MAPK), transforming growth factor-β (TGF-β)/Smad, phosphatidyl inositol 3-kinase (PI3K)/serine/threonine-specific protein kinase Akt (Akt) and mammalian target of rapamycin (mTOR) signaling pathways are well-documented signaling pathways in the study of ICA-regulated stem cells (Figure 1) (38-41,66-70). Therefore, ICAII may have a role in regulating penile stem cells to repair pathological damage to the corpus cavernosum and restore erectile function.
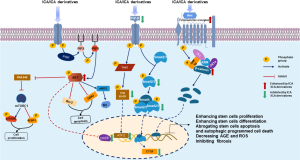
It should first be stated that the pathophysiology of ED is often interconnected and coexistent, including loss of smooth muscle and endothelial cells, abnormal collagen ratios, and reduction of neural nitric oxide synthase (nNOS)-positive nerves, and that the role of ICA in repairing penile injured and restoring erectile function should be studied as a whole. In order to more clearly illustrate the therapeutic effect of ICA, it was classified according to the study type of animal model and stem cell.
Cavernous nerve regeneration
Erection is a complex physiological process. After the brain receives sexual stimulation, nerves transmit it to the target organ and secrete nitric oxide synthase (nNOS), which coordinates three downstream hemodynamic events: smooth muscle relaxation, arterial dilation and venous restriction, culminating in penile erection. The cavernous nerve (CN) originating from the pelvic ganglion (PG), with sympathetic and parasympathetic fibers, is capable of releasing nitric oxide (NO) and nNOS and activating endothelial cell NOS (eNOS), resulting in a rise in NO content in the relaxed smooth muscle of the cavernous tissue.
ICAII restored nerve density and microstructure of pelvic floor ganglia in the penile corpus cavernosum of ED rats with diabetes-induced nerve damage, and in vitro ICAII resulted in longer synapses and more branching of ganglion tissue (42). In addition, YS-10, a new flavonoid based on the ICA II structure, treated rats with bilateral cavernous nerve injury (BCNI) and showed a significant reduction in smooth muscle atrophy, collagen deposition, endothelial and neurological dysfunction (43). In order to assess the efficacy and mechanisms of ICA treated BCNI rat. Newborn male rats were injected with 5-ethynyl-2-deoxyuridine (EdU, 50 mg/kg) to track endogenous stem cells in penis. Adult rats underwent bilateral cavernous nerve injury in the penis, and the experimental group was treated with ICA (1.5 mg/kg/d) by gavage for 8 weeks. Nerve structures at the ICA-treated injury were restored to normal, and the number of EDU and S100 (a nervous specificity protein)-positive co-expressing cells and nNOS levels were positively correlated and significantly increased (25). It is suggested that promoting endogenous stem cell differentiation is an important mechanism for ICA to repair damaged cavernous nerves. Further studies confirmed the role of ICA to stimulate NSCs self-renewal, proliferation and differentiation in vitro (44,45).
Previous study found that ICAII significantly increased the levels of S100 and NGF in the penile tissue of rats after cavernous nerve injury and prevented distortion of neuroanatomical structures and neurotransmission dysfunction. Meanwhile, the expression trend of p38 MAPK in the penis was basically the same as that of S100, suggesting that the p38 MAPK signaling pathway may be involved in this process (69). In vitro experiments reveal more mechanisms of ICA regulation of NSCs. Yang et al. (46) found that ICA (10 µM for 7 days) enhanced NSCs proliferation and neurosphere formation, by upregulating the expression of frizzled class receptor 7 (the key proteins of Wnt pathway), dishevelled segment polarity protein 3 (the key proteins of Wnt pathway), bFGF receptor 1, and downregulating glycogen synthase kinase-3β (the Wnt pathway inhibitor) in NSCs. In another study suggested that ICA promoted the proliferation and differentiation of NSCs through the brain-derived neurotrophic factor (BDNF)-tyrosine kinase receptor B (TrkB)-extracellular regulated protein kinases (ERK)/Akt signaling pathway. By treating β-amyloid protein (Aβ)-damaged NSCs cells, the scholars found that ICA reversed the reduction of BDNF and TrkB expression and ERK/Akt phosphorylation caused by Aβ toxicity, and promoted NSCs proliferation and differentiation (47). In addition, the PI3K/Akt and IL-17 pathways were found to play a role in the regulation of functional proteins such as Rps14, Hsp90b1 and Htra1 in the cerebrospinal fluid by ICA (48).
However, it should be cautioned that mechanistic studies are often more advantageous in in vitro experiments, but due to technical limitations, penile stem cells and cavernous nerve stem cells are difficult to isolate. With the improvement of technology various tissue stem cell isolation will also be an important research direction.
Endothelium regeneration
After receiving sexual stimulation from cavernous nerve, the cavernous endothelial cells secrete NO to maintain smooth muscle relaxation. Therefore, normal function and number of endothelial cells are important for erectile function of the penis. Studies found that the content of smooth muscle and endothelial cells were significantly reduced in diabetic rats. The expression of platelet endothelial cell adhesion molecule-1 (PECAM-1), eNOS and Von Willebrand factor (vWF) in the cavernous sinus endothelium were also reduced. ICA and ICAII treatments reversed these changes and increased the number of endothelial cells and smooth muscle cells (SMCs), possibly associated with downregulation of the TGFβ1/Smad2 signaling pathway (26,49).
Chen et al. (71) used high glucose to inhibit endothelial progenitor cell viability in a dose-dependent manner, while 1 µM ICA treatment partially restored glucose-induced impairment of EPCs migration and tube formation. One µM ICA significantly inhibited high glucose-induced phosphorylation of p38 and CREB (cAMP-response element binding protein) and increased Akt and eNOS activity in endothelial progenitor cells. Activation of Akt/eNOS could increase NO expression to regulate migration of endothelial progenitor cells. Tang et al. (50) found that ICA had the ability to activate rapamycin/70 kDa ribosomal protein S6 kinase (p70S6K)/eukaryotic translation initiation factor 4E (eIF4E)-binding protein 1 (4EBP1) and increased ATF2 and ERK1/2 protein levels to inhibit oxidative stress-induced damage to EPCs and promoted proliferation and differentiation of EPCs, and augmented capillary tube formation. Similar findings on the effects of ICA treatment on EPCs across different causes of injury further added credibility to the protection and activation effects of ICA for aforementioned signaling pathways on ECP.
Cavernous smooth muscle regeneration
Smooth muscle relaxation leads to rapid filling of the cavernous sinus with arterial blood, resulting in penile erection. Vascular smooth muscle has multiple sources, including mainly the proliferation of smooth muscle itself settled in the vasculature, transdifferentiation of circulating hematopoietic stem cells, and transdifferentiation of stem cells in the outer stromal layer of the vasculature.
Previous studies have demonstrated that ICA and ICAII treatment may reverse the reduction of penile SMCs caused by diabetes through downregulation of the TGF-β1 signaling and upregulate α-smooth muscle actin (α-SMA) (26,49). Furthermore, Ruan et al. (64) found that ICA II (1.5 mg/kg/day for 4 weeks) improved erectile function and smooth muscle pathological changes through activation of endogenous stem cells in obesity-related ED rats. Zhang et al. (24) demonstrated that ICA II (10 mg/kg for 8 weeks) ameliorated SMCs injury and restored smooth muscle to collagen ratio in diabetic rats. In this study, the percentage of SMCs in S phase (DNA synthesis phase), proliferation index (PI) were higher in the ICAII-treated group compared to the diabetic ED group. These effects may be related to the upregulation of the NO-cGMP pathway and the inhibition of excessive SMC autophagy and advanced glycation end products (AGE) deposition by ICAII. However, Hu et al. (72) showed that ICA dose-dependently (10 and 40 µM) inhibited oxidized low-density lipoprotein (ox-LDL)-induced vascular SMC proliferation and suppressed ERK1/2 pathway and PCNA expression. The findings of this study diverge from those mentioned above but seem to be explicable. The complex processes and mechanisms such as oxidative stress in the penis of diabetic and obese rats ultimately lead to reduced damage to SMCs, and ox-LDL in vitro may be a stronger stimulus to activate the ERK1/2 pathway and promote SMC proliferation. Inhibition of oxidative stress by ICAII may be responsible for the discrepancy in the above findings. However, this study has less experimental data and lack of in vitro mechanism studies. Further experimental validation is necessary.
Modulation extracellular matrix and inhibition of fibrosis
Recent studies reported that diabetic rats had a lower ratio of type I to type III collagen, fragmented elastic fibers, and decreased elastic fiber content (27,73). After treating with ICA II (10 mg/kg/day) for 12 weeks, the α-SMA content and the ratio of collagen I to III were significantly higher in penis of diabetic rat compared to the untreated diabetic group. The results also showed a dramatic change in elastic fibers (71), which might be related to the fact that ICA II has the ability to improve lipid metabolism, reduce AGE concentration and mitochondrial autophagy.
There are at least 60 related genes that are downstream targets of TGF-β1 (74). TGF-β1 has been shown to increase collagen synthesis in cultured human corpus cavernosa SMCs in vitro (75). TGF-β1 activated Smad2 and Smad3, leading to fibrosis-related changes (76,77). Connective tissue growth factor (CTGF), which plays an important role in connective tissue homeostasis, fibroblast proliferation, migration, and adhesion, is another downstream target of TGF-β1 (78). The expression of TGF-β1, total Smad2 and phospho-Smad2 was significantly higher in arteries, vena cava and penile corpus cavernosum of diabetic rats. In addition, higher CTGF expression was shown in fibroblasts, endothelial cells and SMCs in the penile tissue of diabetic rats (51,52,79). In contrast, ICA II (1, 5, 10 mg/kg) treatment significantly reduced the expression of TGF-β1/Smad/CTGF signaling pathway members in brain and penile tissues of diabetic rats and inhibited the fibrotic process in penile tissues (80).
Regulating endogenous testosterone (Table 4)
Table 4
ICA/ICA II | Year of publication | Study design | Study type | Treatment results | Molecular mechanisms | Effective dose | STAIR list score (full 7 points) | Key reference |
---|---|---|---|---|---|---|---|---|
ICA | 2020 | Mice with nicotine | In vivo | Improved sperm density, hormone levels and antioxidant enzyme activity | Activated antioxidant enzymes | 75 mg/kg/day | 5 | (28) |
ICA | 2019 | Mouse and Leydig cells with (2-Ethylhexyl) Phthalate | In vivo and in vitro | Promoted cell proliferation, and testosterone levels; Inhibited reactive oxygen species levels, mitochondrial membrane potential | Increased SF-1 and steroidogenic enzymes (CYP11, 3β-HSD and 17β-HSD) | 50, 100 or 150 mg/kg/day in vivo; 1 μg/mL, and 5 μg/mL in vitro | 5 | (80) |
ICA | 2021 | Rat with high fat diet and streptozotocin | In vivo | recovered the number of spermatogonia, primary spermatocytes and Sertoli cells | upregulated the expression of PCNA, activated SRIT1-HIF-1α signaling pathway; Up-regulated the expression of Bcl-2 and down-regulated the expression of Bax and caspase 3 | 80 mg/kg/day | 4 | (81) |
ICAII | 2014 | Rat with streptozotocin | In vivo | Increased epididymal sperm parameters and testicular Johnsen’s scores | Increased antioxidant enzyme activities and the expression of Sertoli cell Vimentin filaments, and | 0.5, 1.5 or 4.5 mg/kg/day | 4 | (82) |
ICA | 2022 | Mice and Leydig cells with (2-Ethylhexyl) Phthalate | In vivo and in vitro | Promotes testosterone synthesis | Activated Esr1/Src/Akt/Creb/Sf-1 signaling pathway | 100 mg/kg/day in vivo; 5 μg/mL in vitro | 5 | (83) |
ICA, icariin; ICAII, icariside II; ROS, reactive oxygen species; PBR, peripheral-type benzodiazepine receptor; SF-1, Steroidogenic factor-1; CYP11, Cytochrome P450 Family 11; 3β-HSD, 3-beta (β)-hydroxysteroid dehydrogenase; 17β-HSD, 3-beta (β)-hydroxysteroid dehydrogenase; STAIR list, The Initial Stroke Therapy Academic Industry Roundtable (Evaluation tools commonly used in quality assessment of animal experiments); PCNA, proliferating cell nuclear antigen; SRIT1, sirtuin 1; HIF-1α, hypoxia-inducible factor-1; Bcl-2, B-cell lymphoma-2; Bax, Bcl-2-associated X; Esr1, estrogen receptor 1; Src, Src family kinases; Akt, threonine-specific protein kinase Akt; Creb, cAMP response element binding protein.
ICA and testosterone production
In the absence of testosterone, men may have symptoms including decreased libido, erectile dysfunction, reduced muscle mass and bone density, depression, and anemia. Although testosterone supplementation therapy (TST) has been reported to be potentially effective for these conditions, TST has potential adverse effects (81). The effect of ICA and ICAII in increasing testosterone levels could be related to their ability to mitigate the effects of Leydig cell damage in a variety of testicular damaging environmental exposures (e.g., diabetes, toxic substances) (82,84,85). More importantly, ICA and ICAII directly increase the level of steroids (the raw material required for testosterone production) and the expression of several key enzymes in testosterone synthesis (86-88).
Biological effects and molecular mechanisms of ICA in alleviating Leydig cell injury
Approximately 90% of testosterone is derived from the conversion of cholesterol by testicular Leydig cells and its level is regulated by follicle stimulating hormone (FSH) and luteinizing hormone (LH). Sun and his colleagues showed the protective effect of ICA (1 µm/mL) reduced diethylhexyl phthalate (DEHP) injury-induced reactive oxygen species (ROS) levels of Leydig cells In vitro experiments, and increased mitochondrial membrane potential (ΔΨm) (82). Further study Sun et al. found that estrogen receptor 1 (Esr1)/Src family kinases (Src)/Akt/CREB/steroidogenic factor-1 (Sf-1) pathway may play an important role in ICA to protect Leydig cells from DEHP damage (83).
In addition, ICAII treatment (1.5 or 4.5 mg/kg/d for 28 days) increased superoxide dismutase (SOD), glutathione peroxidase (GPx) activity and inhibited malondialdehyde (MDA) activity, thereby attenuating diabetes, nicotine and aging on rat testicular Leydig cell damage (28,85).
Oxidative stress is one of the most important pathogenesis of testicular and penile tissue damage. Current study found that nuclear factor- e2-related factor 2 (Nrf2) could prevent ROS overexpression and accumulation (86,87). ICA can upregulate the expression of Nrf2 and its downstream heme oxygenase-1 (HO-1), nicotinamide adenine dinucleotide phosphate (NADPH), quinone oxidoreductase-1 (NQO-1) in high glucose-stimulated TM4 cells, which can increase SOD activity, decrease MDA content, and inhibit the production of ROS by high glucose stimulation. The above effects of ICA were diminished after Nrf2 knockdown treatment. These results suggest that the Nrf2 pathway is an important molecular to mediate excessive oxidative stress inhibition activity of ICA. Further study revealed that the activation of Nrf2 pathway by ICA was mainly mediated by G protein-coupled estrogen receptor (GPER), which further promoted the dissociation of Nrf2/keap1 complex and the translocation of Nrf2 to nucleus (88).
Molecular mechanism of ICA on testosterone production
In addition to protecting testicular Leydig cells, ICA plays an important regulatory role in testosterone production. Steroids are the precursors of testosterone, which are transported to the mitochondria of Leydig cells by acute regulatory protein (StAR) and then converted to testosterone through a series of cleavage and dehydrogenation reactions by enzymes including 3β-Hydroxysteroid Dehydrogenase (3β-HSD) and 17β-Hydroxysteroid Dehydrogenase (17β-HSD) (89). ICA (1 µg/mL) treatment can reverse the DEPH-induced decrease of steroid levels and expression of StAR, 3β-HSD, 17β-HSD, and SF-1 in Leydig cells, enhancing testosterone synthesis (82).
In addition, the cGMP/PKG signaling pathway in Leydig cells is involved in the regulation of steroidogenic activity. It has been demonstrated that cGMP and protein kinase G (PKG) promote phosphorylation of StAR protein involved in testosterone synthesis, which can be inhibited by PDE5 (90). Therefore, the activation of cGMP and PKG by ICA and ICA derivatives and the inhibition of PDE5 may also be a mechanism for the regulation of testosterone production.
Summary
Researches in recent years have demonstrated the therapeutic effects of ICA, ICAII (an ICA metabolite isolated from epimedium herb) and its synthetic derivative YS-10 on ED, including inhibition of PDE5 enzymatic activity, promotion of testosterone production, and modulation of endogenous stem cells to promote regeneration of damaged penile tissues. As novel candidate agents for regenerative medicine on ED, the ICA, ICAII and YS-10 displayed bright application and research prospects. There are also several aspects that need to be studied in future research: (I) Flavonoids are generally insoluble in water, and experiments usually require the use of toxic organic solvents such as DMSO, so chemical modifications are also needed to increase the solubility of ICA and its derivatives; (II) The safety of the chemically modified compounds and the possible metabolic pathways need to be investigated; (III) ICA and ICA derivatives increase the number of penile stem cells and may also be the result of recruitment of stem cells from other sources, which warrants further investigation; (IV) ICA has been used for efficacy and mechanism studies in a variety of diseases, but no human data is currently available. This is likely to be the most important direction for future research. High quality and large sample of clinical and evidence-based medicine studies are recommended while ensuring safety.
Acknowledgments
Funding: This work was supported and funded by the National Natural Science Foundation of China (grant No. 81971379).
Footnote
Reporting Checklist: The authors have completed the Narrative Review reporting checklist. Available at https://tau.amegroups.com/article/view/10.21037/tau-22-232/rc
Peer Review File: Available at https://tau.amegroups.com/article/view/10.21037/tau-22-232/prf
Conflicts of Interest: All authors have completed the ICMJE uniform disclosure form (available at https://tau.amegroups.com/article/view/10.21037/tau-22-232/coif). The authors have no conflicts of interest to declare.
Ethical Statement: The authors are accountable for all aspects of the work in ensuring that questions related to the accuracy or integrity of any part of the work are appropriately investigated and resolved.
Open Access Statement: This is an Open Access article distributed in accordance with the Creative Commons Attribution-NonCommercial-NoDerivs 4.0 International License (CC BY-NC-ND 4.0), which permits the non-commercial replication and distribution of the article with the strict proviso that no changes or edits are made and the original work is properly cited (including links to both the formal publication through the relevant DOI and the license). See: https://creativecommons.org/licenses/by-nc-nd/4.0/.
References
- Lei H, Liu J, Li H, et al. Low-intensity shock wave therapy and its application to erectile dysfunction. World J Mens Health 2013;31:208-14. [Crossref] [PubMed]
- Krakhotkin DV, Ralph DJ, Blecher GA, et al. Management of residual penile curvature after penile prosthesis placement. Asian J Androl 2021;23:129-34. [Crossref] [PubMed]
- Rota C, Morigi M, Imberti B. Stem Cell Therapies in Kidney Diseases: Progress and Challenges. Int J Mol Sci 2019;20:2790. [Crossref] [PubMed]
- Ma H, He X, Yang Y, et al. The genus Epimedium: an ethnopharmacological and phytochemical review. J Ethnopharmacol 2011;134:519-41. [Crossref] [PubMed]
- Zhang HF, Yang TS, Li ZZ, et al. Simultaneous extraction of epimedin A, B, C and icariin from Herba Epimedii by ultrasonic technique. Ultrason Sonochem 2008;15:376-85. [Crossref] [PubMed]
- Zhang DW, Cheng Y, Wang NL, et al. Effects of total flavonoids and flavonol glycosides from Epimedium koreanum Nakai on the proliferation and differentiation of primary osteoblasts. Phytomedicine 2008;15:55-61. [Crossref] [PubMed]
- Zhang DC, Liu JL, Ding YB, et al. Icariin potentiates the antitumor activity of gemcitabine in gallbladder cancer by suppressing NF-κB. Acta Pharmacol Sin 2013;34:301-8. [Crossref] [PubMed]
- Kapoor S. Icariin and its emerging role in the treatment of osteoporosis. Chin Med J (Engl) 2013;126:400. [PubMed]
- Liu M, Zhong C, He RX, et al. Icariin associated with exercise therapy is an effective treatment for postmenopausal osteoporosis. Chin Med J (Engl) 2012;125:1784-9. [PubMed]
- Pan Y, Wang FM, Qiang LQ, et al. Icariin attenuates chronic mild stress-induced dysregulation of the LHPA stress circuit in rats. Psychoneuroendocrinology 2010;35:272-83. [Crossref] [PubMed]
- Pan Y, Kong LD, Li YC, et al. Icariin from Epimedium brevicornum attenuates chronic mild stress-induced behavioral and neuroendocrinological alterations in male Wistar rats. Pharmacol Biochem Behav 2007;87:130-40. [Crossref] [PubMed]
- Liao H, Jacob R. Chinese herbal drugs for erectile dysfunction through NO-cGMP-PDE5 signaling pathway. Zhonghua Nan Ke Xue 2012;18:260-5. [PubMed]
- Chen Y, Zhao YH, Jia XB, et al. Intestinal absorption mechanisms of prenylated flavonoids present in the heat-processed Epimedium koreanum Nakai (Yin Yanghuo). Pharm Res 2008;25:2190-9. [Crossref] [PubMed]
- Cao YF, He RR, Cao J, et al. Drug-Drug Interactions Potential of Icariin and Its Intestinal Metabolites via Inhibition of Intestinal UDP-Glucuronosyltransferases. Evid Based Complement Alternat Med 2012;2012:395912. [Crossref] [PubMed]
- Cai WJ, Huang JH, Zhang SQ, et al. Icariin and its derivative icariside II extend healthspan via insulin/IGF-1 pathway in C. elegans. PLoS One 2011;6:e28835. [Crossref] [PubMed]
- Cheng T, Zhang Y, Zhang T, et al. Comparative Pharmacokinetics Study of Icariin and Icariside II in Rats. Molecules 2015;20:21274-86. [Crossref] [PubMed]
- Wu H, Kim M, Han J. Icariin Metabolism by Human Intestinal Microflora. Molecules 2016;21:1158. [Crossref] [PubMed]
- Xu W, Zhang Y, Yang M, et al. LC-MS/MS method for the simultaneous determination of icariin and its major metabolites in rat plasma. J Pharm Biomed Anal 2007;45:667-72. [Crossref] [PubMed]
- Liu M, Liu H, Lu X, et al. Simultaneous determination of icariin, icariside II and osthole in rat plasma after oral administration of the extract of Gushudan (a Chinese compound formulation) by LC-MS/MS. J Chromatogr B Analyt Technol Biomed Life Sci 2007;860:113-20. [Crossref] [PubMed]
- Qian Q, Li SL, Sun E, et al. Metabolite profiles of icariin in rat plasma by ultra-fast liquid chromatography coupled to triple-quadrupole/time-of-flight mass spectrometry. J Pharm Biomed Anal 2012;66:392-8. [Crossref] [PubMed]
- Chang Q, Wang GN, Li Y, et al. Oral absorption and excretion of icaritin, an aglycone and also active metabolite of prenylflavonoids from the Chinese medicine Herba Epimedii in rats. Phytomedicine 2012;19:1024-8. [Crossref] [PubMed]
- Wong SP, Shen P, Lee L, et al. Pharmacokinetics of prenylflavonoids and correlations with the dynamics of estrogen action in sera following ingestion of a standardized Epimedium extract. J Pharm Biomed Anal 2009;50:216-23. [Crossref] [PubMed]
- Ahn GJ, Sohn YS, Kang KK, et al. The effect of PDE5 inhibition on the erectile function in streptozotocin-induced diabetic rats. Int J Impot Res 2005;17:134-41. [Crossref] [PubMed]
- Zhang J, Li AM, Liu BX, et al. Effect of icarisid II on diabetic rats with erectile dysfunction and its potential mechanism via assessment of AGEs, autophagy, mTOR and the NO-cGMP pathway. Asian J Androl 2013;15:143-8. [Crossref] [PubMed]
- Xu Y, Xin H, Wu Y, et al. Effect of icariin in combination with daily sildenafil on penile atrophy and erectile dysfunction in a rat model of bilateral cavernous nerves injury. Andrology 2017;5:598-605. [Crossref] [PubMed]
- Liu T, Xin H, Li WR, et al. Effects of icariin on improving erectile function in streptozotocin-induced diabetic rats. J Sex Med 2011;8:2761-72. [Crossref] [PubMed]
- Zhang J, Li S, Zhang S, et al. Effect of Icariside II and Metformin on Penile Erectile Function, Histological Structure, Mitochondrial Autophagy, Glucose-Lipid Metabolism, Angiotensin II and Sex Hormone in Type 2 Diabetic Rats With Erectile Dysfunction. Sex Med 2020;8:168-77. [Crossref] [PubMed]
- Ni G, Zhang X, Afedo SY, et al. Evaluation of the protective effects of icariin on nicotine-induced reproductive toxicity in male mouse -a pilot study. Reprod Biol Endocrinol 2020;18:65. [Crossref] [PubMed]
- Wang H, Liu Y, Huai Q, et al. Multiple conformations of phosphodiesterase-5: implications for enzyme function and drug development. J Biol Chem 2006;281:21469-79. [Crossref] [PubMed]
- Chau Y, Li FS, Levsh O, et al. Exploration of icariin analog structure space reveals key features driving potent inhibition of human phosphodiesterase-5. PLoS One 2019;14:e0222803. [Crossref] [PubMed]
- Xin ZC, Kim EK, Lin CS, et al. Effects of icariin on cGMP-specific PDE5 and cAMP-specific PDE4 activities. Asian J Androl 2003;5:15-8. [PubMed]
- Ning H, Xin ZC, Lin G, et al. Effects of icariin on phosphodiesterase-5 activity in vitro and cyclic guanosine monophosphate level in cavernous smooth muscle cells. Urology 2006;68:1350-4. [Crossref] [PubMed]
- Zhang J, Wang YB, Ma CG, et al. Icarisid II, a PDE5 inhibitor from Epimedium wanshanense, increases cellular cGMP by enhancing NOS in diabetic ED rats corpus cavernosum tissue. Andrologia 2012;44:87-93. [Crossref] [PubMed]
- Dell'Agli M, Galli GV, Dal Cero E, et al. Potent inhibition of human phosphodiesterase-5 by icariin derivatives. J Nat Prod 2008;71:1513-7. [Crossref] [PubMed]
- Jiang Z, Hu B, Wang J, et al. Effect of icariin on cyclic GMP levels and on the mRNA expression of cGMP-binding cGMP-specific phosphodiesterase (PDE5) in penile cavernosum. J Huazhong Univ Sci Technolog Med Sci 2006;26:460-2. [Crossref] [PubMed]
- Li Y, Jiang J, He Y, et al. Icariin combined with breviscapine improves the erectile function of spontaneously hypertensive rats. J Sex Med 2014;11:2143-52. [Crossref] [PubMed]
- Li WK, Zhang RY, Xiao PG. Flavonoids from Epimedium wanshanense. Phytochemistry 1996;43:527-30. [Crossref] [PubMed]
- Fan JJ, Cao LG, Wu T, et al. The dose-effect of icariin on the proliferation and osteogenic differentiation of human bone mesenchymal stem cells. Molecules 2011;16:10123-33. [Crossref] [PubMed]
- Zhang S, Feng P, Mo G, et al. Icariin influences adipogenic differentiation of stem cells affected by osteoblast-osteoclast co-culture and clinical research adipogenic. Biomed Pharmacother 2017;88:436-42. [Crossref] [PubMed]
- Jones NC, Tyner KJ, Nibarger L, et al. The p38alpha/beta MAPK functions as a molecular switch to activate the quiescent satellite cell. J Cell Biol 2005;169:105-16. [Crossref] [PubMed]
- Zhai YK, Guo XY, Ge BF, et al. Icariin stimulates the osteogenic differentiation of rat bone marrow stromal cells via activating the PI3K-AKT-eNOS-NO-cGMP-PKG. Bone 2014;66:189-98. [Crossref] [PubMed]
- Bai GY, Zhou F, Hui Y, et al. Effects of Icariside II on corpus cavernosum and major pelvic ganglion neuropathy in streptozotocin-induced diabetic rats. Int J Mol Sci 2014;15:23294-306. [Crossref] [PubMed]
- Gu SJ, Li M, Yuan YM, et al. A novel flavonoid derivative of icariside II improves erectile dysfunction in a rat model of cavernous nerve injury. Andrology 2021;9:1893-901. [Crossref] [PubMed]
- Fu X, Li S, Zhou S, et al. Stimulatory effect of icariin on the proliferation of neural stem cells from rat hippocampus. BMC Complement Altern Med 2018;18:34. [Crossref] [PubMed]
- Huang JH, Cai WJ, Zhang XM, et al. Icariin promotes self-renewal of neural stem cells: an involvement of extracellular regulated kinase signaling pathway. Chin J Integr Med 2014;20:107-15. [Crossref] [PubMed]
- Yang P, Guan YQ, Li YL, et al. Icariin promotes cell proliferation and regulates gene expression in human neural stem cells in vitro. Mol Med Rep 2016;14:1316-22. [Crossref] [PubMed]
- Lu Q, Zhu H, Liu X, et al. Icariin sustains the proliferation and differentiation of Aβ25-35-treated hippocampal neural stem cells via the BDNF-TrkB-ERK/Akt signaling pathway. Neurol Res 2020;42:936-45. [Crossref] [PubMed]
- Zeng NX, Li HZ, Wang HZ, et al. Exploration of the mechanism by which icariin modulates hippocampal neurogenesis in a rat model of depression. Neural Regen Res 2022;17:632-42. [Crossref] [PubMed]
- Zhou F, Xin H, Liu T, et al. Effects of icariside II on improving erectile function in rats with streptozotocin-induced diabetes. J Androl 2012;33:832-44. [Crossref] [PubMed]
- Tang Y, Jacobi A, Vater C, et al. Icariin promotes angiogenic differentiation and prevents oxidative stress-induced autophagy in endothelial progenitor cells. Stem Cells 2015;33:1863-77. [Crossref] [PubMed]
- Castañares C, Redondo-Horcajo M, Magán-Marchal N, et al. Signaling by ALK5 mediates TGF-beta-induced ET-1 expression in endothelial cells: a role for migration and proliferation. J Cell Sci 2007;120:1256-66. [Crossref] [PubMed]
- Hyman KM, Seghezzi G, Pintucci G, et al. Transforming growth factor-beta1 induces apoptosis in vascular endothelial cells by activation of mitogen-activated protein kinase. Surgery 2002;132:173-9. [Crossref] [PubMed]
- Yang A, Yu C, Lu Q, et al. Mechanism of Action of Icariin in Bone Marrow Mesenchymal Stem Cells. Stem Cells Int 2019;2019:5747298. [Crossref] [PubMed]
- Ye Y, Jing X, Li N, et al. Icariin promotes proliferation and osteogenic differentiation of rat adipose-derived stem cells by activating the RhoA-TAZ signaling pathway. Biomed Pharmacother 2017;88:384-94. [Crossref] [PubMed]
- Li X, Yang HF, Chen Y, et al. Effect of the icariin on endothelial microparticles, endothelial progenitor cells, platelets, and erectile function in spontaneously hypertensive rats. Andrology 2022;10:576-84. [Crossref] [PubMed]
- Cui H, Liu Z, Wang L, et al. Icariin-treated human umbilical cord mesenchymal stem cells decrease chronic liver injury in mice. Cytotechnology 2017;69:19-29. [Crossref] [PubMed]
- Luo Z, Liu M, Sun L, et al. Icariin recovers the osteogenic differentiation and bone formation of bone marrow stromal cells from a rat model of estrogen deficiency-induced osteoporosis. Mol Med Rep 2015;12:382-8. [Crossref] [PubMed]
- Sun ZB, Wang JW, Xiao H, et al. Icariin may benefit the mesenchymal stem cells of patients with steroid-associated osteonecrosis by ABCB1-promoter demethylation: a preliminary study. Osteoporos Int 2015;26:187-97. [Crossref] [PubMed]
- Wang X, Liu C, Xu Y, et al. Combination of mesenchymal stem cell injection with icariin for the treatment of diabetes-associated erectile dysfunction. PLoS One 2017;12:e0174145. [Crossref] [PubMed]
- Xu Y, Yang Y, Zheng H, et al. Intracavernous injection of size-specific stem cell spheroids for neurogenic erectile dysfunction: Efficacy and risk versus single cells. EBioMedicine 2020;52:102656. [Crossref] [PubMed]
- Kurzrock EA, Lieu DK, Degraffenried LA, et al. Label-retaining cells of the bladder: candidate urothelial stem cells. Am J Physiol Renal Physiol 2008;294:F1415-21. [Crossref] [PubMed]
- Huang YL, Tao X, Xia J, et al. Distribution and quantity of label-retaining cells in rat oral epithelia. J Oral Pathol Med 2009;38:663-7. [Crossref] [PubMed]
- Song J, Shu L, Zhang Z, et al. Reactive oxygen species-mediated mitochondrial pathway is involved in Baohuoside I-induced apoptosis in human non-small cell lung cancer. Chem Biol Interact 2012;199:9-17. [Crossref] [PubMed]
- Ruan Y, Lin G, Kang N, et al. In Situ Activation and Preservation of Penile Progenitor Cells Using Icariside II in an Obesity-Associated Erectile Dysfunction Rat Model. Stem Cells Dev 2018;27:207-15. [Crossref] [PubMed]
- Xu Y, Guan R, Lei H, et al. Implications for differentiation of endogenous stem cells: therapeutic effect from icariside II on a rat model of postprostatectomy erectile dysfunction. Stem Cells Dev 2015;24:747-55. [Crossref] [PubMed]
- Ding L, Liang XG, Zhu DY, et al. Icariin promotes expression of PGC-1alpha, PPARalpha, and NRF-1 during cardiomyocyte differentiation of murine embryonic stem cells in vitro. Acta Pharmacol Sin 2007;28:1541-9. [Crossref] [PubMed]
- Qin S, Zhou W, Liu S, et al. Icariin stimulates the proliferation of rat bone mesenchymal stem cells via ERK and p38 MAPK signaling. Int J Clin Exp Med 2015;8:7125-33. [PubMed]
- Fu S, Yang L, Hong H, et al. Wnt/β-catenin signaling is involved in the icariin induced proliferation of bone marrow mesenchymal stem cells. Journal of Traditional Chinese Medicine 2016;36:360-8. [Crossref] [PubMed]
- Luo G, Xu B, Huang Y. Icariside II promotes the osteogenic differentiation of canine bone marrow mesenchymal stem cells via the PI3K/AKT/mTOR/S6K1 signaling pathways. Am J Transl Res 2017;9:2077-87. [PubMed]
- Lin G, Alwaal A, Zhang X, et al. Presence of stem/progenitor cells in the rat penis. Stem Cells Dev 2015;24:264-70. [Crossref] [PubMed]
- Chen S, Wang Z, Zhou H, et al. Icariin reduces high glucose-induced endothelial progenitor cell dysfunction via inhibiting the p38/CREB pathway and activating the Akt/eNOS/NO pathway. Exp Ther Med 2019;18:4774-80. [Crossref] [PubMed]
- Hu Y, Liu K, Yan M, et al. Icariin inhibits oxidized low-density lipoprotein-induced proliferation of vascular smooth muscle cells by suppressing activation of extracellular signal-regulated kinase 1/2 and expression of proliferating cell nuclear antigen. Mol Med Rep 2016;13:2899-903. [Crossref] [PubMed]
- Hsu GL, Brock G, von Heyden B, et al. The distribution of elastic fibrous elements within the human penis. Br J Urol 1994;73:566-71. [Crossref] [PubMed]
- Verrecchia F, Chu ML, Mauviel A. Identification of novel TGF-beta /Smad gene targets in dermal fibroblasts using a combined cDNA microarray/promoter transactivation approach. J Biol Chem 2001;276:17058-62. [Crossref] [PubMed]
- Moreland RB. Is there a role of hypoxemia in penile fibrosis: a viewpoint presented to the Society for the Study of Impotence. Int J Impot Res 1998;10:113-20. [Crossref] [PubMed]
- Verrecchia F, Mauviel A. Control of connective tissue gene expression by TGF beta: role of Smad proteins in fibrosis. Curr Rheumatol Rep 2002;4:143-9. [Crossref] [PubMed]
- McNairn AJ, Brusadelli M, Guasch G. Signaling moderation: TGF-beta in exocrine gland development, maintenance, and regulation. Eur J Dermatol 2013; [Crossref] [PubMed]
- Yamagishi S, Inagaki Y, Okamoto T, et al. Advanced glycation end products inhibit de novo protein synthesis and induce TGF-beta overexpression in proximal tubular cells. Kidney Int 2003;63:464-73. [Crossref] [PubMed]
- Xiang Y, Cai C, Wu Y, et al. Icariin Attenuates Monocrotaline-Induced Pulmonary Arterial Hypertension via the Inhibition of TGF-β1/Smads Pathway in Rats. Evid Based Complement Alternat Med 2020;2020:9238428. [Crossref] [PubMed]
- Dong C, Ming X, Ye Z, et al. Icariside II Attenuates Chronic Hydrocephalus in an Experimental Subarachnoid Hemorrhage Rat Model. J Pharm Pharm Sci 2018;21:318-25. [Crossref] [PubMed]
- Lang PO, Samaras D, Samaras N. Testosterone replacement therapy in reversing "andropause": what is the proof-of-principle?. Rejuvenation Res 2012;15:453-65. [Crossref] [PubMed]
- Sun J, Wang D, Lin J, et al. Icariin protects mouse Leydig cell testosterone synthesis from the adverse effects of di(2-ethylhexyl) phthalate. Toxicol Appl Pharmacol 2019;378:114612. [Crossref] [PubMed]
- Sun J, Xu W, Zheng S, et al. Icariin promotes mouse Leydig cell testosterone synthesis via the Esr1/Src/Akt/Creb/Sf-1 pathway. Toxicol Appl Pharmacol 2022;441:115969. [Crossref] [PubMed]
- He W, Liu H, Hu L, et al. Icariin improves testicular dysfunction via enhancing proliferation and inhibiting mitochondria-dependent apoptosis pathway in high-fat diet and streptozotocin-induced diabetic rats. Reprod Biol Endocrinol 2021;19:168. [Crossref] [PubMed]
- Xu Y, Lei H, Guan R, et al. Prophylactic protective effects and its potential mechanisms of IcarisideII on streptozotocin induced spermatogenic dysfunction. Int J Mol Sci 2014;15:16100-13. [Crossref] [PubMed]
- Loboda A, Damulewicz M, Pyza E, et al. Role of Nrf2/HO-1 system in development, oxidative stress response and diseases: an evolutionarily conserved mechanism. Cell Mol Life Sci 2016;73:3221-47. [Crossref] [PubMed]
- Jha JC, Banal C, Chow BS, et al. Diabetes and Kidney Disease: Role of Oxidative Stress. Antioxid Redox Signal 2016;25:657-84. [Crossref] [PubMed]
- Wang K, Zheng X, Pan Z, et al. Icariin Prevents Extracellular Matrix Accumulation and Ameliorates Experimental Diabetic Kidney Disease by Inhibiting Oxidative Stress via GPER Mediated p62-Dependent Keap1 Degradation and Nrf2 Activation. Front Cell Dev Biol 2020;8:559. [Crossref] [PubMed]
- Chen X, Liu YN, Zhou QH, et al. Effects of low concentrations of di-(2-ethylhexyl) and mono-(2-ethylhexyl) phthalate on steroidogenesis pathways and apoptosis in the murine leydig tumor cell line MLTC-1. Biomed Environ Sci 2013;26:986-9. [PubMed]
- Andric SA, Janjic MM, Stojkov NJ, et al. Testosterone-induced modulation of nitric oxide-cGMP signaling pathway and androgenesis in the rat Leydig cells. Biol Reprod 2010;83:434-42. [Crossref] [PubMed]