The predictive accuracy of preoperative erythrocyte count and maximum tumor diameter to maximum kidney diameter ratio in renal cell carcinoma
Introduction
Renal cell carcinoma (RCC) is the sixth and eighth most common primary cancer in men in women, respectively (1). According to cancer statistics (2), there was an estimated 73,750 new diagnoses of renal cancer and an estimated 14,830 deaths from RCC in the United States in 2020. Globally, Western countries have the highest incidence of RCC, which account for about 3% of all cancers in this region (3). Over the past 20 years, the global incidence of RCC has increased by approximately 2% per year. Furthermore, approximately 99,200 new cases and 39,100 related deaths were reported in Europe in 2018 (3).
At first diagnosis, the cancer in approximately 30% of patients with RCC has already metastasized, and in nearly 30% of patients with RCC, the cancer metastasizes even after radical surgery (4,5). Despite the development of newly targeted therapies and immune checkpoint blockades, the majority of metastatic RCCs still eventually lead to the death of patients (6-8). Therefore, the identification of prognostic factors after surgical treatment would be of great value to patients’ management.
Previous studies (9,10) have shown that a hypoxic tumor microenvironment is an important factor in promoting tumor metastasis. In renal cancer tissue, the uncontrolled growth and proliferation of tumor cells consume a large amount of nutrition and oxygen, the internal network of new blood vessels cannot be established in a timely or effective manner, and the structure and function of the neovascularization network become abnormal. Moreover, the permeability of neovascularization is high, and fluid extravasation into tissue space leads to increased blood flow viscosity resistance (11-13). These factors reduce the blood flow inside the tumor, the oxygen supply is far below the oxygen demand, and the tumor cells enter a state of hypoperfusion deficiency or diffusion disorder hypoxia, which become especially pronounced in larger tumors (14,15). A series of methods (16-18) have been used to detect hypoxia in tumor tissues, including inserting probes into the tumor for oxygen pressure detection, inputting antibodies to exogenous hypoxia markers into the patient before surgical resection of the tumor for detection through tumor sections after surgery, and imaging detection, but these methods have the following disadvantages including with invasive operation, the process is complicated and the cost is high.
As a component of the erythrocyte, hemoglobin performs oxygen transport by reverse-binding to oxygen. Numerous studies have confirmed a low hemoglobin level to be a risk factor for the poor prognosis of renal cancer (19,20). In addition, by virtue of the structure and function of erythrocytes, information can be more easily drawn from analysis of the erythrocyte count than from hemoglobin. Like other solid tumors, RCC can produce mechanical compressive stress in the tumor when the tumor grows and develops in a confined space (21,22). Meanwhile, as the tumor grows and presses on the surrounding normal kidney tissue, it can also come under external pressure. These mechanical stresses can further exacerbate the local hypoxic microenvironment of the tumor (23-25). As patients’ tumors vary in size and biology, so does the internal pressure of the tumor; similarly, as the length of the renal diameter lines varies across patients, so does the external pressure from the surrounding normal kidney tissue. This study is the first to report on the relationship between erythrocyte count, maximum tumor diameter to maximum kidney diameter ratio (TKR), and the prognosis of RCC through analysis of clinically available data. We present the following article in accordance with the STARD reporting checklist (available at https://tau.amegroups.com/article/view/10.21037/tau-22-414/rc).
Methods
Case selection
In our preliminary study (26), we retrospectively studied the clinicopathological epidemiological characteristics of 2,154 patients diagnosed with RCC in Shanxi Province from 2005 to 2014. After screening was conducted according to the completeness of case data and the rate of loss to follow-up, data from 295 patients with complete case data and follow-up records diagnosed in the First Hospital of Shanxi Medical University from 2010 to 2014 were collected for this study. The study was conducted in accordance with the Declaration of Helsinki (as revised in 2013). The study was approved by the Ethics Committee of the First Hospital of Shanxi Medical University, China (No. 2021K034). Written informed consent was obtained from all patients prior to the commencement of the study. All patient information was anonymized and de-identified prior to analysis.
Management and follow-up
All surgeries were performed by chief physicians with more than 10 years of independent operating experience. According to the follow-up results of patients, patients with metastasis or death caused by RCC were defined as the group with poor prognosis, while those who did not experience these outcomes were defined as the good prognosis group. Detailed clinicopathological data, including length of each diameter line of the tumor and kidney, erythrocyte count (first test results after admission), and tumor stage and metastasis, were collected statistically. All patients were followed up via telephone interviews or outpatient reviews. All information was recorded on the data collection form designed for this study, and the data were collected separately by 2 investigators who were trained in the same manner of RCC diagnosis and grading criteria.
Statistical analysis
After being input into Excel (Microsoft Corp.), the clinicopathological parameter data were analyzed by SPSS 22.0 statistical software (IBM Corp.). A chi-square test was used to compare qualitative data, including the constituent ratio/rate across the different groups. Non-normal distribution quantitative data were compared by rank-sum test. A receiver operating characteristic (ROC) curve was used to find the appropriate cutoff value, with the Youden index being equal to the sensitivity value plus the specificity value minus 1. The test level of P<0.05 was considered statistically significant.
Results
General patient characteristics
Of the 295 included patients, 199 (67.5%) were male, 96 (32.5%) were female, and the mean age was 56.45±11.03 years. There were 174 patients (59.0%) who had a poor prognosis (metastasis or death caused by RCC) and 121 patients (41.0%) who had a good prognosis. The incidence of poor prognosis was 59.3% in males (118/199) and 58.3% in females (56/96), but this did not constitute a significant difference between the sexes (χ2=0.025; P=0.875).
ROC curve analysis of erythrocyte count for the prognosis of patients with RCC
The cutoff value of the erythrocyte count was analyzed by the ROC curve method. The area under the curve (AUC) of erythrocyte count for predicting the prognosis of RCC was 0.672 (SD 0.031; P<0.001; Figure 1). When the cutoff value was 3.975, the highest Youden index was 0.309, and the corresponding sensitivity and specificity were 0.826 and 0.483, respectively (Table 1). The 295 patients were divided into 2 groups, with the lower limit of the erythrocyte count normal range [(3.8–5.1)×1012/L] being the cutoff value. According to the χ2 test, the detection rate of poor prognosis in patients with an erythrocyte count greater than or less than the lower limit of the normal range was 52.8% and 82.8%, respectively, and the difference was statistically significant (χ2=19.183; P<0.001).
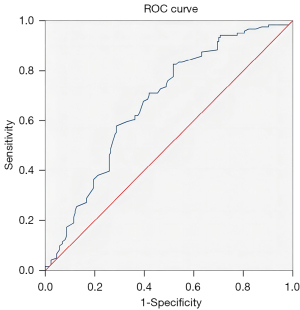
Table 1
Cut-off value | Sensitivity | 1-specificity | Specificity | Youden index |
---|---|---|---|---|
2.030 | 0.983 | 0.983 | 0.017 | 0.001 |
2.280 | 0.983 | 0.960 | 0.040 | 0.024 |
2.370 | 0.983 | 0.937 | 0.063 | 0.047 |
2.600 | 0.983 | 0.908 | 0.092 | 0.075 |
2.865 | 0.983 | 0.902 | 0.098 | 0.081 |
2.985 | 0.975 | 0.902 | 0.098 | 0.073 |
3.835 | 0.884 | 0.695 | 0.305 | 0.189 |
3.850 | 0.876 | 0.632 | 0.368 | 0.244 |
3.865 | 0.860 | 0.632 | 0.368 | 0.227 |
3.885 | 0.835 | 0.552 | 0.448 | 0.283 |
3.905 | 0.835 | 0.546 | 0.454 | 0.289 |
3.925 | 0.835 | 0.540 | 0.460 | 0.294 |
3.950 | 0.826 | 0.529 | 0.471 | 0.298 |
3.975 | 0.826 | 0.517 | 0.483 | 0.309* |
4.000 | 0.810 | 0.517 | 0.483 | 0.293 |
4.015 | 0.802 | 0.517 | 0.483 | 0.284 |
4.025 | 0.793 | 0.517 | 0.483 | 0.276 |
4.035 | 0.785 | 0.517 | 0.483 | 0.268 |
5.120 | 0.149 | 0.086 | 0.914 | 0.063 |
5.140 | 0.140 | 0.086 | 0.914 | 0.054 |
5.160 | 0.124 | 0.080 | 0.920 | 0.044 |
5.185 | 0.116 | 0.069 | 0.931 | 0.047 |
*, the highest Youden index value.
ROC curve analysis of TKR for the prognosis of patients with RCC
The cutoff value of the TKR was analyzed with the ROC curve method. The AUC of TKR for predicting the prognosis of RCC was 0.800 (SD 0.030; P<0.001; Figure 2). When the cutoff value was 0.452, the highest Youden index was 0.685, and the corresponding sensitivity and specificity were 0.685 and 1.000, respectively (Table 2).
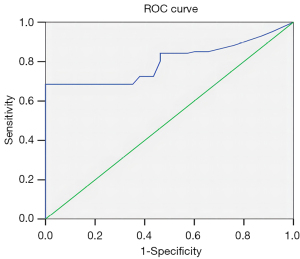
Table 2
Cut-off value | Sensitivity | 1-specificity | Specificity | Youden index |
---|---|---|---|---|
0.1066 | 0.984 | 0.972 | 0.028 | 0.012 |
0.1432 | 0.969 | 0.944 | 0.056 | 0.025 |
0.1519 | 0.953 | 0.917 | 0.083 | 0.036 |
0.1652 | 0.929 | 0.870 | 0.130 | 0.059 |
0.1775 | 0.921 | 0.852 | 0.148 | 0.069 |
0.1793 | 0.913 | 0.833 | 0.167 | 0.080 |
0.4045 | 0.685 | 0.241 | 0.759 | 0.444 |
0.4129 | 0.685 | 0.213 | 0.787 | 0.472 |
0.4226 | 0.685 | 0.157 | 0.843 | 0.528 |
0.4330 | 0.685 | 0.056 | 0.944 | 0.629 |
0.4410 | 0.685 | 0.037 | 0.963 | 0.648 |
0.4472 | 0.685 | 0.019 | 0.981 | 0.666 |
0.4523 | 0.685 | 0 | 1 | 0.685* |
0.458 | 0.638 | 0 | 1 | 0.638 |
0.4808 | 0.598 | 0 | 1 | 0.598 |
0.5192 | 0.228 | 0 | 1 | 0.228 |
0.5955 | 0.118 | 0 | 1 | 0.118 |
0.6077 | 0.102 | 0 | 1 | 0.102 |
*, the highest Youden index value.
Discussion
RCC is one of the 10 most common cancers worldwide (1,27), accounts for about 3% of all malignancies, and is also the most lethal urinary cancer, with approximately 403,200 newly diagnosed cases and 175,000 deaths annually (1). Moreover, 30% of patients with kidney cancer confined to the organ develop generalized disease even after surgical treatment (4,5). Given these facts, it is critical that the prognostic factors for RCC be identified.
Previous studies have shown that a hypoxic tumor microenvironment is an important factor in promoting tumor metastasis (9,10,28). An oxygen concentration in tissues less than 1% (PO2 <7 mmHg) leads to inhibited cell proliferation, promotes cell differentiation, and induces cell necrosis and apoptosis (29). Additionally, hypoxia, as a stress factor, may cause adaptive changes in the cells within the tumor, for instance, by changing the expression of hypoxia-inducible factor-1 (HIF-1) and other related genes to produce a more aggressive phenotype. This in turn leads to increased cell invasion, proliferation, and resistance to apoptosis, thus increasing the likelihood of metastasis (30-33). HIF-1, first discovered by Semenza in 1991 (34), is a DNA sequence of great significance in hypoxia response. It is composed of HIF-1α and HIF-1β. The expression of HIF-1β is stable in cells, while the expression of HIF-1α is closely related to the level of oxygen in cells. The expression and degradation of HIF-1α are in dynamic balance in a non-hypoxic state, while the expression of HIF-1α is increased and degradation is decreased in a hypoxic state. In a hypoxic microenvironment, HIF-1α in the cytoplasm enters the nucleus and forms the HIF-1 complex with HIF-1β through the specific basic helix-loop-helix/Per-ARNT-SIM (bHLH-PAS) domain. Subsequently, HIF-1α binds to the hypoxic reaction element (HRE) in the promoter region of the HIF-1α downstream target gene by recruiting transcription activation factors, such as AMP and cap-binding protein, thus regulating the transcription of related genes (35-37).
One such factor is erythropoietin (EPO). Many studies have confirmed the overexpression of EPO and erythropoietin receptor (EPOR) in RCC. EPO is a major regulator of erythropoiesis in tissues involved in erythrocyte production. In cancer cells, EPO may inhibit apoptosis, stimulate angiogenesis, and promote cell proliferation (38,39). Considering the functional characteristic of EPO, we speculated that elevated EPO levels in patients with RCC would be associated with polycythemia. In practice, however, anemia is observed in up to 35% of patients with RCC, and paraneoplastic polycythemia occurs in fewer than 5% of patients (19,40). Possible mechanisms for the low incidence of paraneoplastic polycythemia (38,41,42) include the low activity of EPO produced by tumors or tissues involved in blood production being less sensitive to the factor. In addition, the breakdown of tumor cells in the microvessels and a low or abnormal iron metabolism may also be important contributors. Meanwhile, the growth of inflammatory factors and antibodies during carcinogenesis may also lead to autoimmune hemolysis. These phenomena suggest that reduced erythrocyte count may be a poor prognostic factor for RCC. Alternatively, low hemoglobin is also considered to be a risk factor for poor prognosis of renal cancer, which has been confirmed by previous studies (19,20,43). Hemoglobin, as a component of erythrocyte, facilitates oxygen transport by reverse-binding with oxygen. With this in mind, we speculated that erythrocyte count could also be used as a prognostic indicator of RCC. In addition, given the specific cell structure and function, erythrocyte contains far more information than does hemoglobin.
The ROC curve method was used to determine the optimal erythrocyte cutoff value. The results showed that when the highest Youden index was 0.309, the corresponding cutoff value was 3.975, which was slightly higher than the erythrocyte lower limit of 3.8. This may be due to the renal cancer cells in the hypoxic microenvironment being more sensitive to the hypoxic condition. According to the χ2 test, the detection rates of poor prognosis in patients with erythrocyte count greater than or less than 3.8×1012/L were 52.8% and 82.8%, respectively, and the difference was statistically significant (χ2=19.183; P<0.001). These results suggest that an erythrocyte count less than 3.975×1012/L can be used as a risk factor for predicting the unfavorable prognosis of RCC.
As a solid tumor, RCC is an abnormal tissue consisting of cancer cells and various host cells embedded in the extracellular matrix that are nourished by blood vessels and drained by lymphatic vessels (44). The growth and development of solid tumors in a confined space can generate mechanical compressive stress within the tumor; meanwhile, as the growing tumor deforms normal tissue, it receives external pressure. These mechanical forces within the tumor and between the tumor and host tissue can compress blood vessels and lymphatics, reduce blood perfusion to tissues, and cause hypoxia; furthermore, when these mechanical forces act directly on cancer cells, they can increase their invasion and metastasis potential (23-25,45). Moreover, newly formed tumor blood vessels, while nourishing the tumor tissue, are often themselves leaky and curved, further reducing tissue perfusion (11,12).
In a hypoxic tumor microenvironment, tumor cells can activate a series of signal transduction pathways that may alter metabolic patterns, inhibit the antitumor effect of immune cells, or facilitate the activation of drug-resistant genes. Meanwhile, vascular leakage and compression lead to increased fluid pressure between tissues, which can impede the delivery of blood-derived therapeutic drugs (9,46,47). All of these factors may reduce the efficacy of various therapies and contribute to the progression and metastasis of malignant tumors (23,24,44,48).
In general, the tumor tissue is subject ted to internal and external pressure during the process of tumor proliferation, with the tumor itself causing internal pressure and the surrounding normal kidney tissue exerting external pressure. As the tumor grows in size, the internal pressure within it increases. Regarding the external pressure, as each patient's tumor size and kidney differs in diameter, so does the degree of this external pressure. Therefore, we tried to comprehensively evaluate the tumor pressure by combining tumor size and kidney diameter. We analyzed the ratio of the maximum diameter of the tumor to the upper and lower pole, anteroposterior diameter, and left and right diameter of the kidney, respectively. We then performed a rank-sum test according to the different prognoses and found that the mean rank difference of the TKR was statistically significant. We subsequently used the ROC curve method and found that the cutoff value was 0.452, suggesting that a TKR greater than 0.452 could be used as a risk factor for predicting the unfavorable prognosis of RCC.
In conclusion, our study demonstrated that an erythrocyte count <3.975×1012/L and a TKR >0.452 are risk factors for poor prognosis in RCC patients. It is worth mentioning that these clinicopathological parameters related to the prognosis of RCC were extracted from our readily available clinical data, which are simple, cost-effective to procure, and easy to apply. To our knowledge, this is the first large-cohort study to demonstrate the significance of the TKR in the prognosis of RCC.
However, some limitations to our study should also be mentioned. First, this is a retrospective analysis, and a prospective multi-institution study involving a large number of RCC patients with external validation is needed to further validate the current data. Second, although we found erythrocyte count and TKR to be associated with the prognosis of RCC patients, which may be related to the hypoxic microenvironment of RCC, the exact mechanisms underlying this association remain unclear. In ensuing studies, we will explore the potential mechanisms through cell function experiments and the construction of corresponding animal models.
Acknowledgments
Funding: This study was supported by the Shanxi Scholarship Council of China (No. 2021-161), the Youth Project of Basic Research Program of Shanxi Province (No. 202103021223431), the Shanxi Provincial Basic Applied Research Project (No. 201801D121331), and the Scientific Research Program of Shanxi Provincial Health Commission (No. 2017035). The funders had no role in study design, data collection and analysis, decision to publish, or preparation of the manuscript.
Footnote
Reporting Checklist: The authors have completed the STARD reporting checklist. Available at https://tau.amegroups.com/article/view/10.21037/tau-22-414/rc
Data Sharing Statement: Available at https://tau.amegroups.com/article/view/10.21037/tau-22-414/dss
Conflicts of Interest: All authors have completed the ICMJE uniform disclosure form (available at https://tau.amegroups.com/article/view/10.21037/tau-22-414/coif). The authors have no conflicts of interest to declare.
Ethical Statement: The authors are accountable for all aspects of the work in ensuring that questions related to the accuracy or integrity of any part of the work are appropriately investigated and resolved. The study was conducted in accordance with the Declaration of Helsinki (as revised in 2013). The study was approved by the Ethics Committee of the First Hospital of Shanxi Medical University, China (No. 2021K034). Written informed consent was obtained from all patients prior to the commencement of the study. All patient information was anonymized and de-identified prior to analysis.
Open Access Statement: This is an Open Access article distributed in accordance with the Creative Commons Attribution-NonCommercial-NoDerivs 4.0 International License (CC BY-NC-ND 4.0), which permits the non-commercial replication and distribution of the article with the strict proviso that no changes or edits are made and the original work is properly cited (including links to both the formal publication through the relevant DOI and the license). See: https://creativecommons.org/licenses/by-nc-nd/4.0/.
References
- Bray F, Ferlay J, Soerjomataram I, et al. Global cancer statistics 2018: GLOBOCAN estimates of incidence and mortality worldwide for 36 cancers in 185 countries. CA Cancer J Clin 2018;68:394-424. [Crossref] [PubMed]
- Siegel RL, Miller KD, Jemal A. Cancer statistics, 2020. CA Cancer J Clin 2020;70:7-30. [Crossref] [PubMed]
- Ferlay J, Colombet M, Soerjomataram I, et al. Cancer incidence and mortality patterns in Europe: Estimates for 40 countries and 25 major cancers in 2018. Eur J Cancer 2018;103:356-87. [Crossref] [PubMed]
- Gupta K, Miller JD, Li JZ, et al. Epidemiologic and socioeconomic burden of metastatic renal cell carcinoma (mRCC): a literature review. Cancer Treat Rev 2008;34:193-205. [Crossref] [PubMed]
- Athar U, Gentile TC. Treatment options for metastatic renal cell carcinoma: a review. Can J Urol 2008;15:3954-66. [PubMed]
- Mollica V, Di Nunno V, Gatto L, et al. Novel Therapeutic Approaches and Targets Currently Under Evaluation for Renal Cell Carcinoma: Waiting for the Revolution. Clin Drug Investig 2019;39:503-19. [Crossref] [PubMed]
- Haibe Y, El Husseini Z, El Sayed R, et al. Resisting Resistance to Immune Checkpoint Therapy: A Systematic Review. Int J Mol Sci 2020;21:6176. [Crossref] [PubMed]
- Kasherman L, Siu DHW, Woodford R, et al. Angiogenesis Inhibitors and Immunomodulation in Renal Cell Cancers: The Past, Present, and Future. Cancers (Basel) 2022;14:1406. [Crossref] [PubMed]
- Petrova V, Annicchiarico-Petruzzelli M, Melino G, et al. The hypoxic tumour microenvironment. Oncogenesis 2018;7:10. [Crossref] [PubMed]
- Wang B, Zhao Q, Zhang Y, et al. Targeting hypoxia in the tumor microenvironment: a potential strategy to improve cancer immunotherapy. J Exp Clin Cancer Res 2021;40:24. [Crossref] [PubMed]
- Semenza GL. Hypoxia-inducible factors: mediators of cancer progression and targets for cancer therapy. Trends Pharmacol Sci 2012;33:207-14. [Crossref] [PubMed]
- Eales KL, Hollinshead KE, Tennant DA. Hypoxia and metabolic adaptation of cancer cells. Oncogenesis 2016;5:e190. [Crossref] [PubMed]
- Zheng R, Li F, Li F, et al. Targeting tumor vascularization: promising strategies for vascular normalization. J Cancer Res Clin Oncol 2021;147:2489-505. [Crossref] [PubMed]
- Lugano R, Ramachandran M, Dimberg A. Tumor angiogenesis: causes, consequences, challenges and opportunities. Cell Mol Life Sci 2020;77:1745-70. [Crossref] [PubMed]
- Carraway RE, Cochrane DE. Enhanced vascular permeability is hypothesized to promote inflammation-induced carcinogenesis and tumor development via extravasation of large molecular proteins into the tissue. Med Hypotheses 2012;78:738-43. [Crossref] [PubMed]
- Ryniawec JM, Coope MR, Loertscher E, et al. GLUT3/SLC2A3 Is an Endogenous Marker of Hypoxia in Prostate Cancer Cell Lines and Patient-Derived Xenograft Tumors. Diagnostics (Basel) 2022;12:676. [Crossref] [PubMed]
- Huang Y, Fan J, Li Y, et al. Imaging of Tumor Hypoxia With Radionuclide-Labeled Tracers for PET. Front Oncol 2021;11:731503. [Crossref] [PubMed]
- Purkayastha P, Jaiswal MK, Lele TP. Molecular cancer cell responses to solid compressive stress and interstitial fluid pressure. Cytoskeleton (Hoboken) 2021;78:312-22. [Crossref] [PubMed]
- Prokopowicz G, Życzkowski M, Nowakowski K, et al. Basic Parameters of Blood Count as Prognostic Factors for Renal Cell Carcinoma. Biomed Res Int 2016;2016:8687575. [Crossref] [PubMed]
- Shin D, Jeong CW, Song C, et al. Prognostic factors for overall survival in patients with clear cell metastatic renal cell carcinoma: Model development and external validation with Memorial Sloan Kettering Cancer Center model and the international metastatic renal cell carcinoma database consortium model. Medicine (Baltimore) 2021;100:e26826. [Crossref] [PubMed]
- Gensbittel V, Kräter M, Harlepp S, et al. Mechanical Adaptability of Tumor Cells in Metastasis. Dev Cell 2021;56:164-79. [Crossref] [PubMed]
- Grossen A, Smith K, Coulibaly N, et al. Physical Forces in Glioblastoma Migration: A Systematic Review. Int J Mol Sci 2022;23:4055. [Crossref] [PubMed]
- Jain RK, Martin JD, Stylianopoulos T. The role of mechanical forces in tumor growth and therapy. Annu Rev Biomed Eng 2014;16:321-46. [Crossref] [PubMed]
- Tse JM, Cheng G, Tyrrell JA, et al. Mechanical compression drives cancer cells toward invasive phenotype. Proc Natl Acad Sci U S A 2012;109:911-6. [Crossref] [PubMed]
- Northcott JM, Dean IS, Mouw JK, et al. Feeling Stress: The Mechanics of Cancer Progression and Aggression. Front Cell Dev Biol 2018;6:17. [Crossref] [PubMed]
- Bai T, Wang L, Wang D, et al. Clinicopathologica Epidemiological Characteristics and Change Tendencies of Renal Cell Carcinoma in Shanxi Province of China from 2005 to 2014. PLoS One 2015;10:e0144246. [Crossref] [PubMed]
- Hsieh JJ, Purdue MP, Signoretti S, et al. Renal cell carcinoma. Nat Rev Dis Primers 2017;3:17009. [Crossref] [PubMed]
- Muz B, de la Puente P, Azab F, et al. The role of hypoxia in cancer progression, angiogenesis, metastasis, and resistance to therapy. Hypoxia (Auckl) 2015;3:83-92. [Crossref] [PubMed]
- Vaupel P. Hypoxia and aggressive tumor phenotype: implications for therapy and prognosis. Oncologist 2008;13:21-6. [Crossref] [PubMed]
- Rouschop KM, van den Beucken T, Dubois L, et al. The unfolded protein response protects human tumor cells during hypoxia through regulation of the autophagy genes MAP1LC3B and ATG5. J Clin Invest 2010;120:127-41. [Crossref] [PubMed]
- Infantino V, Santarsiero A, Convertini P, et al. Cancer Cell Metabolism in Hypoxia: Role of HIF-1 as Key Regulator and Therapeutic Target. Int J Mol Sci 2021;22:5703. [Crossref] [PubMed]
- Ma Z, Wang LZ, Cheng JT, et al. Targeting Hypoxia-Inducible Factor-1-Mediated Metastasis for Cancer Therapy. Antioxid Redox Signal 2021;34:1484-97. [Crossref] [PubMed]
- Emami Nejad A, Najafgholian S, Rostami A, et al. The role of hypoxia in the tumor microenvironment and development of cancer stem cell: a novel approach to developing treatment. Cancer Cell Int 2021;21:62. [Crossref] [PubMed]
- Semenza GL, Nejfelt MK, Chi SM, et al. Hypoxia-inducible nuclear factors bind to an enhancer element located 3' to the human erythropoietin gene. Proc Natl Acad Sci U S A 1991;88:5680-4. [Crossref] [PubMed]
- Masoud GN, Li W. HIF-1α pathway: role, regulation and intervention for cancer therapy. Acta Pharm Sin B 2015;5:378-89. [Crossref] [PubMed]
- Ziello JE, Jovin IS, Huang Y. Hypoxia-Inducible Factor (HIF)-1 regulatory pathway and its potential for therapeutic intervention in malignancy and ischemia. Yale J Biol Med 2007;80:51-60. [PubMed]
- Kumar A, Vaish M, Karuppagounder SS, et al. HIF1α stabilization in hypoxia is not oxidant-initiated. Elife 2021;10:72873. [Crossref]
- Morais C, Johnson DW, Vesey DA, et al. Functional significance of erythropoietin in renal cell carcinoma. BMC Cancer 2013;13:14. [Crossref] [PubMed]
- Tsiftsoglou AS. Erythropoietin (EPO) as a Key Regulator of Erythropoiesis, Bone Remodeling and Endothelial Transdifferentiation of Multipotent Mesenchymal Stem Cells (MSCs): Implications in Regenerative Medicine. Cells 2021;10:2140. [Crossref] [PubMed]
- Westenfelder C, Baranowski RL. Erythropoietin stimulates proliferation of human renal carcinoma cells. Kidney Int 2000;58:647-57. [Crossref] [PubMed]
- Arfin S, Jha NK, Jha SK, et al. Oxidative Stress in Cancer Cell Metabolism. Antioxidants (Basel) 2021;10:642. [Crossref] [PubMed]
- Hao X, Ren Y, Feng M, et al. Metabolic reprogramming due to hypoxia in pancreatic cancer: Implications for tumor formation, immunity, and more. Biomed Pharmacother 2021;141:111798. [Crossref] [PubMed]
- Grivas N, Kafarakis V, Tsimaris I, et al. Clinico-pathological prognostic factors of renal cell carcinoma: A 15-year review from a single center in Greece. Urol Ann 2014;6:116-21. [Crossref] [PubMed]
- Jain RK. Normalizing tumor microenvironment to treat cancer: bench to bedside to biomarkers. J Clin Oncol 2013;31:2205-18. [Crossref] [PubMed]
- Levayer R. Solid stress, competition for space and cancer: The opposing roles of mechanical cell competition in tumour initiation and growth. Semin Cancer Biol 2020;63:69-80. [Crossref] [PubMed]
- Al Tameemi W, Dale TP, Al-Jumaily RMK, et al. Hypoxia-Modified Cancer Cell Metabolism. Front Cell Dev Biol 2019;7:4. [Crossref] [PubMed]
- Jing X, Yang F, Shao C, et al. Role of hypoxia in cancer therapy by regulating the tumor microenvironment. Mol Cancer 2019;18:157. [Crossref] [PubMed]
- Abou Khouzam R, Brodaczewska K, Filipiak A, et al. Tumor Hypoxia Regulates Immune Escape/Invasion: Influence on Angiogenesis and Potential Impact of Hypoxic Biomarkers on Cancer Therapies. Front Immunol 2021;11:613114. [Crossref] [PubMed]