A scoping review of penile implant biofilms—what do we know and what remains unknown?
Introduction
Due to its long-term durability and high rates of patient and partner satisfaction, penile prosthesis (PP) implantation is now regarded as a gold standard treatment for medically refractory erectile dysfunction (ED) (1,2). According to the American Urological Association guidelines, PP can be considered as a first-line treatment option for ED, which differs from the previously recommended stepwise approach (3). Recent reviews have suggested that PP implantations may not only be the most effective treatment for ED, but also the most cost effective compared to other medical therapies in specific populations, such as those after ischemic priapism (4-6). With the growing body of literature surrounding the efficacy of PP for ED, device failure rates and surgical complications have also been well established. Of these, infection remains the most concerning sequelae, often necessitating device removal and subsequent revision surgery with suboptimal outcomes (7).
Device infections are thought to be caused by the introduction of microorganisms via incisions at the time of surgery or via hematogenous spread. Typically, the host defense mechanisms and prophylactic antibiotics kill the bacteria; however, in the setting of medical device implantation into a surgical wound, the implant is rapidly coated with serum proteins and ultimately the body deems it as a foreign body and coats it with a conditioning layer of fibrous capsule, which can alter the surface characteristics of the inanimate object (8). This serum-coated surface is ideal for bacterial adherence and subsequent biofilm formation (Figure 1). Bacterial biofilms are communities of adherent bacteria protected against the body’s immune system and antibiotics by a protein-containing polysaccharide matrix. During this process, the cells undergo phenotypic changes that render them less metabolically active and, therefore, more drug resistant (9). The risk of device infection is further increased after revision surgery due to weakened host-resistance factors, impaired wound healing related to scar formation and, most importantly, decreased antibiotic penetration secondary to bacterial biofilm formation (10).
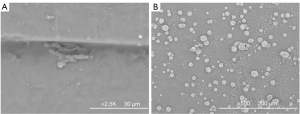
Bacterial biofilms are problematic to the prosthetic surgeon and catastrophic for the patient as they are extremely difficult to prevent or treat. Within the urologic field, biofilms can cause complications with simple devices such as urethral catheters or indwelling ureteral stents, as well as PP implants. An understanding of these biofilms and the microbes they harbor is essential to understanding the pathophysiology of device infection and malfunction. Herein, we aim to provide the readership with a scoping review of the current literature pertaining to biofilm formation in the setting of PP surgeries. We present the following article in accordance with the PRISMA-ScR reporting checklist (available at https://tau.amegroups.com/article/view/10.21037/tau-22-195/rc).
Methods
A literature review of articles indexed in the MEDLINE online database was performed through PubMed from January 2022 to February 2022. Keyword searches including a combination of the terms “penile prosthetic” OR “penile prosthesis” OR “penile implant” AND “biofilm” OR “revision” OR “removal” OR “infection” OR “explant” were utilized to identify appropriate articles to include in our review in accordance with the PRISMA Extension for Scoping Reviews protocol (11). Only original articles that were peer-reviewed and published in English were included. There was no limit placed on publication year. Article types including editorial comments, review articles or systematic reviews and meta-analysis were excluded. Articles spanned from 1953 to 2022. Titles, abstracts, and full texts were reviewed for inclusion based on appropriateness by three authors (JYL, CEC, MJD) independently. Articles were only included if they specifically identified or cultured biofilm during PP revision surgeries. Variables including presence of biofilm, microbial data (number of isolates, number of species, organism type), time to reoperation, culture sensitivities and administered antibiotics were abstracted. Upon identifying and screening the 160 eligible articles, a total of 11 articles that met inclusion criteria were found (Figure 2). We included an early case report that first described and identified biofilm in two patients with infected PP implants. We qualitatively analyzed and summarized the data from these articles and descriptively presented them in Table 1.
Table 1
First author, year | Percentage of biofilm present | Microbial data | Time to reoperation, median (range) | Culture sensitivities | Perioperative antibiotics | ||
---|---|---|---|---|---|---|---|
Number of isolates | Number of species | Organism type | |||||
Chung, 2022* | 48/83 (56%) on next-generation sequencing; 24/83 (29%) on standard culture | – | 21 | Infected: P. aeruginosa (50%); Erosion: S. epidermidis (75%); Mechanical malfunction: E. coli (72%) | 28 months (interquartile range 43.5 months) | Vancomycin + gentamicin with broadest coverage | – |
Gross, 2020* | 71% | – | – | Gram-positive bacteria (44%), Gram-negative bacteria (25%) | 2 months (2–81 months); mean 5.4 months | – | – |
Gross, 2019* | 26/26 (100%) infected implants (fungal) | 26 | 5 | Candida sp. (97%), C. albicans (62%) | 4.8 months (12–120 months) | – | In addition to standard perioperative antibiotics, no patients received antifungals at initial implantation, 15% received antifungals before explant, 31% received antifungals during explant |
Jani, 2018* | 130/236 (55%) | 127 | 27 | Staphylococcal sp. (77%), S. epidermidis (43%) | Mean 56 months (standard deviation 51 months) | All isolates sensitive to tetracycline/rifampin | – |
Gross, 2017* | 153/227 (67%) infected implants | 204 | 35 | E. coli (18%), Coagulase-negative Staphylococcal sp. (15%), Candida sp. (11%) | 1.5 months (0.5–81 months); mean 4.8 months | Vancomycin + gentamicin OR vancomycin + aztreonam (86% efficacy) | Implantation: 56% vancomycin/gentamicin, 22% ancef/gentamicin; Salvage/explant: 50% vancomycin/gentamicin, 15% ancef/gentamicin |
Ciftci, 2016 | 2/71 (3%) non-infected implants; 2/18 (11%) positive culture | 5 | 21 | S. epidermidis (57%), 2/2 biofilms cultured S. epidermidis | 41 months (8–82 months) | – | – |
Kava, 2011* | 5/51 (10%) | 7 | 6 | S. epidermidis (29%) | 9.6 months (6–138 months) | – | – |
Henry, 2008* | 97/148 (66%) non-infected implants | 124 | 20 | Staphylococcal sp. (87%), S. epidermidis (44%) | Mean 47.9 months (range 1–190 months) | – | – |
Silverstein, 2006 | 7/10 (70%) non-infected implants; 7/8 (88%) positive culture | – | – | Gram-positive cocci (80%), Gram-negative rods (70%), Yeast (60%) | >2 years | – | – |
Henry, 2004* | 54/77 (70%) non-infected implants | 64 | 15 | Staphylococcal sp. (81%), S. epidermidis (39%) | Mean 53 months (range 2–190 months) | All Staphylococcal sp. isolates sensitive to tetracycline/rifampin | – |
Nickel, 1986 | 2/2 (100%) infected implants | – | – | Coccoid bacterial cells (100%), P. aeruginosa (50%) | 1 month; 2 years | – | Cefalexin; trimethoprim-sulfamethoxazole |
*, Studies did not explicitly mention the number of biofilms identified, but noted that bacterial biofilm was swabbed and cultured if observed during salvage procedure.
Results
While there were only three studies that explicitly listed the number of biofilms identified in their cohort, we also included eight others that mentioned the swabbing and culturing of any bacterial biofilm during revision procedures for both clinically infected and non-infected implants. These results are summarized in Table 1.
The first study describing biofilms on PP was published by Nickel et al. in 1986 whereby two patients with clinically infected PP harbored rod and coccoid shaped bacterial cells (12). These patients were successfully treated with oral antibiotics. The next study by Silverstein et al. found a 70% rate of biofilm formation among non-infected, non-antibiotic coated implants, 88% of which did have positive cultures (13). Most recently in 2016, Ciftci et al. identified biofilm in 11% of implants removed for non-infectious reasons, all of which grew Staphylococcus epidermidis (14).
Next, we also noted that for non-infected implants or implants removed secondary to mechanical malfunction, the most common reported organisms were coagulase-negative staphylococcus (i.e., S. epidermidis), which ranged from 15–81% in our cohort. Conversely, implants removed for infection harbored other organisms such as P. aeruginosa in 50% of one reported cohort or E. coli in 18% of another cohort.
The percentage of biofilm presence were based on whether the PP were clinically infected or not. When PP were infected, the rate of biofilm presence ranged from 11–100%, while biofilm presence ranged from 3–70% in non-infected PP. Time to reoperation from initial PP placement were also largely variable, ranging from 2 weeks to over 2 years.
Discussion
What is biofilm?
Historically, biofilm is defined as “a structured consortium of bacteria encased in a self-producing matrix that exhibits a unique pattern of gene expression and growth” and is almost always associated with a surface for attachment (15-17). Its structure can be divided into three layers—a deep, linking layer abutting the adherent surface, a compact base layer of bacteria, and a superficial surface film on which free-floating bacteria can arise and spread (18).
Biofilm formation can be distilled down to four phases—attachment, aggregation, maturation and detachment (19). The first stage is arguably the most important step, and involves foundational forces and biological proteins from planktonic bacteria that establish interactions to adhere to the inanimate substratum (15,18). The second stage involves accumulation, growth and development of cell layers on the surface. During the third maturation phase, the insoluble three-dimensional matrix or extracellular polymeric substance (EPS) is formed. This “film” encases the microbes and serves as a channel for bulk fluids to flow and permits the distribution of chemical signals and nutrients (20). Lastly, as the colonies grow, quorum sensing occurs triggering expression of cleavage enzymes that subsequently release bacteria from the colonies resulting in the last step, detachment and dispersion (21). These bacteria are now free to seed other locations within the host and begin the cycle anew (22,23).
The challenges of biofilm
Biofilm can be particularly problematic to prosthetic surgeons and patients as they are not only resistant to most traditional treatments but are also difficult to prevent. Most antibiotic strategies, such as identifying the minimum inhibitory concentration of an antibiotic to determine the appropriate amount of drug for a specific strain of bacteria, are designed for planktonic bacteria and may be insufficient for adherent bacteria, sometimes by several orders of magnitude (24). While current antibiotics were designed to eradicate planktonic bacteria, biofilm colonies are not so easily studied as they are difficult to reproduce by standard methods (16,22). These infections also tend to resist innate and adaptive immune responses as well as antimicrobial agents due to their thick extracellular matrix that serves as a barrier to impede the penetration of antibiotics (25). Moreover, antibiotics that do reach the microbe biomass layer may be rendered less effective due to their reduced metabolism and sub-therapeutic concentrations. Finally, biofilms are slow growing to the point of dormancy, and these “persister cells” have the ability to remain viable even after treatment with very high doses of antibiotics (26).
With regards to PP implants, the formation of a fibrous capsule represents another hurdle for antibiotic treatment and penetration of infected implants. These capsules are typically avascular, which further results in reduced antibiotic delivery to the intended area of treatment (27). With reduced drug delivery, there is decreased distribution of chemotactic signals, e.g., cytokines that are necessary to induce an inflammatory response or stimulate neutrophils to reach microbes deep within the biofilm colonies (15).
Biofilms may possibly play a role in the spread of antimicrobial resistance through the horizontal transfer of resistance and virulence genes when in close proximity within the extracellular biofilm matrix (28). Exposure to subtherapeutic concentrations of antibiotics allows for selection pressures and the potential for development of resistant or virulent strains of bacteria. Together, the challenges in treating biofilms associated with prosthetic-associated infections emphasize the importance of prosthesis removal in most cases.
Biofilms in penile prostheses
Biofilm development is widely accepted as a pathologic step in PP infections, and their tolerance or resistance to traditional antimicrobial regimens heightens their clinical importance. Prosthetic surgeons have attempted to use standard culture results to tailor antibiotic therapy for revision patients. Nonetheless, multi-institutional data evaluating clinically infected PP explants have documented non-specific or even no growth cultures in up to 33% of cases (29). This may be secondary to flaws in culture collection techniques, the administration of antibiotics prior to culture collection, or the challenges in growing and identifying all biofilm-associated microbes (8).
With the advent of infection-retardant coatings and revision washout protocols, current literature documents a decrease in infection rates from 2–4% to less than 2% in primary implants, and from 7–18% to 2–3% in revision cases (10,30-35). Moreover, recent systematic reviews report a change in the microbial composition in clinically uninfected and infected PP over time (29). The abundance of coagulase-negative staphylococcal species, most commonly S. epidermidis, have shown a decreased proportion in cultures for explanted PP. Other species such as E. coli, P. aeruginosa, Enterococcus species, and even fungi that form biofilms have been on the rise (29,36).
Table 1 summarizes the review of the presence of biofilm identified on PP revision surgery for both clinically infected and non-infected implants. While there were only three studies that explicitly listed the number of biofilms identified in their cohort, we also included several others that mentioned swabbing and culturing of any bacterial biofilm during revision procedures. In 1986, Nickel et al. described one of the first instances of biofilm presence in two patients with clinically infected PP, both of which harbored rod-shaped and coccoid bacterial cells (12). Subsequently in 2006, Silverstein et al. described the presence of bacteria on laser microscopy among eight of ten non-antibiotic coated-PP explanted for mechanical function, seven of which had biofilm (13). Ciftci et al. also specifically identified biofilm in two of 18 non-infected implants who had a positive culture; both of these biofilms grew S. epidermidis (14). Most recently, Chung et al. also identified biofilm on both standard culture and next-generation sequencing (NGS) in 24 and 48 of 83 samples, respectively (36). Interestingly, this pilot study was the first to assess the utility of NGS in the detection of biofilm and was not only able to detect microbes better than traditional culture, but also detected different microbial profiles for PP explanted for different surgical indications. This may help guide the selection of peri-operative antibiotics and PP-coated antibiotics or hydrophilic dips for individualized scenarios.
Based on historic trends, in revision cases performed for clinically uninfected cases, most cultures from explanted PP are positive for S. epidermidis, a part of common skin flora. Even in the setting of infected PP, the reported presentations are typically with lower toxicity and are confined to the implant space. These bacteria are likely introduced during primary implantation and once they form their mucinous biofilm, they appear able to live in the PP environment without always causing clinical signs of infection (10,27). During revision or salvage procedures, the disruption of pre-existing biofilms and dissemination of these bacteria are thought to contribute to the higher infection rates (37).
In recent articles assessing antibiotic-coated implants, the prevalence of Staphylococcal cultures seems to have decreased, with a slow rise in the incidence of more toxic organisms such as E. coli, P. aeruginosa, Enterococcus species, and even fungal species, such as C. albicans. The recent multi-centered study by Gross et al. assessing cultures in 227 infected implants undergoing revisions found that E. coli was the most common isolate (18%), coagulase-negative staphylococcus was the second (15%), and Candida species isolates were the third most common (11%) (29,38). Even more recently, a study using NGS found that P. aeruginosa and E. coli were the most frequent and abundant organisms encountered in their cohort of infected and mechanically malfunctioned patients, respectively (36). Some reports have also demonstrated a discrepancy in the culture data between the first revision surgery for non-infectious reasons when compared to the second revision for infectious etiologies (14). Of 202 revision surgeries for infection reported by Chandrapal et al., they found that only 22% of implants grew the same organisms at explantation for infection when compared to their original swabs at first revision (39).
Current applications to reduce biofilm attachment
Advances in PP designs with the use of infection-retardant coatings have also led to significant decreases in overall PP infection rates (40). Boston Scientific (Marlborough, MA) introduced the InhibiZone technology into the AMS 700 inflatable PP in 2001, which contains minocycline and rifampin (41). Devices were impregnated with this combination due to the low incidence of allergies and the efficacy of these antibiotics against Gram positive and negative bacteria commonly seen in PP infections (41). The Coloplast (Minneapolis, MN) Titan inflatable PP comes with a hydrophilic coating in 2002, known as polyvinylpyrrolidone, which absorbs an antibiotic when dipped into an aqueous solution, giving prosthetic surgeons more flexibility when tailoring their antibiotic of choice (42,43). While the ideal antibiotic solution and “dipping time” have yet to be described, a combination of vancomycin and gentamicin mixed in normal saline solution is typically the antibiotics of choice unless clinically contraindicated due to its broad coverage against most Gram positive and negative microbes. Due to its convenience and efficacy, some authors have also studied the utility of Irrisept (Irrimax Corporation, Lawrenceville, GA), a low-concentration 0.05% chlorhexidine gluconate solution that has broad spectrum antibacterial, antifungal, and antiviral properties as a dipping solution (44). They found satisfactory coating results on Coloplast Titan PP when compared to saline soaked controls. Furthermore, they found no difference in coating adherence between soaking times of 1, 15, 30 and 60 minutes. The introduction of antibiotic-coated PP has also been shown to improve infection-free survival in diabetic cohorts (45). A systematic review and meta-analysis of 14 clinical case studies found the rates of infectious complications to be significantly lower in the cohort with antibiotic coated prostheses at 0.89% when compared to those without (2.32%; P<0.01) (46). A recent study performed by Jani et al. also found higher rates of culture positive isolates in uncoated PP regardless of whether explant surgery was performed for infectious or non-infectious etiologies (47). Overall, the utility of antibiotic coatings confers significant advantages in preventing postoperative device infections (48,49).
The use of antibiotic washout during revision surgery is also a critical step that has been shown to significantly reduce infection rates. Mulcahy et al. first revolutionized the management of infected PP by assessing the feasibility of immediate replacement of inflatable PP at the time of revision surgery after a seven-step antibiotic irrigation protocol (50). Prior to the introduction of the salvage technique, treatment of PP infection involved the removal of all prosthetic components along with copious antibiotic irrigation to the PP site (51). This often resulted in fibrosis and scarring of the corpora cavernosa, complicating subsequent reimplantation in the future. Since the development of the Mulcahy protocol in 1996, other groups have demonstrated promising results with the use of the immediate salvage technique and also modified their techniques with a delayed or malleable salvage method with other antibiotic irrigation solutions (52-56). For example, cohorts from Wilson and Henry both reported a reduction of infection rate from 10% to 3% in the cohort who underwent antiseptic washout after revision surgery (27,33). Importantly, while antibiotic coatings on PP have demonstrated desirable outcomes in primary surgeries, its effects on revision cases are less pronounced, and studies have noted decreased rates of infection in revision surgeries only if adjunctive revision washout was performed (33,57). This indicates that while antibiotic coatings can prevent infections secondary to planktonic bacteria during initial implantations, once biofilms are established, a more rigorous irrigation and lavage is necessary to eliminate latent microbes and disrupt biofilms in previous implant spaces (10). Hence, revision washout is recommended even in patients who undergo revision for non-infectious indications (27). A report by Abouassaly et al. also commented that revision washouts should be aggressive, with the use of copious amounts of one type of antimicrobial solution rather than smaller amounts of several antibiotics (58). Occasionally, mechanical debridement of biofilm in the implant space may also be necessary.
Patients with prior PP who are undergoing revision surgeries are also considered high risk for infection, likely for reasons related to biofilm formation as mentioned previously. Traditionally, revision surgeries have a 10–13% rate of infection, a significantly higher percentage when compared to primary cases at <3% (32,33). Another study demonstrated that risk of device infection strongly correlated with an increased number of implantations with 6.8% risk for the primary implantation compared to 100% by the fifth implantation (59). Finally, the consequence of device infection is multifactorial; hence, careful patient selection, paired with the advent of recent technological innovations is essential to ameliorate the risk of this complication.
Future of biofilm prevention and treatment
While there are many novel possibilities for the prevention and treatment of bacterial biofilms, the majority of these methods are still in the experimental phase and are still being studied in vitro. Given the surgical volume in orthopedics and neurosurgery, most innovative biomaterial strategies are focused on these implants, but the advancements can be translated to the urologic prosthetic realm (9). The primary strategy to prevent biofilm formation and subsequent device infections is to prevent bacterial attachment altogether. Surface modification or impregnated antibiotic are methods that can render biologic surfaces inhospitable to microbes. Both the Boston Scientific and Coloplast inflatable PP are coated implants, but only the Coloplast malleable PP is coated with a hydrophilic layer to create a physical barrier to prevent microbial attachment (23). A new inflatable and malleable PP from Rigicon (Ronkonkoma, NY) also supports a hydrophilic layer (60). Changes in hydrophobicity as a result of altering the electrical charges of a surface can also prevent certain proteins from binding to solid surfaces. For example, the application of heparin coating has been used on intraocular lenses and urethral catheters to reduce bacterial adhesion (61-63). The addition of morphologic barriers such as antimicrobial peptides have also been utilized in the orthopedic field (64-68). However, these peptides have shorter duration of action which may pose a limitation for its use in PP. Other antimicrobials that have been tested for prosthetic coating include chlorhexidine, nitric oxide and triclosan, but have not been used in PP (69). Also, biologic approaches such as the use of commensal bacteria to prevent the colonization of pathogenic bacteria may play a protective role in the adhesion and proliferation of pathologic bacteria. The use of biosurfactant produced by these probacteria to inhibit attachment of other virulent strains of bacteria in clinical practice is still unclear (70,71).
Aside from targeting adhesion, the first phase of biofilm formation, studies have attempted to inhibit microcolony formation by disrupting the EPS. The formation of EPS allows for cell-to-cell communication between microbes that aid in the development of resistance through clonal gene expression changes and can also act as a diffusion barrier for antibiotics (23). One method to destabilize the EPS include enzymatic disruption of fibrin deposits that act as the central structural unit of biofilms with the use of tissue plasminogen activator. Mechanical disruption using microbubble-based, contrast enhanced, ultrasound imaging creates cavitations that disrupt the biologic fluid and tissue membrane interfaces of biofilm (72). It can delineate anatomy intraoperatively, allow for targeted drug delivery and facilitate gene therapy through alterations in cell membrane permeability, as seen in hepatobiliary anatomy (73-77). In 2015, an in vivo study by Li et al. evaluated the effects of ultrasound-targeted microbubble destruction in combination with a cationic antimicrobial peptide, human β-defensin 3 on antibiotic-resistant Staphylococcus biofilms (78). Their findings suggest that the combination of ultrasound use significantly decreased the biofilm densities, percentage of live cells, and viable counts of tested Staphylococcus colony forming units. The degree of mechanical insult induced by acoustic rupture of these microbubbles depends largely on biofilm age and thickness. Future work is necessary to determine if this modality will be a safe and efficacious method in preventing or treating PP biofilms.
Experimental studies evaluating dispersion-inducing agents that coax microbes to shed their protective biofilm coating may be an important proof-of-concept that can aid in biofilm control. Studies in S. aureus species have shown that active quorum-sensing prevents the formation of biofilm (79). The disruption of the accessory gene regulator (agr) gene function, which mediates the quorum-sensing mechanism, may theoretically represent a method to induce biofilm dispersion (79,80). In Pseudomonas species, alterations in genetic regulation of intracellular signal transducers e.g., Lipopolysaccharide assembly protein A (LapA) proteins, and activation of EPS enzymes e.g., LapG proteinase, represents mechanisms to promote dispersion of established biofilms as well (81,82).
Recently, emerging technologies have allowed for more sensitive and superior testing, one of which is the advancement of rapid molecular sequencing. Our group recently performed a study assessing the utility of a novel technology, NGS, for the identification of microorganism profiles on explanted biofilms (36). We found that NGS was able to detect microbes more abundantly and frequently when compared to standard culture and that the microbial profiles differed based on etiologies for revision surgery, including infection, erosion, or mechanical malfunction. We also found that NGS tended to detect a polymicrobial profile, while culture results were only monomicrobial. Although the significance of the polymicrobial findings detected by NGS have yet to be ascertained, these findings may guide surgeons in the selection of perioperative antibiotics and hydrophilic antibiotic dips in individualized clinical scenarios for the treatment of biofilms (36,83).
Limitations
There are some limitations to our scoping review process. While it entails a different screening criteria or process than a systematic review, it is a broader and less refined search. It also requires multiple search strategies and increases the emphasis for hand searching within individual articles. Hence, it requires a larger team for screening larger volumes of literature which may lead to inconsistencies in interpreting and conducting these reviews. There is also a possibility that we may have missed some relevant studies due to database selection or inclusion of only articles published in English. Depth of analysis may be limited by time constraints as well to review all articles. Lastly, the lack of critical appraisal of included studies is also a limitation. It cannot be used to endorse guideline recommendations as it did not assess the quality of included studies and is also limited to identifying gaps in the literature related to low quality research.
Conclusions
PP remains the gold standard for treatment of ED given its reliability and efficacy. Infection remains the most feared complication of prosthetic surgery, which usually results in device removal. While biofilms are believed to be the culprit, the degree to which this bacterial matrix is truly pathogenic remains unknown—especially given its high prevalence even in asymptomatic patients. What has been noticed is that in the era of antibiotic-coated implants, less common, but more virulent organisms are beginning to replace the more common Staphylococcal species in clinically infected implants. These patients also present with more toxic, systemic infections and ultimately require device removal altogether for source control. While revision washout protocols and antibiotic-coated implants have decreased overall infection rates, testing of preliminary and experimental biofilm-control strategies is necessary to further address this clinical issue. Moreover, additional studies including a prospective, randomized controlled trial is currently underway to define the significance of bacterial presence in biofilms using innovative technology such as NGS.
Acknowledgments
Funding: None.
Footnote
Reporting Checklist: The authors have completed the PRISMA-ScR reporting checklist. Available at https://tau.amegroups.com/article/view/10.21037/tau-22-195/rc
Peer Review File: Available at https://tau.amegroups.com/article/view/10.21037/tau-22-195/prf
Conflicts of Interest: All authors have completed the ICMJE uniform disclosure form (available at https://tau.amegroups.com/article/view/10.21037/tau-22-195/coif). PHC serves as an unpaid editorial board member of Translational Andrology and Urology from December 2021 to November 2023. GDH consults for and receives research support from Boston Scientific, MicroGenDx, Coloplast, Signati Medical. PHC consults for and receives research support from Boston Scientific and Coloplast. The other authors have no conflicts of interest to declare.
Ethical Statement: The authors are accountable for all aspects of the work in ensuring that questions related to the accuracy or integrity of any part of the work are appropriately investigated and resolved.
Open Access Statement: This is an Open Access article distributed in accordance with the Creative Commons Attribution-NonCommercial-NoDerivs 4.0 International License (CC BY-NC-ND 4.0), which permits the non-commercial replication and distribution of the article with the strict proviso that no changes or edits are made and the original work is properly cited (including links to both the formal publication through the relevant DOI and the license). See: https://creativecommons.org/licenses/by-nc-nd/4.0/.
References
- Wilson SK, Delk JR, Salem EA, et al. Long-term survival of inflatable penile prostheses: single surgical group experience with 2,384 first-time implants spanning two decades. J Sex Med 2007;4:1074-9. [Crossref] [PubMed]
- Trost LW, McCaslin R, Linder B, et al. Long-term outcomes of penile prostheses for the treatment of erectile dysfunction. Expert Rev Med Devices 2013;10:353-66. [Crossref] [PubMed]
- Burnett AL, Nehra A, Breau RH, et al. Erectile Dysfunction: AUA Guideline. J Urol 2018;200:633-41. [Crossref] [PubMed]
- Rezaee ME, Ward CE, Brandes ER, et al. A Review of Economic Evaluations of Erectile Dysfunction Therapies. Sex Med Rev 2020;8:497-503. [Crossref] [PubMed]
- Moore J, Whelan TF, Langille GM. The use of penile prostheses in the management of priapism. Transl Androl Urol 2017;6:S797-803. [Crossref] [PubMed]
- Ralph DJ, Garaffa G, Muneer A, et al. The immediate insertion of a penile prosthesis for acute ischaemic priapism. Eur Urol 2009;56:1033-8. [Crossref] [PubMed]
- Scherzer ND, Dick B, Gabrielson AT, et al. Penile Prosthesis Complications: Planning, Prevention, and Decision Making. Sex Med Rev 2019;7:349-59. [Crossref] [PubMed]
- Wilson SK, Gross MS. Biofilm and penile prosthesis infections in the era of coated implants: 2021 update. Int J Impot Res 2022;34:411-5. [Crossref] [PubMed]
- Isguven S, Chung PH, Machado P, et al. Minimizing Penile Prosthesis Implant Infection: What Can We Learn From Orthopedic Surgery? Urology 2020;146:6-14. [Crossref] [PubMed]
- Dawn LE, Henry GD, Tan GK, et al. Biofilm and Infectious Agents Present at the Time of Penile Prosthesis Revision Surgery: Times Are a Changing. Sex Med Rev 2017;5:236-43. [Crossref] [PubMed]
- Tricco AC, Lillie E, Zarin W, et al. PRISMA Extension for Scoping Reviews (PRISMA-ScR): Checklist and Explanation. Ann Intern Med 2018;169:467-73. [Crossref] [PubMed]
- Nickel JC, Heaton J, Morales A, et al. Bacterial biofilm in persistent penile prosthesis-associated infection. J Urol 1986;135:586-8. [Crossref] [PubMed]
- Silverstein AD, Henry GD, Evans B, et al. Biofilm formation on clinically noninfected penile prostheses. J Urol 2006;176:1008-11. [Crossref] [PubMed]
- Ciftci S, Nemut T, Culha MM, et al. Non-infected penile prosthesis cultures during revision surgery; comparison between antibiotic coated and non - coated devices. Int Braz J Urol 2016;42:1183-9. [Crossref] [PubMed]
- Arciola CR, Campoccia D, Speziale P, et al. Biofilm formation in Staphylococcus implant infections. A review of molecular mechanisms and implications for biofilm-resistant materials. Biomaterials 2012;33:5967-82. [Crossref] [PubMed]
- Bjarnsholt T, Ciofu O, Molin S, et al. Applying insights from biofilm biology to drug development - can a new approach be developed? Nat Rev Drug Discov 2013;12:791-808. [Crossref] [PubMed]
- Donlan RM, Costerton JW. Biofilms: survival mechanisms of clinically relevant microorganisms. Clin Microbiol Rev 2002;15:167-93. [Crossref] [PubMed]
- Silverstein A, Donatucci CF. Bacterial biofilms and implantable prosthetic devices. Int J Impot Res 2003;15:S150-4. [Crossref] [PubMed]
- Mack D, Becker P, Chatterjee I, et al. Mechanisms of biofilm formation in Staphylococcus epidermidis and Staphylococcus aureus: functional molecules, regulatory circuits, and adaptive responses. Int J Med Microbiol 2004;294:203-12. [Crossref] [PubMed]
- Bjarnsholt T, Alhede M, Alhede M, et al. The in vivo biofilm. Trends Microbiol 2013;21:466-74. [Crossref] [PubMed]
- Yarwood JM, Bartels DJ, Volper EM, et al. Quorum sensing in Staphylococcus aureus biofilms. J Bacteriol 2004;186:1838-50. [Crossref] [PubMed]
- Faller M, Kohler T. The Status of Biofilms in Penile Implants. Microorganisms 2017;5:19. [Crossref] [PubMed]
- Herati AS, Lo EM. Penile prosthesis biofilm formation and emerging therapies against them. Transl Androl Urol 2018;7:960-7. [Crossref] [PubMed]
- Isguven S, Fitzgerald K, Delaney LJ, et al. In vitro investigations of Staphylococcus aureus biofilms in physiological fluids suggest that current antibiotic delivery systems may be limited. Eur Cell Mater 2022;43:6-21. [Crossref] [PubMed]
- Davies D. Understanding biofilm resistance to antibacterial agents. Nat Rev Drug Discov 2003;2:114-22. [Crossref] [PubMed]
- Lee JH, Kim YG, Gwon G, et al. Halogenated indoles eradicate bacterial persister cells and biofilms. AMB Express 2016;6:123. [Crossref] [PubMed]
- Henry GD, Wilson SK, Delk JR 2nd, et al. Revision washout decreases penile prosthesis infection in revision surgery: a multicenter study. J Urol 2005;173:89-92. [Crossref] [PubMed]
- Costerton JW, Montanaro L, Arciola CR. Biofilm in implant infections: its production and regulation. Int J Artif Organs 2005;28:1062-8. [Crossref] [PubMed]
- Gross MS, Phillips EA, Carrasquillo RJ, et al. Multicenter Investigation of the Micro-Organisms Involved in Penile Prosthesis Infection: An Analysis of the Efficacy of the AUA and EAU Guidelines for Penile Prosthesis Prophylaxis. J Sex Med 2017;14:455-63. [Crossref] [PubMed]
- Carrasquillo RJ, Munarriz RM, Gross MS. Infection Prevention Considerations for Complex Penile Prosthesis Recipients. Curr Urol Rep 2019;20:12. [Crossref] [PubMed]
- Nosé BD, Grimberg DCD, Lentz AC. Update on Intraoperative Cultures, Biofilms, and Modifiable Factors During Revision of Clinically Non-Infected Penile Implants. Sex Med Rev 2021;9:160-8. [Crossref] [PubMed]
- Wilson SK, Costerton JW. Biofilm and penile prosthesis infections in the era of coated implants: a review. J Sex Med 2012;9:44-53. [Crossref] [PubMed]
- Wilson SK, Zumbe J, Henry GD, et al. Infection reduction using antibiotic-coated inflatable penile prosthesis. Urology 2007;70:337-40. [Crossref] [PubMed]
- Kava BR, Kanagarajah P, Ayyathurai R. Contemporary revision penile prosthesis surgery is not associated with a high risk of implant colonization or infection: a single-surgeon series. J Sex Med 2011;8:1540-6. [Crossref] [PubMed]
- Gross MS, Vollstedt AJ, Cleves MA, et al. Multicenter investigation on the influence of climate in penile prosthesis infection. Int J Impot Res 2020;32:387-92. [Crossref] [PubMed]
- Chung PH, Leong JY, Phillips CD, et al. Microorganism Profiles of Penile Prosthesis Removed for Infection, Erosion, and Mechanical Malfunction Based on Next-Generation Sequencing. J Sex Med 2022;19:356-63. [Crossref] [PubMed]
- Henry GD, Carson CC, Wilson SK, et al. Revision washout decreases implant capsule tissue culture positivity: a multicenter study. J Urol 2008;179:186-90; discussion 190. [Crossref] [PubMed]
- Gross MS, Reinstatler L, Henry GD, et al. Multicenter Investigation of Fungal Infections of Inflatable Penile Prostheses. J Sex Med 2019;16:1100-5. [Crossref] [PubMed]
- Chandrapal J, Harper S, Gerber-Davis L, et al. 402 Comparison of Intraoperative Cultures in Patients Undergoing Removal/Replacement of a Non-infected Penile Prosthesis Followed by Subsequent Salvage or Explantation. The Journal of Sexual Medicine 2020;17:S116. [Crossref]
- Mulcahy JJ, Köhler TS, Wen L, et al. Penile implant infection prevention part II: device coatings have changed the game. Int J Impot Res 2020;33:801-7. [Crossref] [PubMed]
- Mulcahy JJ. Penile prosthesis infection: progress in prevention and treatment. Curr Urol Rep 2010;11:400-4. [Crossref] [PubMed]
- Dhabuwala C. In vitro assessment of antimicrobial properties of rifampin-coated Titan(®) Coloplast penile implants and comparison with Inhibizone J Sex Med 2010;7:3516-9. [Crossref] [PubMed]
- Dhabuwala C, Sheth S, Zamzow B. Infection rates of rifampin/gentamicin-coated Titan Coloplast penile implants. Comparison with Inhibizone-impregnated AMS penile implants. J Sex Med 2011;8:315-20. [Crossref] [PubMed]
- Irrisept - Antimicrobial Wound Lavage: Irrimax Corporation; 2022. Available online: https://www.irrisept.com/about-us/.
- Lipsky MJ, Onyeji I, Golan R, et al. Diabetes Is a Risk Factor for Inflatable Penile Prosthesis Infection: Analysis of a Large Statewide Database. Sex Med 2019;7:35-40. [Crossref] [PubMed]
- Mandava SH, Serefoglu EC, Freier MT, et al. Infection retardant coated inflatable penile prostheses decrease the incidence of infection: a systematic review and meta-analysis. J Urol 2012;188:1855-60. [Crossref] [PubMed]
- Jani K, Smith C, Delk JR 2nd, et al. Infection Retardant Coatings Impact on Bacterial Presence in Penile Prosthesis Surgery: A Multicenter Study. Urology 2018;119:104-8. [Crossref] [PubMed]
- Mahon J, Dornbier R, Wegrzyn G, et al. Infectious Adverse Events Following the Placement of a Penile Prosthesis: A Systematic Review. Sex Med Rev 2020;8:348-54. [Crossref] [PubMed]
- Carrasquillo RJ, Gross MS. Infection Prevention Strategies Prior to Penile Implant Surgery. Eur Urol Focus 2018;4:317-20. [Crossref] [PubMed]
- Brant MD, Ludlow JK, Mulcahy JJ. The prosthesis salvage operation: immediate replacement of the infected penile prosthesis. J Urol 1996;155:155-7. [Crossref] [PubMed]
- Lao M, Graydon RJ, Bieniek JM. Salvage penile prosthetic surgery utilizing temporary malleable implants. Transl Androl Urol 2017;6:S806-12. [Crossref] [PubMed]
- Knoll LD. Penile prosthetic infection: management by delayed and immediate salvage techniques. Urology 1998;52:287-90. [Crossref] [PubMed]
- Mulcahy JJ. Long-term experience with salvage of infected penile implants. J Urol 2000;163:481-2. [Crossref] [PubMed]
- Kaufman JM, Kaufman JL, Borges FD. Immediate salvage procedure for infected penile prosthesis. J Urol 1998;159:816-8. [Crossref] [PubMed]
- Köhler TS, Modder JK, Dupree JM, et al. Malleable implant substitution for the management of penile prosthesis pump erosion: a pilot study. J Sex Med 2009;6:1474-8. [Crossref] [PubMed]
- Gross MS, Phillips EA, Balen A, et al. The Malleable Implant Salvage Technique: Infection Outcomes after Mulcahy Salvage Procedure and Replacement of Infected Inflatable Penile Prosthesis with Malleable Prosthesis. J Urol 2016;195:694-7. [Crossref] [PubMed]
- Carson CC 3rd. Efficacy of antibiotic impregnation of inflatable penile prostheses in decreasing infection in original implants. J Urol 2004;171:1611-4. [Crossref] [PubMed]
- Abouassaly R, Angermeier KW, Montague DK. Risk of infection with an antibiotic coated penile prosthesis at device replacement for mechanical failure. J Urol 2006;176:2471-3. [Crossref] [PubMed]
- Montgomery BD, Lomas DJ, Ziegelmann MJ, et al. Infection risk of undergoing multiple penile prostheses: an analysis of referred patient surgical histories. Int J Impot Res 2018;30:147-52. [Crossref] [PubMed]
- Rigicon - Innovative Urological Solutions: Rigicon, INC.; 2022 [Available from: https://www.rigicon.com/.
- Ruggieri MR, Hanno PM, Levin RM. Reduction of bacterial adherence to catheter surface with heparin. J Urol 1987;138:423-6. [Crossref] [PubMed]
- Arciola CR, Caramazza R, Pizzoferrato A. In vitro adhesion of Staphylococcus epidermidis on heparin-surface-modified intraocular lenses. J Cataract Refract Surg 1994;20:158-61. [Crossref] [PubMed]
- Nagaoka S, Kawakami H. Inhibition of bacterial adhesion and biofilm formation by a heparinized hydrophilic polymer. ASAIO J 1995;41:M365-8. [Crossref] [PubMed]
- Kazemzadeh-Narbat M, Kindrachuk J, Duan K, et al. Antimicrobial peptides on calcium phosphate-coated titanium for the prevention of implant-associated infections. Biomaterials 2010;31:9519-26. [Crossref] [PubMed]
- Antoci V Jr, Adams CS, Hickok NJ, et al. Vancomycin bound to Ti rods reduces periprosthetic infection: preliminary study. Clin Orthop Relat Res 2007;88-95. [Crossref] [PubMed]
- Antoci V Jr, King SB, Jose B, et al. Vancomycin covalently bonded to titanium alloy prevents bacterial colonization. J Orthop Res 2007;25:858-66. [Crossref] [PubMed]
- Parvizi J, Wickstrom E, Zeiger AR, et al. Frank Stinchfield Award. Titanium surface with biologic activity against infection. Clin Orthop Relat Res 2004;33-8. [Crossref] [PubMed]
- Stewart S, Barr S, Engiles J, et al. Vancomycin-modified implant surface inhibits biofilm formation and supports bone-healing in an infected osteotomy model in sheep: a proof-of-concept study. J Bone Joint Surg Am 2012;94:1406-15. [Crossref] [PubMed]
- Campoccia D, Montanaro L, Arciola CR. A review of the biomaterials technologies for infection-resistant surfaces. Biomaterials 2013;34:8533-54. [Crossref] [PubMed]
- Luna JM, Rufino RD, Sarubbo LA, et al. Evaluation antimicrobial and antiadhesive properties of the biosurfactant Lunasan produced by Candida sphaerica UCP 0995. Curr Microbiol 2011;62:1527-34. [Crossref] [PubMed]
- Zeraik AE, Nitschke M. Biosurfactants as agents to reduce adhesion of pathogenic bacteria to polystyrene surfaces: effect of temperature and hydrophobicity. Curr Microbiol 2010;61:554-9. [Crossref] [PubMed]
- Lattwein KR, Shekhar H, Kouijzer JJP, et al. Sonobactericide: An Emerging Treatment Strategy for Bacterial Infections. Ultrasound Med Biol 2020;46:193-215. [Crossref] [PubMed]
- Huang C, Zhang H, Bai R. Advances in ultrasound-targeted microbubble-mediated gene therapy for liver fibrosis. Acta Pharm Sin B 2017;7:447-52. [Crossref] [PubMed]
- Lee S, Al-Kaabi L, Mawart A, et al. Ultrasound-mediated drug delivery by gas bubbles generated from a chemical reaction. J Drug Target 2018;26:172-81. [Crossref] [PubMed]
- Chandra A, Gupta V, Rahul R, et al. Intraoperative ultrasonography of the biliary tract using saline as a contrast agent: a fast and accurate technique to identify complex biliary anatomy. Can J Surg 2017;60:316-22. [Crossref] [PubMed]
- Nie F, Xu HX, Lu MD, et al. Anti-angiogenic gene therapy for hepatocellular carcinoma mediated by microbubble-enhanced ultrasound exposure: an in vivo experimental study. J Drug Target 2008;16:389-95. [Crossref] [PubMed]
- Delalande A, Kotopoulis S, Postema M, et al. Sonoporation: mechanistic insights and ongoing challenges for gene transfer. Gene 2013;525:191-9. [Crossref] [PubMed]
- Li S, Zhu C, Fang S, et al. Ultrasound microbubbles enhance human β-defensin 3 against biofilms. J Surg Res 2015;199:458-69. [Crossref] [PubMed]
- Boles BR, Horswill AR. Agr-mediated dispersal of Staphylococcus aureus biofilms. PLoS Pathog 2008;4:e1000052. [Crossref] [PubMed]
- Traber KE, Lee E, Benson S, et al. agr function in clinical Staphylococcus aureus isolates. Microbiology (Reading) 2008;154:2265-74. [Crossref] [PubMed]
- Gjermansen M, Nilsson M, Yang L, et al. Characterization of starvation-induced dispersion in Pseudomonas putida biofilms: genetic elements and molecular mechanisms. Mol Microbiol 2010;75:815-26. [Crossref] [PubMed]
- Gjermansen M, Ragas P, Sternberg C, et al. Characterization of starvation-induced dispersion in Pseudomonas putida biofilms. Environ Microbiol 2005;7:894-906. [Crossref] [PubMed]
- Chung PH, Leong JY, Teplitsky S, et al. Next-generation DNA sequencing for infected genitourinary implants: How I do it. Can J Urol 2020;27:10418-23. [PubMed]